Category Archives: Biotechnology
Alternative meat: eating microbial protein and others
18th December 2022
Translated from the original article in Catalan (10th December 2022)
PROBLEMS OF ANIMAL PROTEIN PRODUCTION
Most of the protein that we humans consume is meat protein of animal origin produced by industrial livestock farming, be it birds or mammals, and of these mainly ruminants such as beef, whose global production has doubled in the last 50 years. Industrial farm systems, both intensive and extensive, have very negative environmental consequences. As we see schematically in Figure 1, the main disadvantages are:
The area of land occupied by cattle breeding is increasing. Counting the pastureland and vegetable crops to feed the animals —cereals and soybeans in particular—, almost 30% of the land surface is reached, much more than that devoted to crops for human consumption, 13%. In the case of soy, for example, 3/4 of its production is dedicated to feed, and an important part comes from regions of Brazil that were previously jungle or savannah (Hooper 2022).
The fresh water used for the manufacture of any product is called the water footprint (Hidrofilic 2017). In the case of livestock, which includes the cultivation of vegetable feed, water footprint is much greater than any other agricultural crop: 15000 L of water are needed for each kg of beef, 9000 L per kg of sheep meat, 6000 L per kg of pork, while only 300 L is needed for kg of vegetables or 900 L per kg of fruits or about 4000 L per kg of legumes, even less than for animals (Mekonnen & Hoekstra 2010). As we can see (Figure 1), it is estimated that in the USA 30% of the total water footprint is due to industrial livestock.
A third of global greenhouse gas (GHG) emissions are caused by the food system, and within this livestock farming is the main contributor, especially due to the methane gas expelled by ruminants, a result of the metabolism of methanogenic archaea, the final step of rumen fermentation. As a GHG, methane emissions from cattle cause the most environmental impact by far, being about 100 kg of methane for every 100 g of protein produced (Poore & Nemecek 2018).
Finally, it is necessary to take into account the repercussions of land degradation and erosion caused by industrial livestock farming, as well as soil acidification, contamination by antibiotics and eutrophication due to the excessive use of fertilizers (Humpenöder et al. 2022).
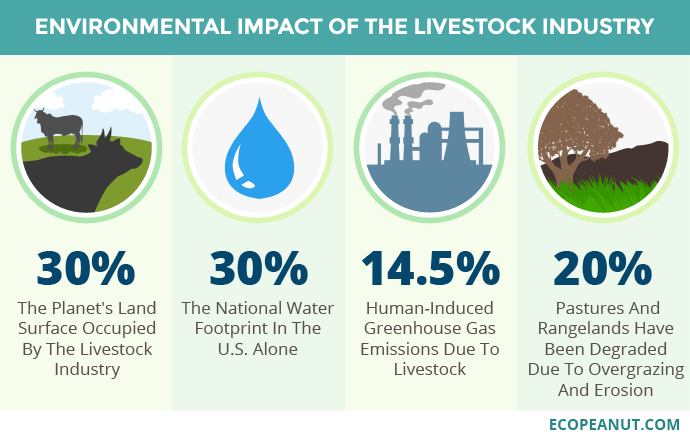
Figure 1. Environmental impact of the livestock industry. Image taken from Ecopeanut.com.
It is estimated that by 2050 the world’s human population will be almost 10,000 million (now in 2022 we are 7,800 million), for which we will need about 400 million tons of meat and 800 of dairy products per year, an amount that cannot be achieved by the low efficiency of vegetable protein in feed to animal protein, which is 6 kg of vegetable for 1 kg of animal (Ritala 2017).
All in all, some very concerned environmental activists like George Monbiot believe that agricultural and livestock exploitations lead to destruction, exploitation and economic senselessness that are killing the planet. However, he also believes that there is hope for a more sustainable and healthier world that would go through a consumption of microbes instead of animals (Hooper 2022).
Therefore, this medium-term situation is not environmentally sustainable, and alternatives must be found to replace much of the animal protein with other sources, such as microbial and others.
———
THE VEGETABLE ALTERNATIVE
The vegetable protein alternative is well known and forms part of our usual diet (Figure 2). Taking it to the extreme, vegetarians base their diet only on vegetables and exclude meat foods. In India they are 1/3 of the population, but in the rest of the world vegetarians are a minority, 5% in Europe. The reasons for vegetarians – and vegans, who are more strict – are very diverse, such as ethical (animal sensitivity), health, religious, political, fashion, aesthetic, economic, but also increasingly there are the reasons of environmental and sustainability awareness.
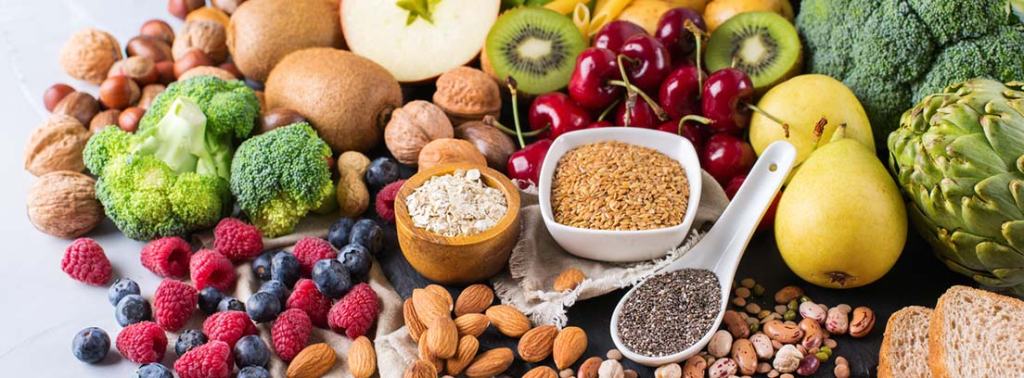
Figure 2. Vegetarian diet. Image taken from Salud Blogs Mapfre.
Vegetable protein sources are nutritionally valuable, since they contain fibre —almost non-existent in meat — and antioxidants, but their protein content is always lower than that of meat, which is 45% of the dry extract The vegetable foods with the most protein are soy (35% of dry extract) and legumes such as peas, chickpeas or beans (20-25%). In contrast, wheat, rice and other cereals or potatoes only contain 10% protein. Milk contains 25% and eggs 40% of the dry extract.
The main disadvantage of vegetable protein compared to animal, in addition to the lower total protein content, is the lower nutritional quality in terms of essential amino acids and lower digestibility, with which it is necessary to increase 10-20% of protein if only plant foods are consumed (Petrusán et al 2016). Another disadvantage is the environmental one, partly like the animal protein, due to the needs of large areas of land and a lot of water (Ritala et al. 2017).
———-
EATING INSECTS ?
Another alternative is the consumption of insect protein, or entomophagy. The protein content of the dry extract of insects is very high, between 35% for termites and 60% for crickets and locusts, and most edible insects have high contents of essential amino acids, and of fibre, minerals and vitamins. Insect “farms” are very suitable for sustainability and the environment, as they require no land, require little water, emit very few GHGs, and are economically advantageous. Therefore, they have very good potential as quality food (Lange & Nakamura 2021). However, consumer acceptance is a major hurdle, especially in Western countries. Instead, they are common in sub-Saharan Africa, Southeast Asia, Australia, and some Ibero-American countries such as Mexico, where “escamoles” are larvae of the Liometopum apiculatum ant very popular since pre-Hispanic times (Figure 3).
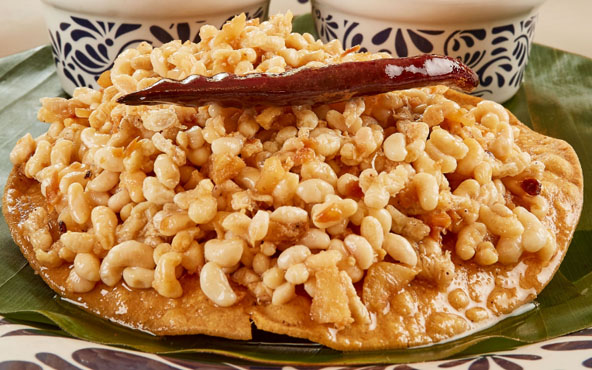
Figure 3. Dish of Mexican escamoles. Image taken from Lideresmexicanos.com.
When insects are produced industrially, it is necessary to consider and control possible sources of food safety risks, such as allergens, pathogenic microorganisms that can be transmitted via insects, or mycotoxins from fungi that contaminate insects (Lange & Nakamura 2021).
Currently, most investments in the production of edible insects are for animal feed. It is being produced as a protein powder for domestic animals, in aquaculture and is starting to be introduced as a supplement to livestock feed. In addition, the droppings of insects in the productive phase can be used as fertilizer (Godwin 2021).
————–
CULTURED OR SYNTHETIC MEAT
Also called “in vitro” meat or “clean” meat or “laboratory meat”, it consists of growing animal muscle tissue in laboratory cultures from stem cells. With this method, which is faster and more efficient than obtaining traditional meat, there is no need to sacrifice animals, nor are there all the mentioned drawbacks of livestock farming.
To obtain cultured meat, a series of requirements summarized in these steps are needed that must work well both from a biological and commercial point of view:
1. Obtain skeletal muscle samples from the appropriate animal
2. Separate the stem cells from the other muscle components and sometimes other cell types
3. Inducing the growth and proliferation of myoblasts in the appropriate physico-chemical conditions and medium, with growth factors
4. Inducing myoblasts to form multinucleated myotubes, in a framework or scaffolding structure, such as collagen
5. Achieve the continued growth of new myoblasts and the differentiation of myotubes into muscle fibres
6. Ensure continued growth throughout scale-up, introducing other components such as adipocytes, with lipids providing palatability
7. Process the resulting product by adding fats, flavourings and other compounds, and shape its physical appearance, all in order to mimic conventional meat products, which is easier with processed products such as minced meat or bacon (Figure 4) (Kadim et al. 2015).
Cultured meat can be just as good and nutritious as conventional meat from farm animals, in addition to the advantages of a drastically lower effect on the environment and on the animal in question. In addition, the yield is clearly greater, since with just one sample of tissue the same amount of meat can be produced as with 80 cows (Bingham 2020).
Although preliminary results so far are very promising, this technology is not yet developed enough for large-scale production, especially in terms of culture media, and consumer acceptance and trust is still very low (Kadim et al. 2015).
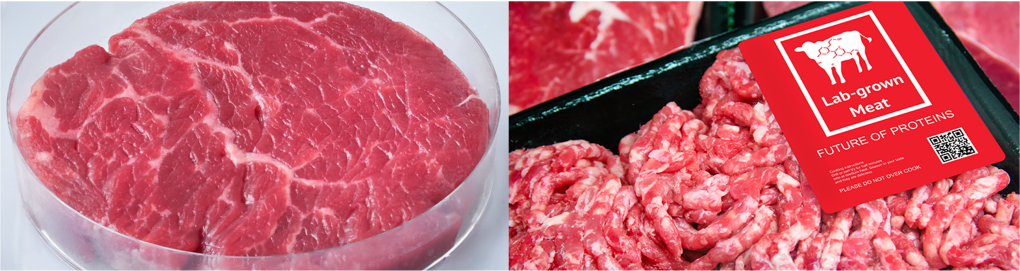
Figure 4. “Culturized” meats, made from animal cell cultures, simulating beef fillet (left) or processed meat (right). Images taken from Bingham (2020).
In a similar way, the production of fish fillets and seafood meat with cell cultures is also being developed, this is what is called cell aquaculture. The progressive replacement of caught fish by “farmed fish” would significantly reduce overfishing, eliminate illegal fishing and destruction of marine ecosystems, and these foods would not have potential pathogens or common contaminants such as methylmercury or particulate microplastics.
These farmed fish and shellfish products also have the organoleptic qualities of wild or farmed marine products but with the advantage of being more sustainable, safer, and healthier. Some of the companies that are developing them use techniques similar to organoid cultures or mini organs, which have been used for about 10 years for the study and treatment of diseases and tumours. Cultures are made in three-dimensional structures allowing the cells to form a natural composition of fat and muscle equivalent to that of the animal (VelSid 2022).
——————-
MICROBIAL PROTEIN
It is also known as Single Cell Protein (SCP) because many were originally single-cell microbes such as most bacteria —including cyanobacteria—, yeasts and some single-celled microalgae, but some filamentous fungi and some multicellular algae are also included. Logically, the microbes that have been studied the most in this regard are the ones that contain the most protein. We have a good review of the types, the process of obtaining and applications in the work of Junaid et al. (2020).
We see in Table 1 a summary of the main products that have been developed, most of them since the 1970s, when, with the first energy and environmental crises, alternatives to the production of meat protein were sought, although most of these products have not had great industrial and/or commercial development.
Table 1. Single Cell Protein products, all with at least 45% protein of dry extract. Adapted from Ritala et al. (2017).
Microorganism | Type | Use | Substrates | Companies (country) | Problems |
Methylophilus,Methylococcus | Methylotroph and methanotroph bacteria, proteobacteria | Animal feed | Methanol, methane | ICI (UK) “Pruteen” 1970s, Calista Inc. (UK) “FeedKind” from biogas | High content of RNA and DNA (>10%), needs processing |
Azonexus, Comamonadaceae | H2-oxidizing bacteria, proteobacteria | In perspective, food or feed | H2, CO2, O2, N2 | Startups: Air Protein (USA), Solar Foods (FI), Deep Branch (UK) | Still in R&D |
Arthrospira maxima, A. platensis | Previously “Spirulina”, bacteria: Cyanobacteria (“microalgae”) | Dietary supplement, food of Aztecs and towns of Chad | CO2, light | BlueBio Tech (D), Cyanotech (Hawaii USA), FEBICO (Taiwan), Parry Nutraceuticals (India) | Possible contamination with toxins (microcystins) from other cyanobacteria, and by heavy metals |
Aphanizomenon flos-aquae(AFA) | Bacteria: Cyanobacteria | Dietary supplement, positive effects on health | CO2, light | Blue Green Foods (USA), E3Live (USA), Klamath Valley (USA) | Some toxic strains |
Chlorella | Green microalgae: Chlorophyta | Dietary supplement | CO2, light | TerraVia (USA), Roquette Klötze (D), FEBICO (Taiwan), BlueBioTech (D) | Expensive production: carbonated water. Indigestible cell wall |
Saccharomyces cerevisiae | Single-celled fungi (yeasts), ascomycetes | Yeast extract: dietary supplement | Molasses, cereals hydrolysate | Bega Cheese (AUS), Flint Hills Resources (USA) | Occasional: tyramine migraines, irregular digestions, intolerance in patients with irritable bowel * |
Torula utilis | Cyberlindnera jadinii (sin. Candida utilis), yeasts, ascomycetes | Flavouring supplement, alternative to glutamate | Methanol, molasses | Phillips Petroleum Co (USA) until 2002, Provesteen Process (USA) until 1990 | Low profitability of the process |
Fusarium venenatum | Filamentous fungi, ascomycetes | “Mycoprotein”, cell wall rich in glucans (fibre), positive health effects | Glucose from starch, salts, inorganic N | Marlow Foods Ltd “QuornTM” (UK), Atlastfood (USA), Nature’s Fynd (USA) and others, in operation | Occasional appearance of mutants with highly branched mycelium, which make it necessary to stop the continuous culture every 6 weeks. |
Paecilomyces varioti | Filamentous fungi, ascomycetes | Animal feed | Sugars from lignocellulosic waste | Finnish paper factories, “Pekilo”, 1970-1990 | It wasn’t profitable, now reviving: https://www.eniferbio.fi/product/ |
As we can see, there are a few products that are microalgae or cyanobacteria, all of them photosynthetic microorganisms, which are interesting for their low production cost as they only need light and a little CO2 and some salts. Of these, we should highlight those known as Spirulina, which are cyanobacteria and have been a food source since the time of the Aztecs and other peoples of Central America, as well as the peoples around Chad. Today it is used as a human food supplement, in the form of tablets or powders (Figure 5) and is also used as a food supplement in the aquaculture and poultry industries. One of the main benefits is the high content of vitamin B12. However, it is necessary to monitor the product well as there may occasionally be contamination with cyanotoxins or the presence of pesticides and other toxic compounds, especially if it is consumed regularly (Grosshagauer et al., 2020).
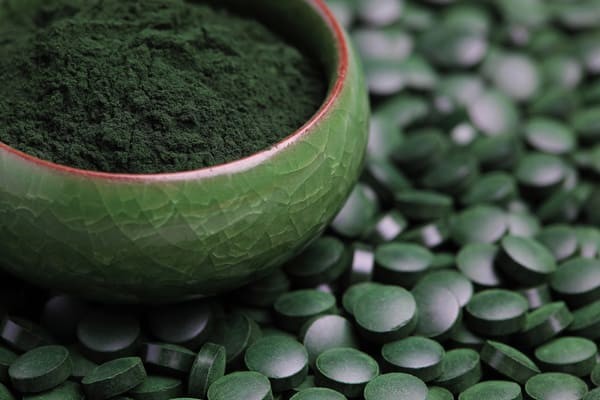
Figure 5. Supplements in powders and tablets based on “Spirulina”, cyanobacteria. Image taken from Iswari.com.
Many different bacteria, apart from cyanobacteria, have been assayed, such as some Bacillus, Corynebacterium and Rhodopseudomonas, but at an industrial level those that have been most successful as SCP are the methylotrophs and/or methanotrophs (Table 1), which contain a lot of protein (50-80% of the dry weight), are rich in essential amino acids such as methionine, and with relevant amounts of lipids and vitamins. Already in the 1970s, ICI (UK) developed the product “Pruteen” with Methylophilus methylotrophus from methanol, and currently Calysta Inc. (“FeedKind” product) and other companies are producing Methylococcus and other methanotrophs by converting methane, surplus on farms, into bacterial protein (Ritala et al. 2017).
The main problem with bacteria – and fungi – is their high content of nucleic acids, especially RNA, due to their rapid growth and protein synthesis, which requires high rates of transcription and translation. This is not the case with photosynthetics (microalgae and cyanobacteria) which grow more slowly. Ingestion of RNA-derived purines increases plasma uric acid concentration, which can lead to gout and kidney stones. Therefore, it is necessary to partially remove these nucleic acids during the SCP preparation process. The most common method is the combination of mild heat treatment with the use of ribonucleases (Ritala et al. 2017).
A promising case of bacteria are hydrogen-oxidizing chemolithotrophs that are also nitrogen (N2) fixers, such as Azonexus and the Comamonadaceae. They are known as “air-munching microbes” (De Sousa 2021) because they can grow only with N2, O2, CO2 and H2 because they oxidize hydrogen with O2 and fix atmospheric N2 (Figure 6). But since H2is almost non-existent in the atmosphere, it is necessary to provide it, obtaining it by hydrolysis of water with green energies. The main advantage of being N2 fixers is the saving of ammonium, the production of which requires a lot of energy, apart from the yield in protein (Hu et al., 2020).
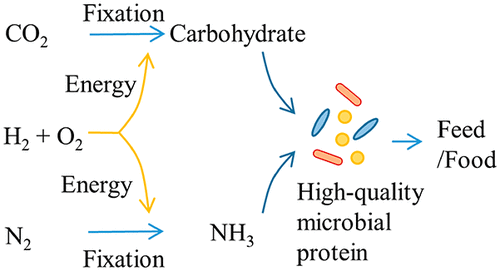
Figure 6. Scheme of the metabolism of nitrogen-fixing hydrogen-oxidizing bacteria. Taken from Hu et al. (2020).
Fungi and yeasts dominate the global SCP market for human consumption, as yeasts in particular have a long history of acceptance, especially in the form of extracts. Logically, most yeasts marketed as SCP are Saccharomyces cerevisiae, but there are also Torula utilis, Candida and Kluyveromyces. Yeasts and other fungi also have the drawback of nucleic acid content (10%), lower than bacteria, but which also requires processing to reduce them.
The mycelial fungus most used as SCP is undoubtedly Fusarium venenatum, mostly marketed under the name QuornTM by Marlow Foods Ltd. since 1985. In fact, it is the only SCP product used exclusively for human consumption. Like other fungi, apart from the high content of protein (mycoprotein), it is a good source of essential amino acids, vitamins and especially glucans, which contribute to the contribution of fibre to the diet. It is an ascomycete, considered a microfungus (Figure 7) due to the absence of macroscopic fruiting bodies, as are also Penicillium, Aspergillus and many other non-mushroom filamentous fungi.
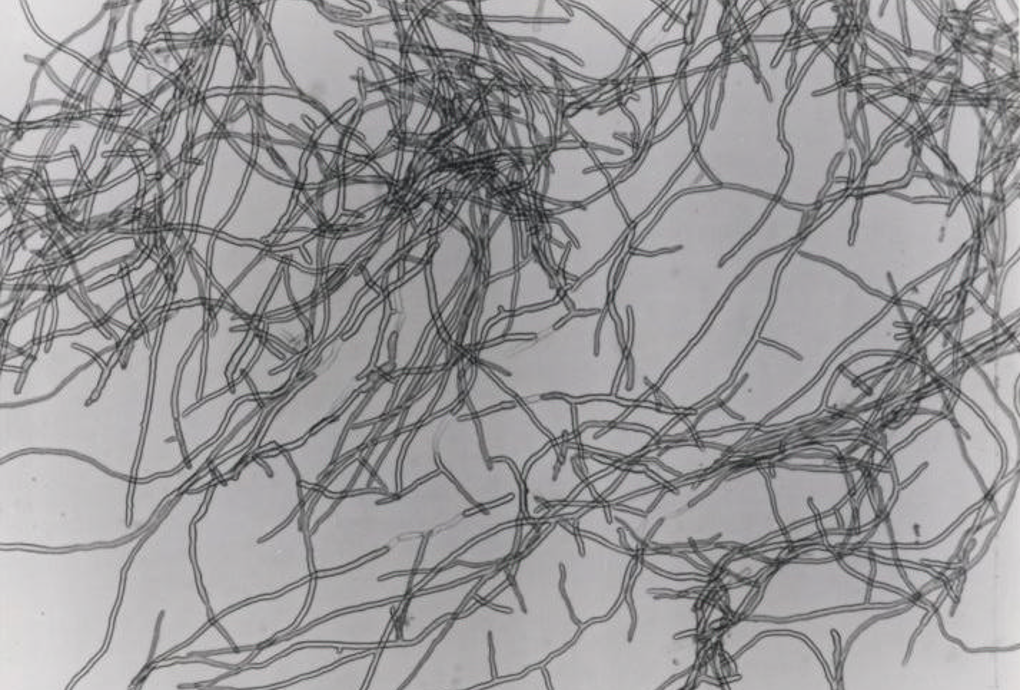
Figure 7. Electron micrograph (350 x) of the mycelium of Fusarium venenatum on the surface of Quorn product. Image taken from Ugalde & Castrillo (2002).
The production of F. venenatum is carried out in continuous bioreactors with aeration in an aqueous medium with glucose obtained by starch hydrolysis, a source of N, vitamins and minerals. The resulting mycelium is extracted (Figure 8) and treated to remove RNA, and dried.
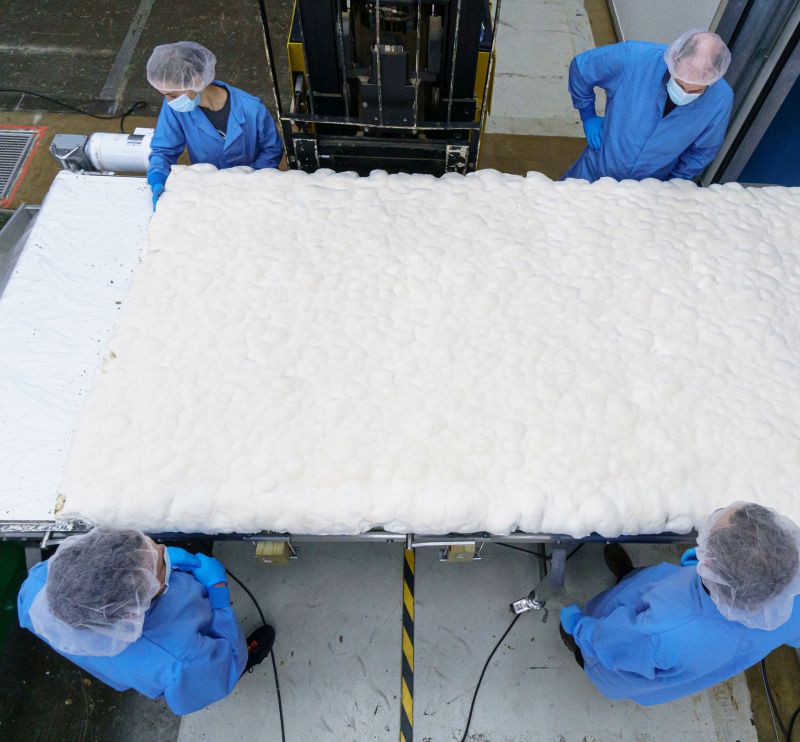
Figure 8. Preparing a Fusarium mycelium layer. Photo by MyForest Foods Co, taken from Dietrich (2022).
Products with mycoprotein such as Quorn in particular are intended mainly as a substitute for meat and are sold mainly in the form of prepared dishes, with an enormous variety of tastes and textures (Figure 9), logically adding additives. As a curiosity, see Quorn’s advertising website (www.quorn.co.uk), full of recipes and suggestions. Most products contain egg albumin in addition to the fungus, which acts as a binder. Vegan formulations replace the egg with potato.
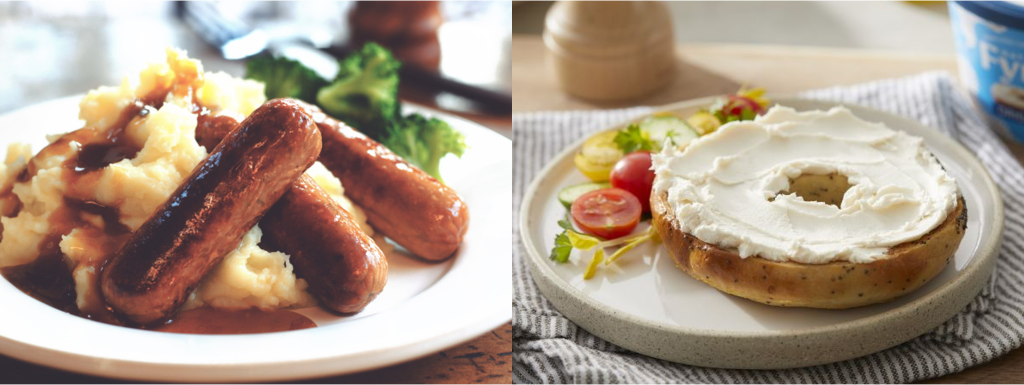
Figure 9. Examples of products made with F. venenatum: Quorn pseudosausages and Nature’s Fynd non-dairy cream cheese. Images courtesy of quorn.co.uk and Dietrich 2022.
Interest in SCP is growing, and related research and development is increasing greatly, especially in China, where 70% of the world’s SCP patents since 2001 have been registered, often related to the exploitation of agricultural waste such as methane (Ritala et al. 2017).
In any case, microbial protein must be considered as one more of the elements to take into account, in addition to the others mentioned, in the necessary transformation of the agri-food system, combining it with the reduction of food waste, the incentive to eat more healthy, and the marketing of products with less environmental impact than the current ones (Carrington 2022).
—————–
BIBLIOGRAPHY
Berkheiser K (2019) Four potential side effects of nutritional yeast. Nutrition, Healthline, 8 aug 2019.
Carrington D (2022) Swapping 20% of beef for microbial protein “could halve deforestation” The Guardian News website, Food, 4 May 2022.
Bingham L (2020) Cultured meat: better than the real thing ? Foodunfolded, 10 march 2020.
De Sousa A (2021) How air-munching microbes could grow the fake meat of the future. Bloomberg Europe edition website, 11 October 2021.
Dietrich T (2022) Microbes and mushrooms -the future of Earth-friendly food. National Science Foundation website, 20 January 2022.
Godwin R (2021) If we want to save the planet, the future of food is insects. The Guardian News website, The Observer Food, 8 May 2021.
Grosshagauer S, Kraemer K, Somoza V (2020) The true value of Spirulina. J Agric Food Chem 68:4109.
Hidrofílic (2017) Petjada hídrica. Aigües.net, 13/01/2017.
Hooper R (2022) Farming is the most destructive human activity ever (Interview with George Monbiot). New Scientist website, 19 May 2022.
Hu X, Kerckhof FM, Ghesquière J, Bernaerts K, Boeckx P, Clauwaert P, Boon N (2020) Microbial Protein out of Thin Air: Fixation of Nitrogen Gas by an Autotrophic Hydrogen-Oxidizing Bacterial Enrichment. Environ Sci Tech 54:3609
Humpenöder, F., Bodirsky, B.L., Weindl, I. et al. (2022) Projected environmental benefits of replacing beef with microbial protein. Nature 605, 90–96.
Junaid F, Khawaja LA, Sikander A (2020) Single cell protein as a potential meat substitute: a critical review. World J Pharmac Res 9:141.
Kadim IT, Mahgoub O, Baqir S, Faye B, Purchas R (2015) Cultured meat from muscle stem cells: a review of challenges and prospects. J Integr Agric 14, 222–233.
Lange KW, Nakamura Y (2021) Edible insects as future food: chances and challenges. J Future Foods, 1, 38-46
Mekonnen MM, Hoekstra AY (2010) The green blue and grey water footprint of farm animals and animal products. Value of Water Research Report Series n. 48, UNESCO-IHE
Petrusán JI, Rawel H, Huschek G (2016) Protein-rich vegetal sources and trends in human nutrition: A review. Curr Topics Pept Prot Res 17, 1-19
Poore J, Nemecek T (2018) Reducing food’s environmental impacts through producers and consumers. Science 360, 987–992
Quorn: https://www.quorn.co.uk
Ritala A, Häkkinen ST, Toivari M, Wiebe MG (2017) Single cell protein —State-of-the-Art, Industrial landscape and Patents 2001-2016. Front Microbiol 8, 2009
Ugalde UO, Castrillo JL (2002) Single cell proteins from fungi and yeasts. Appl Mycol Biotech 2:123
Velsid (2022) Producción de filetes de pescado a partir de la técnica para cultivar organoides. Gastronomía y Cía, República. 8 set 2022
Wolbachia, the “feminist” bacterium, is effective against dengue and other viruses
13th September 2021
Translated from the original article in Catalan
WHAT IS Wolbachia ?
Wolbachia is a genus of endosymbiont (intracellular) bacteria in insects and other invertebrates, that was discovered to be involved in the phenomenon of cytoplasmic incompatibility, whereby some crosses between insects infected with the bacterium give distortions in the sex ratio or cause the death of the embryo. Although they could not be isolated in culture media, PCR amplification allowed 16S rRNA genes to be sequenced and identified these bacteria as members of the Rickettsiales order within the alpha-proteobacteria phylum (O’Neill et al. 1992). As I commented in another post about bacterial phyla, all bacteria of this order, as well as Rickettsia – a human pathogen – are obligate endosymbionts of eukaryotic cells, and the phylogenetic relationship suggests that mitochondria – also endosymbionts – developed from this group.
As we see (Figure 1, Figure 2), these Wolbachia are pleomorphic, with a predominantly rounded shape, measuring 0.2 to 4 micrometers, and are resident in host vacuoles. They are gram-negative, with an approximate genome of 1 Mb and about 1000 genes (Taylor et al. 2018). The genus Wolbachia was identified in 1925 by M. Hertig and S.B. Wolbach in the common mosquito Culex pipiens and already described it as intracellular and apparently infected only the gonads of the insect (Hertig & Wolbach 1924).
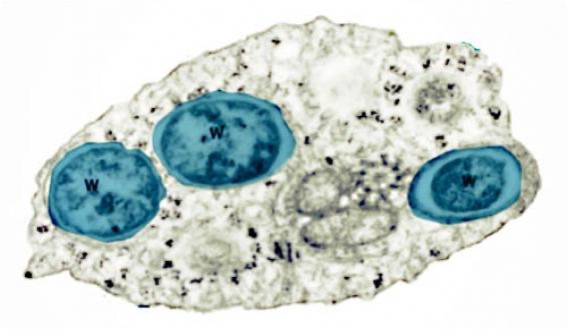
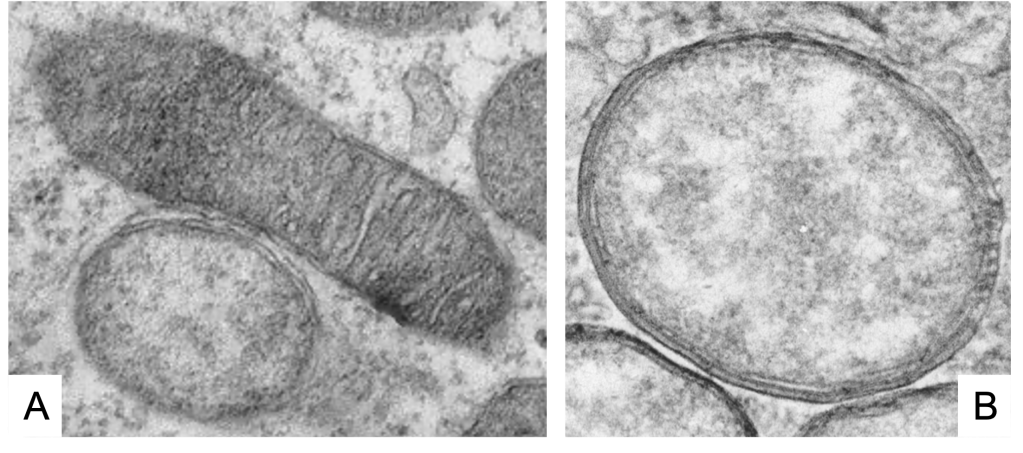
Wolbachia is found in 60% of insect species, and in other invertebrates, such as arachnids, nematodes, isopods (crustaceans) and others. In fact, it is the most common parasitic bacterium in the animal world (LePage & Bordenstein 2013). The most common species is W. pipientis, of which the complete genomic sequence is currently available (Wu et al. 2004b). They are always endosymbionts; they are never found in the environment. Host interactions are often complex and, in some cases, more mutualistic than parasitic (Taylor et al. 2018). Wolbachia is transmitted to the offspring of insects mostly vertically by infected females. It can sometimes be transmitted horizontally, as has been seen in Trichogramma wasps where they can be transmitted by contagion (Schilthuizen & Stouthamer 1997).
Since the early 1980s these bacteria have been described as cytoplasmic “feminizing sexual factors” (Bull 1983) in various invertebrates, such as insects and isopods. In this way, relevant work has been made with Armadillidium vulgare, known as pill-bugs or common woodlouse, where Martin et al (1973) already said that these factors were microorganisms, and then Rigaud & Juchault (1992) already suggested that they must be Wolbachia. These bacteria caused males to become functional females, who had mostly female offspring. Intracellular Wolbachia alter the reproductive biology of the animal host in several ways, as we will see below. For all this and as you see in the title, the joke is often made that Wolbachia is a “feminist” bacterium (Yong et al. 2016).
Wolbachia genes can also be transmitted horizontally (HGT), by transduction, by means a bacteriophage. The fact that there may be several strains of Wolbachia in the same host allows the exchange of genes between them through phages (Kent et al. 2011). This would be the first case of HGT detected in an endosymbiont, even crossing barriers between species, resulting in a global distribution in several invertebrate hosts. All of these are phenomena that contribute to evolution, and it is therefore insinuated that Wolbachia is a manipulator of invertebrate biology (Werren et al. 2008).
METHODS OF SEXUAL DIFFERENTIATION IN HOSTS CAUSED BY Wolbachia, INCLUDING FEMINIZATION
These bacteria can infect a wide variety of organs, but gonadal infections have the most phenotypic repercussions. In hosts containing Wolbachia, bacteria are always present in mature eggs but not in sperm. Therefore, only infected females are those who pass the infection to the offspring. Wolbachia maximizes its spread by significantly altering the reproductive capacity of the host, in 4 possible ways:
1- Infected males die during larval development, which increases the proportion of infected females. It has been observed in beetles and lepidoptera (Hurst et al. 1999).
2- Some infected males develop as females, there is feminization, as in some lepidoptera (Fujii et al 2001).
3- Parthenogenesis, id est, the reproduction of females, infected in this case, without males. It is also a feminizationor predominance of females. More and more cases of parthenogenesis related to the presence of Wolbachia are being found, thus even suggesting that this phenomenon would always be attributable to bacteria (Tortora et al. 2007).
The parthenogenesis of Hymenoptera is one of the best known. This order of insects (ants, bees, wasps, bumblebees, and sawflies) has a haplodiploid sex determination system. Some of them produce haploid males from unfertilized eggs (arrhenotoky), a meiotic parthenogenesis. But also, in some social Hymenoptera queens or workers produce diploid females by thelytoky, an ameiotic parthenogenesis, where the haploid egg is segmented without fertilization. Obviously, sex control of offspring is a key factor in the evolution of colonial structures in these social insects (Pearcy 2004). Parthenogenesis is not unique to Hymenoptera, as other insects and animals have it (Wrensch & Ebbert 1993).
The parthenogenesis caused by Wolbachia in the Trichogramma wasp has been extensively studied. The bacterium is in the cytoplasm of the wasp’s eggs where it induces the duplication of gametes, giving rise to a generation of all females, id est, it is a complete parthenogenesis, of thelytoky type (Schilthuizen & Stouthamer 1997, Huigens & Stouthamer 2003).
In addition to Wolbachia, this induction of parthenogenesis has also been observed in Cardinium (Jeong & Stouthamer 2004), another gram-negative bacterium – a Bacteroidetes in this case – that parasitizes other wasps, of the genus Encarsa (Zchori-Fein et al. 2004).
4- The cytoplasmic incompatibility (CI)
It is the inability of Wolbachia-infected males to reproduce with uninfected females or infected with another strain of the bacterium. On the left in Figure 3 we see that uninfected sperm can fertilize both infected and uninfected eggs with Wolbachia, and on the right we see how sperm modified by Wolbachia infection when fertilizing eggs that do not contain the bacterium, CI is induced, and the embryo dies and does not come to end.
This CI has been linked to deficiencies in the first mitotic division of the embryo, with errors in the opening of the paternal nuclear membrane, instability of maternal histones on the paternal DNA, and slow replication of this DNA, in addition to defects in paternal chromosomes (LePage & Bordenstein 2013).
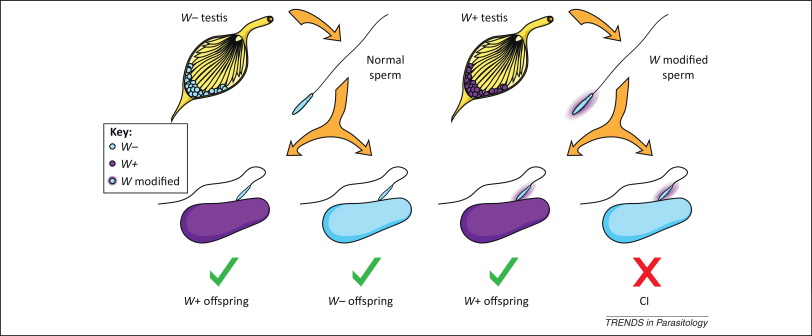
This unidirectional incompatibility implies a clear increase in the offspring of Wolbachia-infected females, making it a positive bacterial selection mechanism. However, this CI mechanism does not influence the sex of the embryo.
Many of these effects of sexual differentiation, and especially the feminization and death of males can lead to the formation of new species. For example, the loss of some of the sex chromosomes has been observed, and that incompatibilities due to infection with different Wolbachia strains can favour host speciation without any ecological or geographical barriers (Charlat 2003).
ADVANTAGES OF “INFECTION” WITH Wolbachia FOR THE HOST
Apart from the possible evolutionary effects just discussed, it has been found that some hosts benefit from the endosymbiotic presence of Wolbachia. So even though we talk about “infection” and “parasites,” the relationship is mostly mutualistic.
One of the advantages is the viral resistance found in Drosophila and several mosquitoes, which when infected with Wolbachia are much more resistant to RNA viruses (Hedges et al. 2008), as we will see below. Bacteria have also been observed to aid Drosophila in the metabolism of iron and some vitamins. In the case of nematodes such as filariasis, Wolbachia appears to provide the worm with some compounds necessary for its reproduction (Foster et al. 2005).
Wolbachia TO FIGHT HUMAN VIRUSES
There are quite a few human pathogenic viruses that are transmitted by mosquitoes. Informally arthropod-transmitted viruses are called arboviruses (arthropod-borne viruses). Among the mosquitoes, Aedes aegypti is the most relevant because it is the transmitting vector of several currently problematic tropical viral diseases, such as dengue, yellow fever, zika, chikungunya, West Nile virus or malaria. Many of these arbovirus diseases are poorly controlled and the causative viruses are emerging or re-emerging pathogens that produce major diseases and epidemics worldwide (Conway et al. 2014). The causes are mainly the increase in population and mobility, urbanization, and the loss of forest areas. For example, the number of symptomatic cases of dengue has doubled in the last 10 years, half of humans live in endemic areas of dengue, and it is estimated that each year there are a total of 390 million new infections and about 13,000 deaths more. This near-pandemic scenario has led the WHO to designate dengue as one of the top 10 threats to global health (Gil Ferreira et al. 2020).
Currently, most arbovirus control strategies are based on insecticides and reducing the environments in which vector mosquitoes thrive, or on non-specific symptomatic treatments of the diseases since in most of these cases there are still no affordable vaccines. However, insecticides are not specific, cause environmental toxicity, and induce resistance to these compounds. More effective and sustainable strategies are urgently needed (Gil Ferreira et al. 2020), and this is where Wolbachia comes in.
As we have seen, features of Wolbachia such as cytoplasmic incompatibility make it useful for promoting genetic drift in an insect population. The fact that Wolbachia-infected females produce offspring of both infected and non-infected males while uninfected females can only have offspring with uninfected males, results in a reproductive advantage for infected females that rapidly increases the presence of Wolbachia (Hancock et al. 2011).
Many mosquitoes, including some of the major disease-transmitting species, carry Wolbachia. For example, the before mentioned common mosquito Culex pipiens and others, carry strains of this bacterium. But in contrast, Aedes aegyptiand other species considered important in the transmission of human pathogens have almost no natural Wolbachia(Moreira et al. 2009).
Therefore, the transfection of Wolbachia into Aedes mosquitoes was carried out and successfully achieved by means of a microinjection technique in the laboratory, both in the tiger mosquito Ae. albopictus (Xi et al. 2005), as for Ae. aegypti (McMeniman et al. 2009).
The breakthrough came immediately when it was discovered that these transferred Wolbachia promoted interference with human pathogenic viruses transmitted by mosquitoes. Indeed, blockade of replication of dengue viruses, Zika, yellow fever, and Mayayo virus was demonstrated (Moreira et al. 2009). The mechanisms of this blockade appear to be diverse, such as increased production of reactive oxygen species (ROS) and competition for cellular resources – such as cholesterol – between Wolbachia and viruses. In addition, it has been observed that the bacterium stimulates the mosquito’s immune system by preventing viral infections, thereby reducing the number of mosquitoes transmitting the diseases to humans (Moreira et al. 2009, Gil Ferreira et al. 2020).
Various strategies have been applied to introduce Wolbachia-infected Aedes into populations where viruses are present, and the one that is working best is releasing a relatively small number of Wolbachia-carrying mosquitoes, both male and female, into the wild. These mosquitoes grow and pass bacteria to the wild mosquito population through the cytoplasmic incompatibility mechanism and become a majority in a few months (Figure 4).
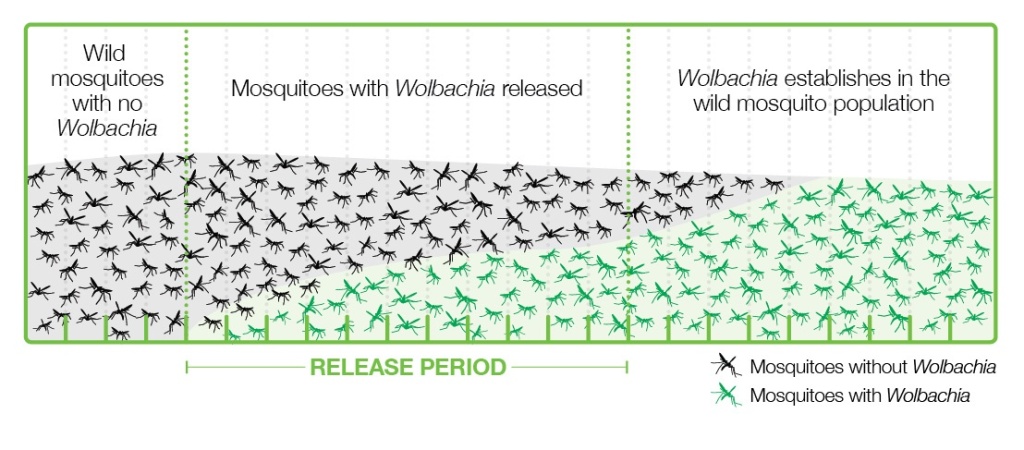
This strategy has the advantages of using natural methods, not being too expensive, easy to carry out, and sustainable, since once the mosquitoes are infected, they are mostly left without human intervention. This strategy is in line with the UN’s 2030 Agenda for Sustainable Development (Gil Ferreira et al. 2020).
In implementing this strategy with Wolbachia and mosquitoes, the World Mosquito Program (2021) should be highlighted. It began in 2004 thanks to the Bill & Melinda Gates Foundation and the NIH Foundation of the USA. In 2011 the first field trials began in Cairns, North Australia. Lots of mosquito eggs were introduced with the collaboration of community members, for 10 weeks, achieving successful mosquito-Wolbachia rates of more than 90% (World mosquito Program / Our story). This was paired with a near-practical disappearance of dengue disease (Figure 5, 1st left column) and the mosquito-Wolbachia ratio has remained stable for 8 years (Gil Ferreira et al. 2020). Subsequently, the WMP has been extended to Malaysia (Wolbadmin 2021), Indonesia (Olazo 2021), Vietnam and other countries with dengue presence and the strategy has been shown to work very well (Figure 5).
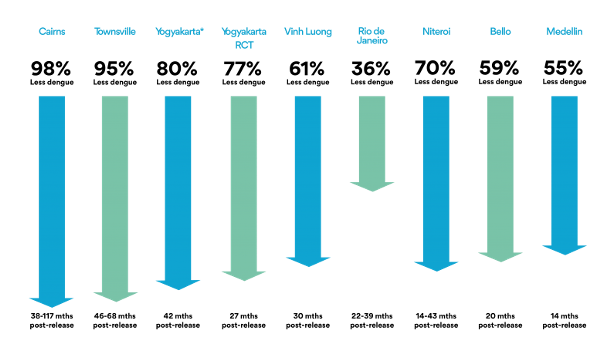
This is being one of the most successful examples of how a vector, the mosquito, can be used as an ally to fight arboviruses (Gil Ferreira et al. 2020).
On the other hand, in the case of Wolbachia-containing nematodes such as filarial worms, which cause filariasis, human tropical diseases and other domestic animals, the solution is the treatment with antibiotics such as doxycycline or others that inhibit the bacterium. In this case, an antibiotic is exceptionally used to combat an invertebrate parasite (Fundación iO 2021, Taylor et al. 2018).
BIBLIOGRAPHY
Bull JJ (1983) Evolution of Sex Determining Mechanisms. Benjamin/CummingS Publ. Co., Menlo Park, CA, USA.
Charlat S (2003) Evolutionary consequences of Wolbachia infections. Trends in Genetics 19(4):217–23
Conway MJ, Colpitts TM, Fikrig E (2014) Role of the Vector in Arbovirus Transmission. Annual Review of Virology 1:1,71-88
Foster J, Ganatra M, Kamal I, et al. (2005) The Wolbachia Genome of Brugia malayi: Endosymbiont Evolution within a Human Pathogenic Nematode. PLOS Biology 3(4): e121.
Fundación iO (2021, August 12) Enfermedades: Wolbachia. Fundación iO. Retrieved from https://fundacionio.com/salud-io/enfermedades/wolbachia/
Fujii Y, Kageyama D, Hoshizaki S, Ishikawa H, Sasaki T (2001) Transfection of Wolbachia in Lepidoptera: the feminizer of the adzuki bean borer Ostrinia scapulalis causes male killing in the Mediterranean flour moth Ephestia kuehniella. Proceedings of the Royal Society of London B: Biological Sciences 268(1469): 855–859.
Gil Ferreira A, Fairlie S, Luciano Moreira LA (2020) Insect vectors endosymbionts as solutions against diseases. Curr Opinion in Insect Sci 40, 56-61.
Hancock PA, Sinkins SP, Godfray HC (2011) Strategies for introducing Wolbachia to reduce transmission of mosquito-borne diseases. PLOS Negl. Trop. Dis. 5(4): e1024.
Hedges L, Brownlie J, O’Neill S, Johnson, K (2008) Wolbachia and Virus Protection in Insects. Science 322(5902):702
Hertig M, Wolbach SB (1924) Studies on Rickettsia-Like Micro-Organisms in Insects. Journal of Medical Research44(3):329-374.
Huigens ME, Stouthamer R (2003). Parthenogenesis associated with Wolbachia. In: Bourtzis K, Miller TA (eds) Insect Symbiosis. CRC Press: Boca Raton, FL, pp 247–266.
Hurst G, Jiggins FM, Graf von der Schulenburg JH, Bertrand D et al. (1999) Male killing Wolbachia in two species of insects. Proceedings of the Royal Society B 266 (1420): 735-740.
Jeong G, Stouthamer R (2004) Genetics of female functional virginity in the Parthenogenesis-Wolbachia infected parasitoid wasp Telenomus nawai (Hymenoptera: Scelionidae). Heredity 94 (4): 402–407.
Kent BN, Salichos L, Gibbons JG, et al. (2011) Complete Bacteriophage Transfer in a Bacterial Endosymbiont (Wolbachia) Determined by Targeted Genome Capture. Genome Biology and Evolution 3:209–218
LePage D, Bordenstein SR (2013) Wolbachia: can we save lives with a great pandemic ? Trends in Parasitology 29, 385-393.
Martin G, Juchault P, Legrand JJ (1973) Mise en evidence d’un micro-organisme intracytoplasmique symbiote de l’oniscoïde Armadillidium vulgare Latr. dont la presence accompagne l’intersexualite ou la feminisation totale des males genetiques de la lignee thelygene. C.R. Acad. Sci. Paris, 276,2312—2316.
McMeniman CJ, Lane RV, Cass BN et al. (2009) Stable introduction of a life-shortening Wolbachia infection into the mosquito Aedes aegypti. Science 323:141-144
Moreira LA, Iturbe-Ormaetxe I, Jeffery Ja, Lu G, Pyke AT, Hedges LM, Rocha BC, Hall-Mendelin S, Day A, Riegler M et al. (2009) A Wolbachia symbiont in Aedes aegypti limits infection with dengue, chikungunya, and plasmodium. Cell139:1268- 1278.
Moronta F (2021 August 12) La bacteria Wolbachia puede frenar la expansión del Zika. Félix-Moronta-Blog, 06/05/2016. Retrieved from http://felixmoronta.pro/wolbachia-zika/
Olazo A (2021, June 15). Wolbachia, la bacteria que infecta mosquitos y reduce la transmisión del dengue en un 77%. Robotitus. Retrieved from https://www.robotitus.com/wolbachia-la-bacteria-que-infecta-mosquitos-y-reduce-la-transmision-del-dengue-en-un-77
O’Neill SL, Giordano R, Colbert AME, Karr TL, Robertson HM (1992) 16S rRNA phylogenetic analysis of the bacterial endosymbionts associated with cytoplasmic incompatibility. Proc Natl Acad Sci USA 89, 2699-2702.
Pearcy, M. (2004). Conditional Use of Sex and Parthenogenesis for Worker and Queen Production in Ants. Science 306(5702): 1780–1783.
Rigaud T, Juchault P (1992) Genetic control of the vertical transmission of a cytoplasmic sex factor in Armadillidium vulgare Latr. (Crustacea, Oniscidea). Heredity 68, 47-52
Schilthuizen MO, Stouthamer R (1997) Horizontal transmission of parthenogenesis-inducing microbes in Trichogramma wasps. Proc. R. Soc. Lond. B Biol. Sci., 264, 361-366.
Taylor MJ, Bordenstein SR, Slatko B (2018) Microbe Profile: Wolbachia: a sex selector, a viral protector and a target to treat filarial nematodes. Microbiology 164(11):1345–1347
Tortora GJ, Funke BR, Case CL (2007) Microbiology: an introduction. Pearson Benjamin Cummings.
Werren JH, Baldo L, Clark ME (2008) Wolbachia: master manipulators of invertebrate biology. Nature Reviews Microbiology 6, 741-751.
Wikipedia (2021 August 12). Wolbachia. Wikimedia Foundation. Retrieved from https://en.wikipedia.org/wiki/Wolbachia
Wolbadmin (2021 August 12). What is Wolbachia. Wolbachia Malaysia. Retrieved from https://www.imr.gov.my/wolbachia/2021/05/25/what-is-wolbachia/
World Mosquito Programm (2021 August 12). The World Mosquito Program’s Wolbachia Method. World Mosquito Program. Retrieved from https://www.worldmosquitoprogram.org
Wrensch DL, Ebbert MA (1993) Evolution and Diversity of Sex Ratio in Insects and Mites. Chapman & Hall: New York and London.
Wu M et al. (2004a) Genome Sequence of the Intracellular Bacterium Wolbachia. PLoS Biology 2(3): e76.
Wu M, Sun LV, Vamathevan J, Riegler M, Deboy R et al. (2004b) Phylogenomics of the Reproductive Parasite Wolbachia pipientis wMel: A Streamlined Genome Overrun by Mobile Genetic Elements. PLoS Biology 2(3): e69.
Xi Z, Dean JL, Khoo C, Dobson SL (2005) Generation of a novel Wolbachia infection in Aedes albopictus (Asian tiger mosquito) via embryonic microinjection. Insect Biochem Mol Biol 35:903-910
Yong E (2016) I Contain Multitudes: The microbes within us a grander view of life. Ed. Penguin Random House, New York USA
Zchori-Fein E, Perlman SJ, Kelly SE, Katzir N, Hunter MS (2004). Characterization of a ‘Bacteroidetes’ symbiont inEncarsia wasps (Hymenoptera: Aphelinidae): proposal of ‘Candidatus Cardinium hertigii’. Int J Syst Evol Microbiol 54: 961–968.
Zhukova M, Voronin D, Kiseleva EV (2008) High temperature initiates changes in Wolbachia ultrastructure in ovaries and early embryos of Drosophila melanogaster. Cell and Tissue Biology 2:546-556.
Plastic-eating bacteria
25th December 2018
Translated from the original article in Catalan.
Plastic ocean
We humans are destroying the planet Earth. Besides climate change (there are still ignorant people who do not believe it), the depletion of natural resources and the massive extinction of animal and plant species, one of the most visual effects is the coverage of the planet with rubbish. Since 71% of the surface is marine, most of the non-degrading waste finishes in the sea. In the oceans there are already large expansions covered by floating debris, especially plastics, called “plastic islands” (Figure 1). In the North Pacific area, where different sea currents come together, the “island” reaches 1500 km of radius, with plastics up to 200 meters deep, and continues to grow. There is more information of it, and also about the environmental consequences, in the Wikipedia article Great Pacific garbage patch.
Figure 1. Small portion of the Great Pacific Garbage Patch (From oceanandreserveconservationalliance.com)
PET plastics
Although there are many types of plastics, one of the most used and most abundant in waste and “plastic islands” is polyethylene terephthalate, known as PET or PETE (Figure 2). It is a type of thermoplastic polymer, vulgarly plastic, which belongs to the so-called polyesters, and is obtained by synthesis from petroleum. It is harmless, very resistant and lightweight and has multiple applications (Figure 3). Counting only bottles of PET for refreshing beverages, 1 million of them per minute are sold in the world. It is a recyclable material (see Pet bottle recycling in Wikipedia) but very resistant to biodegradation. In nature it can last some hundreds of years.
Figure 2. PET, polyethylene terephthalate.
Figure 3. Several applications of PET (From http://www.technologystudent.com).
PET is “eaten” by Ideonella sakaiensis
I. sakaiensis (Figure 4) are bacteria with rod shape, gram-negative, non esporulate aerobic heterotrophic, mobile with a flagellum, and catalase (+) and oxidase (+) (Tanasupawat et al 2016). They grow at neutral pH and are mesophilic, with optimum at 30-37°C. They belong to the phylogenetic group of betaproteobacteria, which include, besides many others, the known Neisseria (gonorrhoea and meningitis) and the nitrifying Nitrosomonas.
Figure 4. Scanning electron microscope images (false colour) of Ideonella sakaiensis cells grown on PET film for 60 h (From Yoshida et al 2016).
The 201-F6 strain, the first of the new species I. sakaiensis, was isolated from a landfill and identified in 2016 by a Japanese group of the Kyoto Institute of Technology that looked for bacteria using plastic as carbon source, from samples of remains of PET bottles (Yoshida et al 2016). They saw that these bacteria adhere to a low-grade PET film and can degrade it, by means of two enzymes characterized by these authors: a PETase and a MHETase, which produce terephthalic acid and ethylene glycol acid (Figure 5), which are benign environmental substances and that the bacteria can be metabolized. A colony of I. sakaiensis completely degraded a low-grade PET bottle in 6 weeks. High-grade PET products need to be heated to weaken them before the bacteria can degrade them. This is the first bacterium found as a PET degrader, and uses it as the only carbon source and energy source. Since PET has existed only for 70 years, these bacteria should have evolved in this short period until being able to degrade PET in a few weeks, instead of hundreds of years in nature (Sampedro 2016).
Figure 5. Predicted metabolic pathway of PET degradation by I. sakaiensis: extracellular PETase hydrolyses PET giving monohydroxyethyl terephthalic (MHET) and terephthalic acid (TPA). MHETase hydrolyses MHET to TPA and ethylene glycol (EG). The TPA is incorporated through a specific transporter (TPATP) and is catabolized to cyclohexadiene and this to protocatechuic acid (PCA) by the DCDDH. Finally, the PCA ring is cut by a PCA 3.4 dioxygenase with oxygen, as known for degradation of phenolic compounds and other xenobiotics. The numbers in parentheses are the ORF of the corresponding genes (From Yoshida et al 2016).
Previously, only some tropical microfungi (Fusarium solani) were known to degrade PET, and they also excreted esterases. In this case, Fusarium would be used to modify the polyester fabric, to achieve more hydrophilic and easier to work (Nimchua et al 2008). It is important to remember the structural similarity of synthetic PET fabrics (Figure 3) to those of natural fibre such as cotton, since these contain cutin, which is a polyester, a waxy polymer from the external parts of the plants. Therefore, the enzymes of Fusarium or Ideonella must be relatively similar to those that were already in nature long before the plastics were invented.
Recent genetic improvement of the enzyme PETase of Ideonella sakaiensis
In order to better understand the function and specificity of the PETase, a group of American and British researchers have recently characterized the structure of this enzyme (Austin et al 2018), mainly by high resolution X-ray crystallography, comparing it with a homologous cutinase obtained from actinobacteria Thermobifida fusca. The main differences between the two have been a greater polarization in the surface of the PETase (pI 9.6) than in the cutinase (pI 6.3), and on the other hand (Figure 6), a greater width of the active-site cleft in the case of PETase of I. sakaiensis. The cleft widening would be related with an easy accommodation of aromatic polyesters such as PET.
Figure 6. Compared structures (left) of the PETase of I. sakaiensis (above) and the cutinase of actinobacterium Thermobifida fusca (below), obtained by high resolution X-ray crystallography (0.92 Å). The active-site cleft is marked with a red dotted circle. Details (right) of the active site with different cleft widths in the PETase of I. sakaiensis (above) and the cutinase of T. fusca (below) are shown. (From Austin et al 2018).
Hypothesizing that the structure of the active site of the PETase would have resulted from a similar cutinase in an environment with PET, Austin et al (2018) proceeded to make mutations in the PETase active-site to make it more similar to cutinase and obtained a double mutant S238F/W159H which theoretically would make the entry of the active site closer (Figure 6). But their surprise was capital when they saw that the mutant degraded the PET better (an improvement of 20%), with an erosion of the PET film (Figure 7 C) even greater than the original PETase (Figure 7B). The explanation was that mutant changes in amino acid residues favoured PET intake in the active site, despite making a closest cleft (Austin et al 2018).
Figure 7. Scanning electronic microscopy images of a piece of PET without microorganisms (A), after incubating 96 h with PETase of the I. sakaiensis 201-F6 (B), and with PETase of the double-mutant S238F/W159H (C) (From Austin et al 2018).
In addition, these authors have shown that this PETase degrades also other similar semi aromatic polyesters, such as polyethylene-2,5-furonicarboxylate (PEF), and therefore this enzyme can be considered an aromatic polyesterase, but it does not degrade aliphatic ones.
The conclusion of their work is that protein engineering is feasible in order to improve the performance of PETase and that we must continue to deepen in the knowledge of their relationships between structure and activity for the biodegradation of synthetic polyesters (Austin et al 2018).
Other plastic-eating microbes ?
The discovery of I. sakaiensis has been very important for the possibility of establishing a rapid recycling process for PET, but it is not the first organism that has been found as plastic consumer. By the way, we can see the formulas of the main plastics derived from petroleum in Figure 8.
Figure 8. Formulas of the most common petroleum plastics: polyethylene (PE), polyvinyl chloride (PVC), polypropylene (PP), polystyrene (PS), polyethylene terephthalate (PET or PETE) and polyurethane (PU) (From Shah et al 2008).
Reviewing the bibliography, we see that many cases of plastic degrading microorganisms have been described (Shah et al 2008), especially polyethylene, polyurethane and PVC: various Pseudomonas, Rhodococcus and Comamonas among bacteria, and some Penicillium, Fusarium and Aspergillus between fungi.
Among the polyurethane consumers, mushrooms are highlighted (Howard 2002), and especially the plants endophyte Pestalotiopsis microspora, which can use polyurethane as the only source of carbon (Russell et al 2011).
On the other hand, the ability of the mealworms, the larval forma of the darkling beetle Tenebrio molitor, to chew and degrade the polystyrene foam is well known (Yang et al 2015). Fed only with the PS, these larvae degrade it completely in relatively short periods. As expected, the degradation of the PS is carried out by the intestinal bacteria of the animal (Figure 9). It has been demonstrated because degradation stops when administering antibiotics to the larva (Yang et al 2015). One of the isolated bacteria that has been shown to degrade PS is Exiguobacterium, from Bacillales group, but it is not the only one. In fact, when performing studies of metagenomics from gut of larvae eating PS, a large variety of bacteria have been found, and these vary depending on the kind of plastic, since the degradation of polyethylene has also been seen. Some of the bacteria with DNA found as predominant would be the enterobacteria Citrobacter and Kosakonia. It seems that the intestinal microbiota of Tenebrio is modified and adapted to the different ingested plastics (Brandon et al 2018).
Figure 9. Biodegradation of polystyrene by the intestinal bacteria of Tenebrio, the mealworm (Yang et al 2015).
Finally, as we see the microbial biodegradation of non-biodegradable or recalcitrant plastics should not surprise us, since on the one hand, there are natural “plastics” such as polyhydroxybutyrate or polylactic acid that are easily degradable (Shah et in 2008), and on the other hand the adaptive capacity of the microorganisms to be able to break the most recalcitrant chemical bonds is very large. Microbes evolve rapidly, and acquire better strategies to break the plastics made by humans (Patel 2018). We have seen in this case the degradation of PET, which in less than 70 years some microbes have already found a way to take advantage of it.
The problem is that we are generating too much plastic waste in no time and the microorganisms have not had time yet to degrade them. It is clear that we will have to help our microbial partners, not generating more degrading polymers, and recycling and degrading them, by using these same degrading microbes, among other ways.
Bibliography
Austin HP et al (2018) Characterization and engineering of a plastic-degrading aromatic polyesterase. Proc Nat Acad Sci 115, 19, E4350-E4357
Brandon AM et al (2018) Biodegradation of Polyethylene and Plastic Mixtures in Mealworms (Larvae of Tenebrio molitor) and Effects on the Gut Microbiome. Environ Sci Technol 52, 6526-6533
Griggs MB (2017 april 24) These caterpillars chow down on plastic bags. Popular Science. http://www.popsci.com
Howard GT (2002) Biodegradation of polyurethane: a review. Int Biodeterior Biodegrad 42, 213-220
https://en.wikipedia.org/wiki/Great_Pacific_garbage_patch
https://en.wikipedia.org/wiki/PET_bottle_recycling
https://en.wikipedia.org/wiki/Polyethylene_terephthalate
Patel NV (2018 april 17) Scientists stumbled upon a plastic-eating bacterium – then accidentally made it stronger. Popular Science. http://www.popsci.com
Russell JR et al (2011) Biodegradation of polyester polyurethane by endophytic fungi. Appl Environ Microbiol 77, 17, 6076-6084
Sampedro J (2016 marzo 10) Descubierta una bacteria capaz de comerse un plástico muy común. El País
Shah AA et al (2008) Biological degradation of plastics: a comprehensive review. Biotechnol Adv 26, 246-265
Tanasupawat et al (2016) Ideonella sakaiensissp. nov., isolated from a microbial consortium that degrades poly(ethylene terephtalate). Int J Syst Evol Microbiol 66, 2813-2818
Yang et al (2015) Biodegradation and mineralization of polystyrene by plastic-eating mealworms: Part 2. Role of gut microorganisms. Environ Sci Technol 49, 12087-12093
Yoshida et al (2016) A bacterium that degrades and assimilates poly(ethylene terephthalate). Science 351,1196–1199
Lactic acid bacteria of beers: the bad guys and the good ones
28th October 2018
It is not easy to “live” in the beer
In principle, lactic acid bacteria (LAB) and many other bacteria and generally most microorganisms, do not have it easy to survive in beer or other alcoholic beverages such as wine. This is one of the main reasons why wines and beers have been from ancient times the safest ways to drink hygienically something similar to water and that it was not contaminated, apart from boiled waters, such as tea and other herbal infusions.
The reasons for the difficult survival of microorganisms in beer are ethanol, the pH quite acidic (around 4), the lack of nutrients due to the fact that the yeasts have assimilated them, the little dissolved oxygen, the high concentration of carbon dioxide (0.5% by weight / volume) and the presence of humulone derived compounds (Figure 1) of hops: iso-alpha-acids, up to 50 ppm, which are microbiocides. All these obstacles make it very difficult for any microorganism to thrive. The most susceptible beers of unwanted microbial growth are those where some of the mentioned obstacles are dampened: beers with a higher pH of 4.5, or with little ethanol or little CO2, or with added sugars – which are nutrients -, or with little amount of compounds derived from hops (Vriesekoop et al 2012).
Figure 1. Humulone (left) of the hop is degraded during beer elaboration to isohumulone (right) and other iso-alpha-acids, which are compounds bitter and microbiocides (Wikipedia; Sakamoto & Konings 2003)
The acid pH of the beer (slightly higher than the wine) inhibits many of the best-known pathogens (Figure 2). And the cases we see that could grow at this pH near 4 are inhibited by other factors such as ethanol.
Figure 2. Range of acid pH for the growth of various bacteria, compared to the typical beer pH (Menz et al 2009).
The “bad” lactic acid bacteria of beer
Despite what we have just seen, some bacteria, particularly some LAB, have been able to adapt evolutionarily to the strict beer conditions, and they can survive and spoil them. In particular, the most frequent harmful species against the quality of beers are Lactobacillus brevis and Pediococcus damnosus (Figure 3). The first is the most frequent, and it can give tastes and undesired aromas, as well as turbidity to the final product. P. damnosus has the advantage of growing at low temperatures, and it can also produce undesired aromas, such as diacetyl (Vriesekoop et al 2012). Some Pediococcus and Lactobacillus may adhere to yeast, inducing them to sediment, which delays fermentation (Suzuki 2011).
Figure 3. Lactobacillus brevis (left) and Pediococcus damnosus (right) at the electronic scanning microscope.
Some Pediococcus may also be responsible for the appearance of biological amines in some beers, at risk for the consumer. Amines in a certain concentration are toxic, they may be present in some fermented foods such as cheese, cold meat and alcoholic beverages such as wines and beers, and are produced by decarboxylation of amino acids by LAB. The level of tyramine and other amines has been used as a measure of quality in some Belgian beers made with LAB (Loret et al 2005).
Apart from these LAB, other bacteria related to problems of beer contamination are acetic acid bacteria such as Acetobacter, typically associated with oxygen intake in packaging or distribution. Other harmful bacteria are some enterobacteria, such as Shimwellia pseudoproteus or Citrobacter freundii, which proliferate in the early stages of fermentation, and produce butanediol, acetaldehyde and other unwanted aromatic compounds (Vriesekoop et al 2012). Other harmful bacteria for beer, especially when bottled, are Pectinatus and Megasphaera, which are strict anaerobes, of the clostridial family, and can produce hydrogen sulphide and short chain fatty acids, all of them unpleasant (Suzuki 2011 ).
The “good” lactic acid bacteria of beer
LAB are well known for being some of the microbes that most benefits contribute to the food production, on the one hand as an economic means of preserving food, and on the other hand to improve their quality and organoleptic characteristics. That’s why they are the main agents of fermented foods, along with yeasts. We have seen some of the LAB’s food benefits in other posts in this blog: prehistoric cheeses, or breast milk microbiota, and even wine bacteria.
Therefore, LAB also have a good role in the production of beers: in particular, as we will see below, in the production of acidified malt, and in some peculiar styles of beer such as the Belgian Lambic and the Berliner Weissbier.
As you know, malt is the raw material for making beer. The cereal is subjected to the malting process, where cereal grains, mainly barley, are germinated, the enzymes hydrolyse the starch into sugars, and all of this is then heated obtaining the must, the substrate solution which will be fermented by the yeasts ferment, producing ethanol and carbon dioxide.
The acidification of the malt, that is, with a lower pH, has the advantages of activating many important enzymes in malting, giving a lower viscosity to the malt and therefore to the final beer. Although adding mineral acids or commercial lactic acid can achieve acidification, it is often recommended or legislated a biological acidification, which is achieved by adding LAB. The use of LAB starter cultures is a relatively new process and in addition to the commented benefits on the quality of the malt, it has been shown to also inhibit unwanted molds that are a real problem in malting and that can give mycotoxins. The compounds produced by LAB that can inhibit the fungi are the same lactic acid and the consequent pH drop, bacteriocins, hydrogen peroxide, and other compounds not well known as perhaps some peptides (Lowe & Arendt 2004).
The most commonly LAB strains used to acidify malt are Lactobacillus amylolyticus previously isolated from the same malt. These strains are moderately thermophile, resistant to compounds derived from humulone, and they have the advantage of being amylolytic in addition to producing lactic acid, which lowers the pH (Vriersekoop et al 2012).
Beers with LAB participating in the fermentation, such as Lambic and Berliner Weissbier styles, belong to the type of spontaneous fermentation beers. The other types of controlled fermentation beers are the best-known Ale and Lager, both inoculated with specific yeasts. Ale beers are those of high fermentation, where Saccharomyces cerevisiae yeast used tends to remain on the surface and the fermentation temperature is above 15-20ºC. Lager ones are those of low fermentation, originally from Bavaria, where yeast S. pastorianus (S. carlsbergensis) tends to settle at the bottom of the fermenter and the temperature is between 7 and 13ºC.
Belgian Lambic beer
Traditional Belgian beers (in Dutch lambiek or lambik) are known for their sensorial characteristics due to LAB activity. They are traditional in Brussels itself and in the neighbouring region of Pajottenland, in the Zenne river valley, in the Flemish Brabant on the SW of the Belgian capital. One of the villages in this valley is Lembeek, which could be the origin of the name of this beer.
These beers of spontaneous fermentation represent the oldest style of making beer in the developed world, for some centuries. For a few years now (since around 2008), similar beers are made in the USA, called “American coolship ales” (Ray 2014).
Lambic beer is made with barley malt and a minimum of 30% of non-malted wheat. The cones of a special hops, completely dried and aged for 3 years, are added to the must. They are added not for their aroma or bitterness, but rather as antimicrobial, to prevent above all, the growth of gram-positive pathogenic bacteria in the fermentation broth.
Also to avoid these contaminants and to promote the microbiota typical of the Lambic fermentation, these beers are brewed only between October and May, since in summer there are too many harmful microorganisms in the air that could spoil the beer, and it is necessary to lower the temperature after boiling. Boiling of the must is done intensively, with an evaporation of 30%.
After boiling, the broth is left in open deposits, and in this way the microorganisms of the air present in the fermentation rooms of the brewery (usually at the top of the building) are acquired, and of the outside air, since the tradition says that the windows must be left open. It is assumed that the captured microbes are specific to the Zenne Valley. These open deposits are the koelschip in Dutch (coolship in English), like swimming pools (Figure 4). Being well open, with a lot of surface (about 6 x 6 m) and shallow depth (about 50 cm), they favour the collection of microbes from the room and from the outside. Another purpose of this form is the fastest cooling of boiled broth to start fermentation. They can be made of wood, copper, or stainless steel more recently.
Figure 4. Koelschip (in Dutch) or coolship in English, the open deposits, as swimming-pools, where the Lambic beer process begins (Brasserie Cantillon, Brussels).
The “inoculated” broth in this spontaneous way is left only one night in the coolship, and on the following day this must is pumped into fermentation tanks where there will stay a year, during which the sugar content will go down, up to about 30 g/L. Then it is transferred to oak barrels, previously used for sherry or port, and there it can be left for another two years, at temperatures of 15-25ºC. Some barrels are the same used since 100 years ago. The final product is a cloudy beer, with a pale yellow, very little CO2, dry, acidic, with about 6-8º of ethanol. It reminds a bit like the sherry and especially the cider, and with a slightly bitter taste (Jackson 1999).
In this long process of fermentation, up to 3 years, of course there is a diversity in the composition of the microbial population. In a first phase there is a certain predominance of Kloeckera yeasts and especially enterobacteria during the first month. After 2 months, Pediococcus damnosus and Saccharomyces spp. predominate, and alcoholic fermentation begins. After 6 months of fermentation the predominant yeast is Dekkera bruxellensis (Spitaels et al 2014), or what is the same, Brettanomyces (Kumara & Verachtert 1991), of which Dekkera is the sexual form.
Figure 5. Species of isolates in MRS and VRBG agar media, for lactic acid bacteria and enterobacteria respectively, during the process of making a Lambic beer. The number of isolates is given between brackets (Spitaels et al 2014).
We see (Figure 5) as in particular after 2 months the predominant bacterium is the LAB P. damnosus. It was appointed in the first studies as “P. cerevisiae“, but this name was finally not admitted because it included other species. The count of these in MRS is 104UFC per mL until the end of fermentation. Acidification seems to be rapidly taking place in the transition from the first stage to that of maturation, coinciding with the growth of P. damnosus, which produces lactic acid, although Dekkera/Brettanomyces and acetic acid bacteria also contribute to the acidification (Spitaels et to 2014).
In other trials with the American coolship ales (ACA) of Lambic style, Lactobacillus spp. have also been found, and in a metagenomic study (Bukolich et al 2012) of these ACA, DNA of several Lactobacillales has been detected. At the end of the process, a predominance of Pediococcus (Figure 6, panel C) was also observed. In the same figure in panel A we observe how the predominant unicellular fungus is also Dekkera/Brettanomyces.
Figure 6. TRFLP analysis (polymorphisms of lengths of PCR-amplified terminal restriction fragments) of total DNA extracted from the fermentation samples of ACA beers (similar to Lambic) during 3 years, using primers for: ITS1/ITS4 of 26S rDNA for yeasts (panel A), 16S rDNA for bacteria (panel B), and specific ones for LAB (panel C). Samples marked with * did not give amplification (Bukolich et al 2012).
Lambic derived beers: Gueuze, Faro, fruity and others
The basic Lambic, which is difficult to purchase, is only found in a few Brussels cafes and the production area. In fact, Lambic is the basis for elaborating the others, much more common to consume:
The Faro is a Lambic sweetened with brown sugar and sometimes with spices.
The fruity Lambic are those that have been added whole fruits or fruit syrup. They can be with bitter cherry (kriek), which are the most traditional, or with raspberry, peach, grapes, strawberry, and sometimes also apple or pineapple or apricot or other.
And finally, the Gueuze, which are sparkling and easy to find. They are made by mixing young Lambics (from 6 months to 1 year) with other more mature ones (2-3 years) in thick glass bottles similar to those of champagne or cava and left for a second fermentation with the remaining sugars from the young Lambic. This would have been begun by a mayor of Lembeek in 1870 that owned a brewery and applied the fermentation techniques in the bottle that had been successful in the Champagne some years before (Cervesa en català 2012). The word Gueuze can have the same etymological origin as gist(yeast in Flemish) and it could also refer to the fact that it produces bubbles of CO2, that is, gas (Jackson 1999). However, another historical version would be that this beer was called “Lambic de chez le gueux” (Welsh from poor people) because the mentioned mayor of Lembeek had similar socialist ideas to those of the “Parti des Gueus” founded by the Calvinists from Flanders in the 16th century to fight against the Spanish empire. And since beer is feminine in French, the gueuxfeminine is gueuze, here it is.
In this refermentation in the bottle the populations of Dekkera/Brettanomycesand LAB are maintained, although other unicellular fungi such as Candida, Hansenula, Pichia or Cryptococcus (Verachtert & Debourg 1999) appear in limited numbers.
Figure 7. Several beer Gueuze and fruity Lambic, mostly Belgian (from www.swanbournecellars.com.au/).
The Berliner Weissbier (Figure 8) is another beer relatively similar to Lambic ones. It is also brewed with an important part of wheat must, it is cloudy, acidic and with 3% ethanol. It is traditional in Berlin and the north of Germany, made from the s. XVI and the most popular alcoholic beverage in Berlin until the end of the s. XIX. It was called the “northern champagne” by the Napoleon’s soldiers. Spontaneous fermentation of must involves a mixture of Dekkera/Brettanomyces, Saccharomycesand hetero-fermentative Lactobacillus.
Figure 8. Berliner Weisse beer (from G-LO, @boozedancing wordpress).
Beers similar to Lambic brewed in Spain
In the same way that the commented American Coolship Ales, Lambic style beers are also made in many other countries and, in the case of Spain, coinciding with the boom of artisanal beers, they are also elaborated, especially the fruity Lambic ones. According to the Birrapedia website, 6 of these are currently being processed, all of which are cherries. Two of them are made in Lleida, one in Barcelona, one in Alicante, one in the Jerte valley, and another in Asturias.
Resistance of lactic acid bacteria from beer to hop compounds
Lactobacillus and Pediococcus, both bad and good we have seen, and other contaminating bacteria of beers, have the ability to withstand hop compounds, which, as we have seen, are natural microbiocides. This resistance can be due to various defence systems, both active and passive (Sakamoto & Konings 2003). The active systems include efflux pumps, such as HorA and HorC, which carry the iso-alpha-acids (Figure 1) out the cell. HorA does it with ATP consumption, and HorC using the proton driving force (Figure 9). The corresponding genes horA and horC were originally found in L. brevis, but later they were also found in L. lindneri, L. paracollinoides and in the best known P. damnosus(Suzuki et al., 2006).
Curiously, HorA shows a resemblance of 54% to OmrA, a membrane transporter of Oenococcus oeni, related to the tolerance of this bacterium from wine to ethanol and other stressors (Bourdineaud et al 2004) (See some more about O. oeni in my post on the bacteria of the vine and the wine). Therefore, it is probable that HorA also has functions of exclusion of other compounds aside from those of the hops. It has been seen that these horAand horC resistance genes and their flanking regions are well preserved and have sequences almost identical to the different species that have them. Therefore, it is very likely that some have been acquired from others by means of horizontal gene transfer, by plasmids or transposons, as is usual in many other bacteria (Suzuki 2011).
Figure 9. Mechanisms of resistance to hop compounds in Lactobacillus brevis (Suzuki 2011).
As we see in Figure 9, protons are pumped out by an ATPase, and the consumption of ATPs is compensated by forming it thanks to the consumption of substrates such as citrate, malate, pyruvate or arginine. Another mechanism of resistance, passive in this case, is the modification of the composition of membrane fatty acids, with the addition of more saturated ones, such as C16:0, which reduces the membrane fluidity and makes it difficult the entrance of the hop compounds. This also reminds us of the changes in membrane of O. oeni related to the resistance to ethanol (Margalef-Català et al 2016). The cell wall also changes its composition in the presence of the hop alpha-iso-acids, increasing the amount of high molecular weight lipoteichoic acid, which would also be a barrier. We also see (Figure 9) how hop compounds can lower the intracellular levels of Mn2+, and then a greater synthesis of Mn-dependent proteins is observed, and a greater capture of Mn2+ from outside. Finally, cells of L. brevis reduce their size when they are in beer (Figure 10), probably in order to decrease the extracellular surface, thus minimizing the effect of external toxic compounds (Suzuki 2011).
Figure 10. Effects of beer adaptation (left) in the size of Lactobacillus brevis cells compared to well grown cells in rich media MRS (right). The bars are 5 mm (Suzuki 2011).
All these mechanisms have been studied in L. brevis strains harmful to beer, but it is assumed that the resistance of beneficial bacteria from Lambic and others would be due to the same mechanisms, since they are of the same bacterial species.
As a conclusion to all said, we see that LAB have outstanding roles as beneficial in various aspects of brewery and malting, despite their most known role of harmful in the processing of the most common beers.
Bibliography
Birrapedia (seen 18 august 2018) Cervezas de tipo Fruit Lambic elaboradas en España. https://birrapedia.com/cervezas/del-tipo-fruit-lambic-elaboradas-en-espana
Bokulich NA et al (2012) Brewhouse resident microbiota are responsible for multi-stage fermentation of American Coolship Ale. PLoS One, 7, e35507
Bourdineaud J et al (2004) A bacterial gene homologous to ABC transporters protect Oenococcus oeni from ethanol and other stress factors in wine. Int J Food Microbiol 92, 1-14.
Cervesa en català (2012) Fitxes de degustació – Timmermans Gueuze Tradition http://cervesaencatala.blogspot.com.es/2012/06/fitxes-de-degustacio-timmermans-gueuze.html
Jackson, Michael (1999) Belgium’s great beers. Beer Hunter Online, July 30, 1999
Kumara HMCS & Verachtert H (1991) Identification of Lambic super attenuating micro-organisms by the use of selective antibiotics. J Inst Brew 97, 181-185
Loret S et al (2005) Levels of biogenic amines as a measure of the quality of the beer fermentation process: data from Belgian samples. Food Chem 89, 519-525
Lowe DP & Arendt EK (2004) The use and effects of lactic acid bacteria in malting and brewing with their relationships to antifungal activity, mycotoxins and gushing: a review. J Inst Brew 110, 163-180
Margalef-Català et al (2016) Protective role of glutathione addition against wine-related stress in Oenococcus oeni. Food Res Int 90, 8-15
Menz G et al (2009) Pathogens in beer, in Beer in Health and Disease Prevention, (Preedy, V. R. Ed.), 403–413, Academic Press, Amsterdam
Ray AL (2014) Coolships rising: the next frontier of sour beers in the U.S. First we feast 27 feb 2014
Sakamoto K & Konings WN (2003) Beer spoilage bacteria and hop resistance. Int J Food Microbiol 89, 105-124
Spitaels F et al (2014) The microbial diversity of traditional spontaneously fermented lambic beer. PLOS One 9, 4, e95384
Suzuki K et al (2006) A review of hop resistance in beer spoilage lactic acid bacteria. J Inst Brew 112, 173-191
Suzuki K (2011) 125th Anniversary Review: microbiological instability of beer caused by spoilage bacteria. J Inst Brew 117, 131-155
The Beer Wench (2008) My obsession with wild beers. Nov. 20, 2008 https://thecolumbuswench.wordpress.com/tag/lambic/
Verachtert H & Debourg A (1999) The production of gueuze and related refreshing acid beers. Cerevisia, 20, 37–41
Vriesekoop F et al (2012) 125th Anniversary review: Bacteria in brewing: the good, the bad and the ugly. J Inst Brew 118, 335-345
Bacillus as probiotics
12th August 2017
The probiotics
Probiotics are living microorganisms that, when ingested in adequate amounts, can have a positive effect on the health of guests (FAO / WHO 2006; World Gastroenterology Organization 2011, Fontana et al., 2013). Guests can be humans but also other animals. Lactic acid bacteria, especially the genus Lactobacillus and Bifidobacterium, both considered as GRAS (Generally recognized as safe), are the microbes most commonly used as probiotics, but other bacteria and some yeasts can also be useful. Apart from being able to be administered as medications, probiotics are commonly consumed for millennia as part of fermented foods, such as yoghurt and other dairy products (see my article “European cheese from 7400 years ago..” “December 26th, 2012). As medications, probiotics are generally sold without prescription, over-the-counter (OTC) in pharmacies.
I have already commented on the other posts of this blog the relevance of probiotics (“A new probiotic modulates microbiota against hepatocellular carcinoma” August 24th, 2016), as well as the microbiota that coexists with our body (“Bacteria in the gut controlling what we eat” October 12th, 2014; “The good bacteria of breast milk” February 3rd, 2013) and other animals (“Human skin microbiota … and our dog” December 25th, 2015; “The herbivore giant panda …. and its carnivore microbiota” September 30th, 2015).
Besides lactic acid bacteria and bifidobacteria, other microorganisms that are also used to a certain extent as probiotics are the yeast Saccharomyces cerevisiae, some strains of Escherichia coli, and some Bacillus, as we will see. Some clostridia are also used, related to what I commented in a previous post of this blog by March 21st, 2015 (“We have good clostridia in the gut ...”).
The Bacillus
In fact, Bacillus and clostridia have in common the ability to form endospores. And both groups are gram-positive bacteria, within the taxonomic phylum Firmicutes (Figure 1), which also includes lactic acid bacteria. However, bacilli (Bacillus and similar ones, but also Staphylococcus and Listeria) are more evolutionarily closer to lactobacillalles (lactic acid bacteria) than to clostridia ones. The main physiological difference between Clostridium and Bacillus is that the first are strict anaerobes while Bacillus are aerobic or facultative anaerobic.
Figure 1. Phylogenetic tree diagram of Gram-positive bacteria (Firmicutes and Actinobacteria). Own elaboration.
Bacterial endospores (Figure 2) are the most resistant biological structures, as they survive extreme harsh environments, such as UV and gamma radiation, dryness, lysozyme, high temperatures (they are the reference for thermal sterilization calculations), lack of nutrients and chemical disinfectants. They are found in the soil and in the water, where they can survive for very long periods of time.
Figure 2. Endospores (white parts) of Bacillus subtilis in formation (Image of Simon Cutting).
Bacillus in fermented foods, especially Asian
Several Bacillus are classically involved in food fermentation processes, especially due to their protease production capacity. During fermentation, this contributes to nutritional enrichment with amino acids resulting from enzymatic proteolysis.
Some of these foods are fermented rice flour noodles, typical of Thailand and Burma (nowadays officially Myanmar). It has been seen that a variety of microorganisms (lactic acid bacteria, yeasts and other fungi) are involved in this fermentation, but also aerobic bacteria such as B. subtilis. It has been found that their proteolytic activity digests and eliminates protein rice substrates that are allergenic, such as azocasein, and therefore they have a beneficial activity for the health of consumers (Phromraksa et al. 2009).
However, the best-known fermented foods with Bacillus are the alkaline fermented soybeans. As you know, soy (Glycine max) or soya beans are one of the most historically consumed nourishing vegetables, especially in Asian countries. From they are obtained “soy milk”, soybean meal, soybean oil, soybean concentrate, soy yogurt, tofu (soaked milk), and fermented products such as soy sauce, tempeh, miso and other ones. Most of them are made with the mushroom Rhizopus, whose growth is favoured by acidification or by direct inoculation of this fungus. On the other hand, if soy beans are left to ferment only with water, the predominant natural microbes fermenting soy are Bacillus, and in this way, among other things, the Korean “chongkukjang” is obtained, “Kinema” in India, the “thua nao” in northern Taiwan, the Chinese “douchi”, the “chine pepoke” from Burma, and the best known, the Japanese “natto” (Figure 3). Spontaneous fermentation with Bacillus gives ammonium as a by-product, and therefore is alkaline, which gives a smell not very good to many of these products. Nevertheless, natto is made with a selected strain of B. subtilis that gives a smoother and more pleasant smell (Chukeatirote 2015).
These foods are good from the nutritional point of view as they contain proteins, fibre, vitamins, and they are of vegetable or microbial origin. In addition, the advertising of the commercial natto emphasizes, besides being handmade and sold fresh (not frozen), its probiotic qualities, saying that B. subtilis (Figure 4) promotes health in gastrointestinal, immunologic, cardiovascular and osseous systems (www.nyrture.com). They say the taste and texture of natto are exquisite. It is eaten with rice or other ingredients and sauces, and also in the maki sushi. We must try it !
Figure 3. “Natto”, soybeans fermented with B. subtilis, in a typical Japanese breakfast with rice (Pinterest.com).
Figure 4. Coloured electronic micrograph of Bacillus subtilis (Nyrture.com).
Bacillus as probiotics
The endospores are the main advantage of Bacillus being used as probiotics, thanks to their thermal stability and to survive in the gastric conditions (Cutting 2011). Although Clostridium has also this advantage, its strict anaerobic condition makes its manipulation more complex, and moreover, for the “bad reputation” of this genus due to some well-known toxic species.
Unlike other probiotics such as Lactobacillus or Bifidobacterium, Bacillus endospores can be stored indefinitely without water. The commercial products are administered in doses of 10^9 spores per gram or per ml.
There are more and more commercial products of probiotics containing Bacillus, both for human consumption (Table 1) and for veterinary use (Table 2). In addition, there are also five specific products for aquaculture with several Bacillus, and also shrimp farms are often using products of human consumption (Cutting 2011).
For use in aquaculture, probiotic products of mixtures of Bacillus (B. thuringiensis, B. megaterium, B. polymixa, B. licheniformis and B. subtilis) have been obtained by isolating them from the bowel of the prawn Penaeus monodon infected with vibriosis. They have been selected based on nutrient biodegradation and the inhibitory capacity against the pathogen Vibrio harveyi (Vaseeharan & Ramasamy 2003). They are prepared freeze-dried or microencapsulated in sodium alginate, and it has been shown to significantly improve the growth and survival of shrimp (Nimrat et al., 2012).
As we see for human consumption products, almost half of the brands (10 of 25) are made in Vietnam. The use of probiotic Bacillus in this country is more developed than in any other, but the reasons are not clear. Curiously, as in other countries in Southeast Asia, there is no concept of dietary supplements and probiotics such as Bacillus are only sold as medications approved by the Ministry of Health. They are prescribed for rotavirus infection (childhood diarrhoea) or immune stimulation against poisoning, or are very commonly used as a therapy against enteric infections. However, it is not clear that clinical trials have been carried out, and they are easy-to-buy products (Cutting 2011).
Table 1. Commercial products of probiotics with Bacillus, for human consumption (modified from Cutting 2011).
Product | Country where it is made | Species of Bacillus |
Bactisubtil ® | France | B. cereus |
Bibactyl ® | Vietnam | B. subtilis |
Bidisubtilis ® | Vietnam | B. cereus |
Bio-Acimin ® | Vietnam | B. cereus and 2 other |
Biobaby ® | Vietnam | B. subtilis and 2 other |
Bio-Kult ® | United Kingdom | B. subtilis and 13 other |
Biosporin ® | Ukraine | B. subtilis + B. licheniformis |
Biosubtyl ® | Vietnam | B. cereus |
Biosubtyl DL ® | Vietnam | B. subtilis and 1 other |
Biosubtyl I and II ® | Vietnam | B. pumilus |
Biovicerin ® | Brazil | B. cereus |
Bispan ® | South Korea | B. polyfermenticus |
Domuvar ® | Italy | B. clausii |
Enterogermina ® | Italy | B. clausii |
Flora-Balance ® | United States | B. laterosporus * |
Ildong Biovita ® | Vietnam | B. subtilis and 2 other |
Lactipan Plus ® | Italy | B. subtilis * |
Lactospore ® | United States | B. coagulans * |
Medilac-Vita ® | China | B. subtilis |
Nature’s First Food ® | United States | 42 strains, including 4 B. |
Neolactoflorene ® | Italy | B. coagulans * and 2 other |
Pastylbio ® | Vietnam | B. subtilis |
Primal Defense ® | United States | B. subtilis |
Subtyl ® | Vietnam | B. cereus |
Sustenex ® | United States | B. coagulans |
* Some labelled as Lactobacillus or other bacteria are really Bacillus
Table 2. Commercial products of probiotics with Bacillus, for veterinary use (modified from Cutting 2011).
Product | Animal | Country where it is made | Species of Bacillus |
AlCare ® | Swine | Australia | B. licheniformis |
BioGrow ® | Poultry, calves and swine | United Kingdom | B. licheniformis and B. subtilis |
BioPlus 2B ® | Piglets, chickens, turkeys | Denmark | B. licheniformis and B. subtilis |
Esporafeed Plus ® | Swine | Spain | B. cereus |
Lactopure ® | Poultry, calves and swine | India | B. coagulans * |
Neoferm BS 10 ® | Poultry, calves and swine | France | B. clausii |
Toyocerin ® | Poultry, calves, rabbits and swine | Japan | B. cereus |
The Bacillus species that we see in these Tables are those that really are found, once the identification is made, since many of these products are poorly labelled as Bacillus subtilis or even as Lactobacillus (Green et al. 1999; Hoa et al. 2000). These labelling errors can be troubling for the consumer, and especially for security issues, since some of the strains found are Bacillus cereus, which has been shown to be related with gastrointestinal infections, since some of them produce enterotoxins (Granum & Lund 1997; Hong et al. 2005)
The probiotic Bacillus have been isolated from various origins. For example, some B. subtilis have been isolated from the aforementioned Korean chongkukjang, which have good characteristics of resistance to the gastrointestinal tract (GI) conditions and they have antimicrobial activity against Listeria, Staphylococcus, Escherichia and even against B. cereus (Lee et al. 2017).
One of the more known probiotics pharmaceuticals is Enterogermina ® (Figure 5), with B. subtilis spores, which is recommended for the treatment of intestinal disorders associated with microbial alterations (Mazza 1994).
Figure 5. Enterogermina ® with spores of Bacillus subtilis (Cutting 2011)
Bacillus in the gastrointestinal tract: can they survive there ?
It has been discussed whether administered spores can germinate in the GI tract. Working with mice, Casula & Cutting (2002) have used modified B. subtilis, with a chimeric gene ftsH-lacZ, which is expressed only in vegetative cells, which can be detected by RT-PCR up to only 100 bacteria. In this way they have seen that the spores germinate in significant numbers in the jejunum and in the ileum. That is, spores could colonize the small intestine, albeit temporarily.
Similarly, Duc et al. (2004) have concluded that B. subtilis spores can germinate in the gut because after the oral treatment of mice, in the faeces are excreted more spores that the swallowed ones, a sign that they have been able to proliferate. They have also detected, through RT-PCR, mRNA of vegetative bacilli after spore administration, and in addition, it has been observed that the mouse generates an IgG response against bacterial vegetative cells. That is, spores would not be only temporary stagers, but they would germinate into vegetative cells, which would have an active interaction with the host cells or the microbiota, increasing the probiotic effect.
With all this, perhaps it would be necessary to consider many Bacillus as not allochthonous of the GI tract, but as bacteria with a bimodal growth and sporulation life cycle, both in the environment and in the GI tract of many animals (Hong et al. 2005).
Regarding the normal presence of Bacillus in the intestine, when the different microorganisms inhabiting the human GI tract are studied for metagenomic DNA analysis of the microbiota, the genus Bacillus does not appear (Xiao et al., 2015). As we can see (Figure 6), the most common are Bacteroides and Clostridium, followed by various enterobacteria and others, including bifidobacteria.
Figure 6. The 20 bacterial genera more abundant in the mice (left) and human (right) GI tract (Xiao et al. 2015).
In spite of this, several species of Bacillus have been isolated from the GI tract of chickens, treating faecal samples with heat and ethanol to select only the spores, followed by aerobic incubation (Barbosa et al. 2005). More specifically, the presence of B. subtilis in the human microbiota has been confirmed by selective isolation from biopsies of ileum and also from faecal samples (Hong et al. 2009). These strains of B. subtilis exhibited great diversity and had the ability to form biofilms, to sporulate in anaerobiosis and to secrete antimicrobials, thereby confirming the adaptation of these bacteria to the intestine. In this way, these bacteria can be considered intestinal commensals, and not only soil bacteria.
Security of Bacillus as probiotics
The oral consumption of important amounts of viable microorganisms that are not very usual in the GI treatment raises additional doubts about safety. Even more in the use of species that do not have a history of safe use in foods, as is the case of sporulated bacteria. Even normal bowel residents may sometimes act as opportunistic pathogens (Sanders et al. 2003).
With the exception of B. anthracis and B. cereus, the various species of Bacillus are generally not considered pathogenic. Of course, Bacillus spores are commonly consumed inadvertently with foods and in some fermented ones. Although Bacillus are recognized as GRAS for the production of enzymes, so far the FDA has not guaranteed the status of GRAS for any sporulated bacteria with application as a probiotic, neither Bacillus nor Clostridium. While Lactobacillus and Bifidobacterium have been the subject of numerous and rigorous tests of chronic and acute non-toxicity, and a lot of experts have reviewed data and have concluded that they are safe as probiotics, there is no toxicity data published on Bacillus in relation to their use as probiotics. When reviewing articles on Medline with the term “probiotic” and limited to clinical studies, 123 references appear, but Bacillus does not appear in any of them (Sanders et al. 2003).
Instead, there are some clinical studies where Bacillus strains have been detected as toxigenic. All this explains that some probiotic Bacillus producers refer to them with the misleading name of Lactobacillus sporogenes, a non-existent species, as can be seen from NCBI (https://www.ncbi.nlm.nih.gov/taxonomy/?term = Lactobacillus + sporogenes).
Finally, we should remember the joint report on probiotics of FAO (United Nations Food and Agriculture Organization) and WHO (World Health Organization) (FAO / WHO 2006), which suggests a set of Guidelines for a product to be used as a probiotic, alone or in the form of a new food supplement. These recommendations are:
- The microorganism should be well characterized at the species level, using phenotypic and genotypic methods (e.g. 16S rRNA).
- The strain in question should be deposited in an internationally recognized culture collection.
- To evaluate the strain in vitro to determine the absence of virulence factors: it should not be cytotoxic neither invades epithelial cells, and not produce enterotoxins or haemolysins or lecithinases.
- Determination of its antimicrobial activity, and the resistance profile, including the absence of resistance genes and the inability to transfer resistance factors.
- Preclinical evaluation of its safety in animal models.
- Confirmation in animals demonstrating its effectiveness.
- Human evaluation (Phase I) of its safety.
- Human evaluation (Phase II) of its effectiveness (if it does the expected effect) and efficiency (with minimal resources and minimum time).
- Correct labelling of the product, including genus and species, precise dosage and conservation conditions.
Conclusions
The use of Bacillus as probiotics, especially in the form of dietary supplements, is increasing very rapidly. More and more scientific studies show their benefits, such as immune stimulation, antimicrobial activities and exclusive competition. Their main advantage is that they can be produced easily and that the final product, the spores, is very stable, which can easily be incorporated into daily food. In addition, there are studies that suggest that these bacteria may multiply in GI treatment and may be considered as temporary stagers (Cutting 2011).
On the other hand, it is necessary to ask for greater rigor in the selection and control of the Bacillus used, since some, if not well identified, could be cause of intestinal disorders. In any case, since the number of products sold as probiotics that contain the sporulated Bacillus is increasing a lot, one must not assume that all are safe and they must be evaluated on a case-by-case basis (Hong et al. 2005).
Bibliography
Barbosa TM, Serra CR, La Ragione RM, Woodward MJ, Henriques AO (2005) Screening for Bacillus isolates in the broiler gastrointestinal tract. Appl Environ Microbiol 71, 968-978.
Casula G, Cutting SM (2002) Bacillus probiotics: Spore germination in the gastrointestinal tract. Appl Environ Microbiol 68, 2344-2352.
Chukeatirote E (2015) Thua nao: Thai fermented soybean. J Ethnic Foods 2, 115-118.
Cutting SM (2011) Bacillus probiotics. Food Microbiol 28, 214-220.
Duc LH, Hong HA, Barbosa TM, Henriques AO, Cutting SM (2004) Characterization of Bacillus probiotics available for human use. Appl Environ Microbiol 70, 2161-2171.
FAO/WHO (2006) Probiotics in food. Health and nutritional properties and guidelines for evaluation. Fao Food and Nutrition Paper 85. Reports of Joint FAO/WHO expert consultations.
Fontana L, Bermudez-Brito M, Plaza-Diaz J, Muñoz-Quezada S, Gil A (2013) Sources, isolation, characterization and evaluation of probiotics. Brit J Nutrition 109, S35-S50.
Granum, P. E., T. Lund (1997) Bacillus cereus and its food poisoning toxins. FEMS Microbiol. Lett. 157:223–228.
Green, D. H., P. R. Wakeley, A. Page, A. Barnes, L. Baccigalupi, E. Ricca, S. M. Cutting (1999) Characterization of two Bacillus probiotics. Appl Environ Microbiol 65, 4288–4291.
Hoa, N. T., L. Baccigalupi, A. Huxham, A. Smertenko, P. H. Van, S. Ammendola, E. Ricca, A. S. Cutting (2000) Characterization of Bacillus species used for oral bacteriotherapy and bacterioprophylaxis of gastrointestinal disorders. Appl Environ Microbiol 66, 5241–5247.
Hong HA, Dic LH, Cutting SM (2005) The use of bacterial spore formers as probiotics. FEMS Microbiol Rev 29, 813-835.
Hong HA, Khaneja R, Tam NMK, Cazzato A, Tan S, Urdaci M, Brisson A, Gasbarrini A, Barnes I, Cutting SM (2009) Bacillus subtilis isolated from the human gastrointestinal tract. Res Microbiol 160, 134-143.
Lee S, Lee J, Jin YI, Jeong JC, Hyuk YH, Lee Y, Jeong Y, Kim M (2017) Probiotic characteristics of Bacillus strains isolated from Korean traditional soy sauce. LWT – Food Sci Technol 79, 518-524.
Mazza P (1994) The use of Bacillus subtilis as an antidiarrhoeal microorganism. Boll Chim. Farm. 133, 3-18.
Nimrat S, Suksawat S, Boonthai T, Vuthiphandchai V (2012) Potential Bacillus probiotics enhance bacterial numbers, water quality and growth during early development of white shrimp (Litopenaeus vannamei). Veterinary Microbiol 159, 443-450.
Phromraksa P, Nagano H, Kanamaru Y, Izumi H, Yamada C, Khamboonruang C (2009) Characterization of Bacillus subtilis isolated from Asian fermented foods. Food Sci Technol Res 15, 659-666.
Sanders ME, Morelli L, Tompkins TA (2003) Sporeformers as human probiotics: Bacillus, Sporolactobacillus, and Brevibacillus. Compr Rev Food Sci Food Safety 2, 101-110
Vaseeharan, B., P. Ramasamy (2003) Control of pathogenic Vibrio spp. by Bacillus subtilis BT23, a possible probiotic treatment for black tiger shrimp Penaeus monodon. Lett Appl Microbiol 36, 83–87
World Gastroenterology Organisation Global Guidelines (2011) Probiotics and Prebiotics.
Xiao et al. (2015) A catalogue of the mouse gut metagenome. Nature Biotechnol 33, 1103-1108.
Agromicrobiome: microorganisms from the roots of crop plants
All we that studied “Bios” probably remember two known aspects of the symbiotic relationships of plant roots with microorganisms:
1) The bacterial Rhizobium nodules on the roots of legumes (Figure 1). These bacteria, with the nitrogenase complex, are among the few organisms capable of fixing atmospheric N2 transforming it into organic nitrogen, which is used by the plant, and symbiotically, the plant provides organic compounds to the bacteria. Thanks to these bacteria, plants such as legumes do not require nitrogen fertilizers.
Figure 1. Rhizobium nodules
2) The mycorrhizae, that is, the symbiotic relationships between fungi and plant roots. The most commonly known are the mushrooms always associated with some trees (Figure 2), such as the Lactarius sanguifluus associated with pines. In fact, mycorrhizae are present in most plants. Through this symbiosis, the fungi receive organic nutrients of the plant, and this can capture more easily water and mineral nutrients (especially P, Zn and Cu) by means of the fungus. In addition, mycorrhizae increase the resistance of plants to diseases coming from the soil and facilitate them inhabiting badlands.
Figure 2. Mycorrhizae of mushrooms with trees. Image from Shannon Wright
But these are only the best known of the symbiotic relationships between microorganisms and plant roots. Indeed, as the soil is full of microorganisms, many of these, including bacteria, fungi, algae, protozoa or viruses, are beneficial, symbiotic or otherwise, for the plants. And what is biotechnologically more interesting, more potential applications of these microorganisms to benefit crop plants are being found, which can be a good alternative to the use of fertilizers and pesticides.
Different microorganisms can have direct positive effects on plant nutrition as nitrogen fixation, mineralization of organic compounds, and solubilisation of elements not available to the plant (such as phosphates, K, Fe), but also indirectly positive effects, such as the production of hormones and growth factors, or protection against pathogens (García 2013).
Thus, there is a growing interest in the biological control of plant pathogens. It has been proven that some of these pathogens are inhibited by antibiotics produced by microorganisms in the rhizosphere (Raaijmakers et al 2002). Bacteria are being used (bacterization) for some years in soil or with seeds or other plant parts, with the aim of improving the growth and health of the plant.
Some of the best known and used bacteria in this sense have been Bacillus and Paenibacillus. Several species of these genera of aerobic spore bacteria are abundant in agricultural soils and can promote plant health in different ways, suppressing pathogens with antibiotic metabolites, stimulating plant defence, facilitating nutrient uptake by the plant, or promoting symbiosis with Rhizobium or with mycorrhizae (McSpadder 2004).
The genus Paenibacillus was reclassified from Bacillus in 1993, and includes P. polymyxa, a species N2 – fixing, which is used in agriculture and horticulture. This and other Paenibacillus species give complex and regular colonial forms in agar, even surprising (Figure 3), which vary according to environmental conditions. For this, a self-organizing and cooperative behaviour between individual bacterial cells is needed, using a system of chemical communication. This bacterial social behaviour would be an evolutive precursor of multicellular organisms.
Figure 3. Colonies of Paenibacillus dendritiformis, 6 cm diameter each, branched (left) and chiral (right) morphotypes. From Wikipedia Creative Commons.
The colonization of plant roots by these bacteria has been demonstrated, and also that they do it by forming biofilms (Figure 4). The inoculation of these bacteria to the roots promotes the growth, as shown in peppers (Figure 5). This appears to be due to the nitrogen fixing bacteria, which increases the formation of plant proteins and chlorophyll, thus increasing photosynthesis and physiological activities. And on the other hand, it has been shown that these bacteria produce siderophores, which facilitate Fe uptake by the plant (Lamsal et al 2012).
Figure 4. Colonization of Paenibacillus polymyxa and biofilm formation on roots of Arabidopsis thaliana. Adapted from Timmusk et al 2005.
Figure 5. Promoting growth effect of peppers (Capsicum annuum) by inoculation with Bacillus subtilis (AB17) and Paenibacillus polymyxa (AB15), respect the non-inoculated control. From Lamsal et al. 2012.
Moreover, bacteria such as Paenibacillus can be effective against plant pathogens. For example, it has been shown that a strain of P. lentimorbus (B-30488r) reduces the incidence of disease done by the fungus Alternaria solani in tomato. It has been tested (Figure 6) that after inoculating with Paenibacillus a plant infected with Alternaria, resistance to the fungus was induced in the plant. The bacteria degraded the cell walls of the fungus and also inhibited it by competition of nutrients. In addition, it was found that Paenibacillus has no negative effect on the microbial population in the rhizosphere of tomato (Khan et al 2012). These treatments are a good alternative to the use of fungicides, avoiding the environmental and health problems of these compounds.
Figure 6. Schema of the influence of Paenibacillus lentimorbus B-30488r in the interactions of tomato plant with Alternaria solani, a fungus pathogen (Khan et al 2012).
Finally, these Paenibacillus can also be useful to avoid the transmission of human pathogens such as Salmonella through the crop plants. Indeed, on the east coast of the USA a few years ago were detected outbreaks of Salmonella on tomatoes due to contamination of water. When they analyzed the microbiome present in the roots of tomatoes and these were compared with those of other places where there were no Salmonella contamination occurred, it was found that these tomatoes of the East Coast had no Paenibacillus, which were present in tomatoes of other places. With this, they decided to inoculate tomatoes with several Paenibacillus and found that Salmonella disappeared. Among the inoculated strains, one was selected as more effective, P. alvei TS -15 , for which a patent was obtained as a biocontrol agent of foodborne human pathogens (Brown et al. 2012) .
Thus, knowledge of the soil microbiota and the many forms of relationships between microorganisms and plants lead to find new strategies for using “good” microbes to prevent food safety problems of transmission of pathogens, while at the same time it can be a good ecological alternative to the massive use of pesticides.
Bibliography
Brown EW, Zheng J, Enurach A, The Government of USA (2012) Paenibacillus alvei strain TS-15 and its use in controlling pathogenic organisms. Patent WO2012166392, PCT/US2012/038584
Conniff R (2013) Super dirt. Scientific American 309, sept, 76-79.
Conniff R (2013) Tierra prodigiosa. Investigación y Ciencia 446, nov, 68-71.
García, Sady (2013) Los microorganismos del suelo y su rol en la nutrición vegetal. Simposium Perú “Manejo nutricional de cultivos de exportación”. Slideshare.net
Khan N, Mishra A, Nautiyal CS (2012) Paenibacillus lentimorbus B-30488r controls early blight disease in tomato by inducing host resistance associated gene expression and inhibiting Alternaria solani. Biological Control 62, 65-74
Lamsal K, Kim SW, Kim YS, Lee YS (2012) Application of rhizobacteria for plant growth promotion effect and biocontrol of anthracnose caused by Colletotrichum acutatum on pepper. Mycobiology 40, 244-251.
McSpadden Gardener BB (2004) Ecology of Bacillus and Paenibacillus spp. in agricultural systems. Phytopathology 94, 1252-1258
Raaijmakers JM, Vlami M, De Souza JT (2002) Antibiotic production by bacterial biocontrol agents. Antonie van Leeuwenhoek 81, 537-547
Sánchez, Manuel. http://curiosidadesdelamicrobiologia.blogspot.com.es/2012/01/la-compania-de-transporte-paenibacillus.html
Timmusk S, Grantcharova N, Wagner EGH (2005) Paenibacillus polymyxa invades plant roots and forms biofilms. Applied and Environmental Microbiology 71, 7292-7300
Viquipèdia: http://ca.wikipedia.org/wiki/Micoriza
Wikipedia: http://en.wikipedia.org/wiki/Paenibacillus_dendritiformis