Category Archives: Lactic acid bacteria and products
The human brain developed thanks to fermented foods
21st February 2024
Translated from the original article in Catalan
Science Alert is a good free scientific newsletter in which every week I find some interesting news. A few weeks ago I was especially surprised by an article (Dyer 2023) on this hypothesis of the title. Apart from this article, to know the details I went to the original publication by Bryant et al. “Fermentation technology as a driver of human brain expansion”, which I will comment below.
LARGER BRAIN
The main distinguishing feature of humans with respect to other primates and other animals is the larger and most complex brain. Because the greater an animal, the greater the weight of the brain, a relative measure is used: the encephalization quotient (EQ), which is the relationship between the mass of the brain and the expected one for a typical animal of the same dimensions. Homo sapiens‘s EQ is around 7.5, while for other primates it is between 2 and 3, and in other mammals like the dog is between 1 and 2, except cetaceans such as orcas or dolphins, which have 3 or 4.
Therefore, the human brain tripled its size with respect to other primates in its evolution since the last Australopithecus —which were already bipedal, and therefore had their hands free— about 2.5 million years ago (Ma) until to the first Homo about half a million years later. The Australopithecus had an endocranial volume (ECV) of 400 mL (Figure 1), while for Homo erectus (Figure 2) it was about 800 mL, and then the expansion of the brain continued with the emergency of H. sapiens and previously with H. neanderthalensis, both with about 1500 mL (Miller et al. 2019; Ponce de León et al. 2021). Obviously, this enlargement of the brain and especially of the prefrontal cortex determined the increase in the capacities of reasoning, reflection, adaptation, socialization and other skills, that is, of the development of human intelligence.
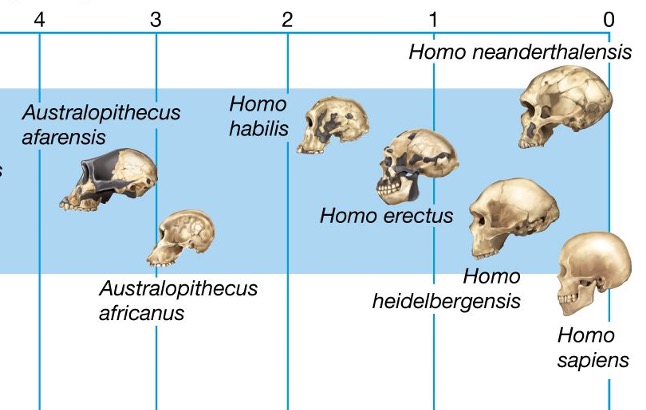
———————————————————–
MORE BRAIN AND LESS GUTS
There are several theories about the mechanisms that would have favoured this accelerated expansion of the brain. The limiting factor in enlargement is the availability of caloric resources because the brain has a high metabolic spending, compared to most other tissues. The metabolic rate of the brain at rest accounts for 22% of that of the human body (McClave & Snider 2001).
Mutations that led to an increase in brain size, although they would have clear final benefits, would not be adaptive if they had a greater risk of hunger. A reduction in the amount of intestinal tissue, which has metabolic needs like those of the brain, would release calories necessary for digestion to reassign them to the brain. This is confirmed by the fact that the size of the colon of humans is the fourth part of the corresponding to primates of our size (Table 1), while the brain of the current H. sapiens is almost the triple.
Table 1. Expected mass of some human organs based on the values of great apes compared to the real ones of a 65 kg western human (adapted from Bryant et al. 2023).
Organ | Expected mass (kg) | Real mass (kg) | Real / expected |
Heart | 0.32 | 0.30 | 0.94 |
Liver | 0.24 | 0.30 | 1.25 |
Small intestine | 0.40 | 0.62 | 1.55 |
Colon | 0.85 | 0.22 | 0.26 |
Brain | 0.45 | 1.30 | 2.89 |
CHANGES IN DIET
However, this intestinal reduction had to be accompanied by a change in the diet, with easier digesting foods and more energetic ones. The precursor hominids of Homo would have changed from an herbivorous-frugivorous regime to an omnivore-carnivorous one. Until now, the hypotheses point to the following two changes, well known and quite plausible:
1) The higher consumption of meat —of animal protein in general— has been argued as one of the key elements in human evolution. H. sapiens‘s diet is clearly more carnivorous than the other primates’ diet, and therefore the hunting of other animals should have been a growing habit in Homo precursors (Mann 2000). However, a weak point of this hypothesis is that hunting seems to be unimportant initially —circa 2 Ma—, since these first Homo and their precursors were mainly gatherers, and hunting was fully strongly developed later, at the end of the lower Paleolithic —about 500,000 years ago— in parallel with the development of the first prehistoric weapons (Bryant et al. 2023).
However, it seems that the consumption of carrion left by other carnivorous animals was prior to the hunt, from the end of the Pliocene or the beginning of the Pleistocene about 3 Ma. An alternative related to scavenging but more profitable for consuming meat is to take the prey hunted by other animals. In fact, archaeological records show that the latter option for fresh meat was predominant on passive carrion, where meat consumption performance is lower (Bunn & Ezzo 1993).
But the consumption of high nutritional animal protein is not limited to mammalian and bird meat: fishing must be considered and especially seafood harvesting. The latter case has the archaeological advantage of having found many places with mollusc shells accumulation, which indicates the great exploitation of this protein resource by humans since long ago. The oldest found shells are those of Pinnacle Point in South Africa 160,000 years ago, very important because together with other remains they are one of the pieces of evidence of the first Homo sapiens (Marean et al. 2007), but of course, they are certainly later than the brain enlargement.
2) The domestication of fire and the consequent possibility of cooking food was another crucial element to obtain more bioavailable caloric substrates and to digest them more easily, both at the mechanical chewing level and of energy expenditure on the digestive tract. This is very evident in the commented consumption of meat, both fresh and carrion, and in this case to mitigate microbial pollution. In addition, cooking was also very important in allowing the ingestion of vegetable foods and especially tubers, rich carbohydrate reserve organs, but they are not directly digestible and/or contain toxic compounds if they are not cooked (Wrangham et al. 1999).
However, there is no clear archaeological evidence that Australopithecus or the first Homo dominated the fire. The first evidence would be for H. erectus between 1 and 1.5 Ma (Hlubik et al. 2019) but more clearly for 800,000 years ago (Goren-Inbar et in 2004). Therefore, the full control of fire would have been achieved after the beginning of the brain development. In fact, fire expertise requires the cognitive ability to plan, create, maintain and use fire effectively, that is, a brain more developed than that of Australopithecus (Bryant et al. 2023).
———————————————————–
HYPOTHESIS OF EXTERNAL FOOD FERMENTATION, in contrast to the usual “INTERNAL”
The authors of the work (Bryant et al. 2023) propose this term of “external” to differentiate it from the internal fermentation that is made by the microbiota of the human gastrointestinal tract in digestion. The idea is that the outsourcing of this internal fermentation released body energy requirements that allowed cerebral expansion.
It should be remembered that the term “fermentation” is used here in its more general meaning transformation of organic compounds by microorganisms, while the original meaning of the concept of “fermentation” in a biochemical sense is strictly the type of metabolism anaerobic metabolism where energy and carbon source are organic compounds, and the electron acceptor are these same compounds. Most food fermentations such as dairy or alcoholic one belong to this biochemically speaking meaning, but other processes of microbial transformations that we include in addition to these when talking about “fermentation” in general, are other types of metabolism, such as aerobic degradation or other reactions. You can see more information on fermented foods in my post “Fermented Foods: consensus statement and reviewing them” (Figure 3).
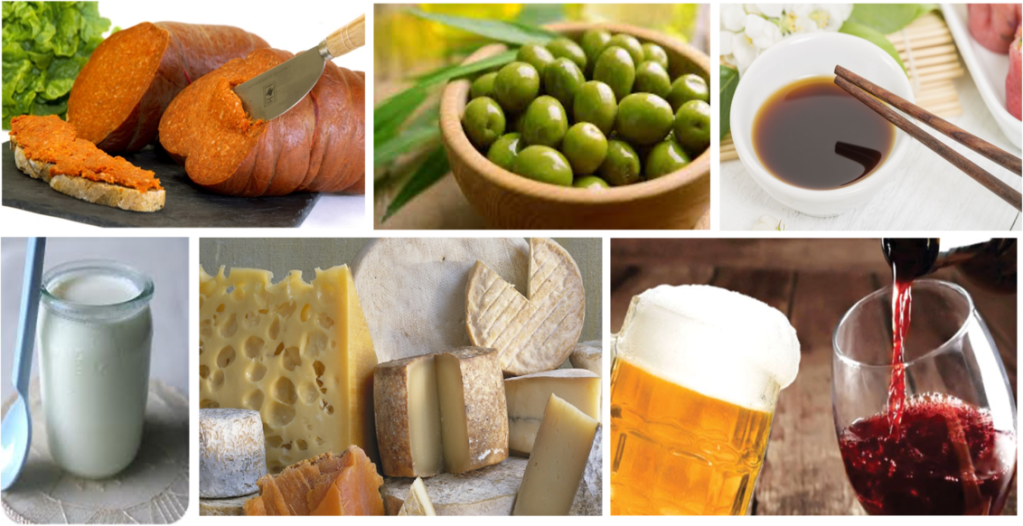
Although it is not usual to call it this way, the digestion that takes place in the human gastrointestinal tract or other animals includes this “internal fermentation“, understood as microbial intervention, that is, the set of transformations performed by microorganisms, the intestinal microbiota, especially in the colon. The digestion of a significant part of plant fibrous components requires this internal fermentation by the microbiota. In ruminants this is achieved with additional stomachs and an abundant cellulolytic microbiota. In the other non-ruminant animals, including primates, there is a more developed colon and cecum, and a higher area for the absorption of nutrients. The colon of humans and many primates contains about 10^12 microbes per mL and the transit time for this large gut is about 20-40 hours, while for the small intestine it is only 2-4 h (Bryant et al. 2023). The relevance of the microbiota of the colon for health is becoming clearer, in terms of nutrient absorption, energy regulation and a correct immune system (O’Hara & Shanahan 2006).
Soluble fibre, especially oligosaccharides, is fermented by the microbiota, producing short chain fatty acids (SCFA), which provide about 2 cal/g of fibre, which is an additional 50% to 4 cal/g available for direct digestion of easy carbohydrates (starch, sugars). These 2 cal/g are 2-10% of the total energy that the diet gives us, which is very small compared to other mammals (Table 2). These SCFAs are mainly acetate, propionate and butyrate. Acetate is used to synthesize cholesterol and other SCFAs, and provides energy to the heart, kidneys and muscle; propionate is a precursor to the liver synthesis of glucose and proteins; and butyrate is the favourite source of energy for colonocytes.
Table 2. Energy derived from short chain fatty acids (SCFA) produced by the fermentation of the gut microbiota.
Species | Diet | % Energy of total digested |
Cattle | Herbivorous ruminant | 72 |
Sheep | Herbivorous ruminant | 84 |
Rabbit | Herbivorous monogastric | 32 |
Beaver | Herbivorous monogastric | 19 |
Porcupine | Herbivorous monogastric | 16 |
Pig | Omnivorous | 36 |
Howler monkey | Herbivorous monogastric | 30 |
Gorilla | Herbivorous monogastric | 57 |
Homo sapiens | Omnivorous | 2-10 |
Apart from the SCFA, the main nutrients produced by the microbiota are vitamins B and K, which are absorbed by the gut. In addition, the microbiota increases the bioavailability of mineral micronutrients by degrading antinutritional factors such as phytates and oxalates —present to many vegetables—, which form complexes with cations (Fe, Zn, Mg, Ca, …) and prevent their absorption.
The external food fermentation that the first humans began to perform has similar functions to the internal one, such as increasing bioavailability and absorption of macronutrients and micronutrients. All this increases the digestibility of carbohydrates and proteins, for example in legumes hydrolysing macromolecules to amino acids and more digestible sugars. Carbohydrate fermentation also increases available vitamins B in an order of magnitude (Sandhu et al. 2017). And the phytates and oxalates can be degraded by the phytase produced by lactic acid bacteria present in external fermentations, increasing the absorption of minerals. This elimination of phytates is even more effective by fermenting than by cooking since the activity of phytase decreases above 80ºC when food is cooked.
A great benefit from external fermentation is that it can make toxic food cease to be. The best -known case is the detoxification of the cyanogenic glycosides of the cassava, a basic food of millions of people in tropical areas. If it is not fermented, these glycosides are hydrolysed by the colon microbes producing the toxic cyanide. When cassava is properly fermented by lactic acid bacteria, the cell walls of tubers break and allow toxin hydrolysis, also favoured by the lactic acid produced by these bacteria (Padmaja & Steinkraus 1995).
In addition, external food fermentation contributes to a better efficiency of the intestinal microbiota in digestion. First, part of the microbiota ingested with fermented food can colonize the intestine, contributing to microbiota biodiversity, increasing the ability to ferment more nutrients, and favouring that some endogenous microbes produce bacteriocins against possible pathogens. It has been seen that these benefits are also possible although fermented food microbes have only transitional contact with resident bacteria (Ohland & Macnaoughton 2010). With this, external fermentation can help the endogenous microbiota to protect human host from infections and diseases, because a correct microbiota producing an amount of SCFA from non-digestible carbohydrate fermentation is well related to a reduction in gastrointestinal disorders (Alexander et in 2019).
EXTERNAL FOOD FERMENTATION, DRIVING THE EXPANSION OF THE HUMAN BRAIN
As seen before, it seems that diet changes observed from Australopithecus to humans, such as the highest meat consumption or cooking food with fire, are relatively later than the expansion of the brain, and only with these changes it is difficult to explain the rapid development of the brain, simultaneous with the reduction of the colon and the displacement of high energy expenditure of the intestine to the brain.
For beginning of external food fermentation, it would not need to have a great capacity for reasoning. Australopithecus already had some simple tools that they could use to scorch animal or carrion, and could transport this food to home, being it a cave or a temporary shelter, thanks to the already developed bipedalism. They could also transport fruits, tubers and other potential foods. Although, for example, chimpanzees can occasionally carry temporary tools or the remains of hunted animals, they do it within short distances, about hundreds of meters at most, and most foods are consumed at the capture site.
Once at home, these first Homo probably left some food to consume it later, and surely accumulate more food than the captured. The reusing of storage site would have promoted a microbial ecosystem that would lead to fermentation. Food again incorporated would have been inoculated with those microbes present in the place, or in the body of the same hominids, for example. This socially transmitted practice to reuse sites, containers or tools for manipulating food would have been promoting the fermentations and stability of fermentative microbial agents. As in any selection process, this primitive technology would have been modified, especially learning not to consume the products damaged with toxic pathogens or compounds, probably with some human victim along the way.
This external food fermentation requires little knowledge, much less than the use of fire, since fermentation is a natural process that can pass spontaneously. It is a passive process for which there is no need for active efforts such as maintaining fire. In addition, fermentation can preserve food for a long time, even years, thanks to some fermentation products such as lactic acid or ethanol.
In fact, it is proposed that other species of Homo such as Neanderthals were already fermenting meat, in such a way that the decrease of pH by the acid produced preserved the vitamin C contained in the meat and thus avoided the scurvy (Speth 2019) .
Surely fermentation would be combined with other conservation techniques such as smoking, drying and salting, as it is currently done. The ease of fermentation in very different types of foods, environments and conditions must have been disseminated. The most obvious proof is that nowadays there are multiple fermented foods, in virtually every part of the world. It is estimated that there are more than 5,000 varieties of fermented foods, which are 35% of the current market of all foods, according to FAO. We see some of them in Table 3. We can also see a selection of 36 of them on a gastronomic information website: howtocook.recipes.
Table 3. List of fermented food, sorted by substrate types: vegetable or animal (modified and enlarged from Bryant et 2023).
Product name | Substrate vegetable: leaves, roots | Type of product | Place of origin | Microorganisms |
Kimchi | Cabbage leaves, radish, others | acid | Korea | Lactic acid bacteria (LAB) |
Sauerkraut | Cabbage leaves | acid | Europe | LAB, enterobacteria |
Pu-erh | Tea leaves | acid, beverage | Asia E | Moulds, yeasts |
Kombutxa | Tea leaves | acid, beverage | Asia E | Acetic acid bacteria, yeasts |
Dolma | Grape leaves | acid | Europe SE | LAB |
Gundruk | Leaves of radish, cabbage and others | acid | Nepal | LAB |
Sinki | Radish root | acid | Nepal | LAB |
Garri | Cassava root | acid | Africa W | LAB, moulds, yeasts |
Sapal | Taro tuber | acid | Papua Nova Guinea | LAB, yeasts |
Poi | Taro tuber | acid | Hawaii | LAB |
Tocosh | Potato tuber | acid | America S | LAB |
Fufu | Nyam and cassava roots | acid | Africa W | LAB |
Product name | Substrate vegetable: fruits, seeds | Type of product | Place of origin | Microorganisms |
Natto, Kinema and others | Soybean | alkali | Japan, Asia E | Bacillus subtilis (more info in my post) |
Gochujang | Pepper, rice, soy, cereals | acid + sweet spicy, condiment | Korea | Bacillus, Enterococcus, cyanobacterium Aerosakkonema, moulds |
Tempeh | Soybean | alkali | Indonesia | Rhizopus |
Soy sauce | Soybean | alkali + acid, condiment | Asia E | Aspergillus oryzae (koji), LAB, yeasts |
Miso | Soybean, cereals | alkali + acid, condiment | Japan | Aspergillus oryzae (koji), LAB, yeasts |
Oncom | By-products of soy, cassava and others | alkali | Indonesia | Rhizopus, Neurospora |
Sumbala, Dawadawa | Néré (Fabaceae) seeds | alkali | Africa W | Bacillus, LAB |
Coffee | Coffee seeds | acid, beverage | Africa E | Enterobacteria, Bacillus, LAB and yeasts |
Cacao | Cacao seeds | acid | America central and S | Yeasts, LAB and acetic acid bacteria |
Table olives | Fruits | acid | Mediterranean | LAB, yeasts |
Pickling | Cucumber, eggplant, radish | acid | Mediterranean | LAB, acetic acid bacteria |
Vinegar | Fruits, cereals | acid, condiment | Mediterranean | Acetic acid bacteria |
Sourdough | Cereal grains | acid, dough | Europe, Asia W, America N | LAB, yeasts |
Appam | Rice, coconut milk | acid, pancake | India | LAB, yeasts |
Idli | Rice and lentils | acid | India | LAB |
Kenkey | Maize grains | acid, dough | Africa W | LAB, yeasts |
Pozol | Maize grains, cacao | acid, beverage | America central | LAB, other bacteria, yeasts, moulds |
Injera | Cereal grains (Eragrostis tef) | acid, pa | Ethiopia, Africa E | LAB, Bacillus, enterobacteria, yeasts |
Product name | Substrate vegetable: fruits, seeds | Type of product (alcoholic) | Place of origin | Microorganisms |
Pulque | Sap of maguey (Agave) | alcohol, beverage | Mexico | Zymomonas, LAB, yeasts |
Wine | Grapes, fruit vine | alcohol, beverage | Mediterranean | Yeasts, and LAB in malolactic fermentation |
Cider | Apple | alcohol, beverage | Europe W | Yeasts |
Pear cider | Pear | alcohol, beverage | United Kingdom, France | Yeasts |
Fruit wine | Fruits: cherry, banana, others | alcohol, beverage | Europe N, America central | Yeasts |
Beer | Cereal grains | alcohol, beverage | Europe, Asia W | Yeasts |
Sour beer (more info in my post) | Cereal grains | alcohol + acid, beverage | Belgium, Germany | Yeasts, LAB |
Kvass | Cereal grains | alcohol + acid, beverage | Europe E | Yeasts, LAB |
Sake, rice wine | Rice grains | alcohol, beverage | Japan | Yeasts, Aspergillus oryzae (koji) |
Makgeolli, Korean rice wine | Cereal grains | alcohol, beverage | Korea | Yeasts, Aspergillus, LAB, proteobacteria |
Chicha | Maize grains | alcohol, beverage | America S | LAB, other bacteria, yeasts |
Product name | Substrate (animal product) | Type of product | Place of origin | Microorganisms |
Med, hydromel, Tej | Honey | alcohol, beverage | Africa, Asia, Europe | Yeasts |
Cheese | Milk | acid | Worldwide | LAB, other bacteria, yeasts, moulds |
Yogurt, other fermented milks | Milk | acid | Europe E, Asia W | LAB |
Crème fraiche | Milk | acid | France, Europe | LAB |
Kefir | Milk | acid | Caucasus | LAB, yeasts |
Kumis | Mare milk | acid, alcohol | Asia central, America S | LAB, yeasts |
Chal | Camel milk | acid | Asia central | LAB, yeasts |
Leben | Milk | acid | Africa N, Asia W | LAB |
Buttermilk | Butter | acid | Europe, Asia W | LAB |
Fermented sausages | Pork meat and others | acid | Europe | LAB, yeasts, moulds |
Ham | Pork meat | acid | Europe | LAB, other bacteria, moulds |
Nem chua | Pork meat, rice, banana leaves | sweet-and-sour | Vietnam | LAB |
Satchu | Meat | acid | Himalayas | LAB, other bacteria, yeasts, moulds |
Pemmican | Bison meat, deer, others | acid | America N | Various bacteria |
Dodery | Bones of animals | acid | Sudan | Bacillus, various bacteria, LAB yeasts |
Tiroi | Mussels, other seafood | acid | New Zealand | Various bacteria, LAB |
Kina | Sea urchin | alkali | New Zealand | Various bacteria |
Hákarl | Shark | alkali | Island | Proteobacteria: Moraxella, Acinetobacter |
Ngari | Fish pool barb | acid | India, Himalayas | BL, Bacillus, yeasts |
Surströmming | Herring | acid | Sweden, Europe N | Halanaerobium (firmicute), LAB, other bacteria |
Nam-pla, bagoong and others | Various fishes | acid, condiment | Asia SE, Philippines, Europe | Bacillus, other bacteria, archaea halophiles |
Garum | Fish entrails | acid, condiment | Old Greece, Rome, Byzantium | Various bacteria and archaea |
Fermented foods currently are an important part of the human diet everywhere, both in regions where food safety and conservation are not well controlled but also in more developed countries. It is a global technology among humans, which is a proof that it comes from early humans. As seen (Table 3), food substrates can be vegetable, from the different parts of plants, or from many different animals.
Also, although cultural practices of fermenting foods vary greatly globally, it seems clear that we humans generally like fermented foods. This preference would have emerged in parallel with an adaptive attraction to the aromas and textures of fermented foods by early humans. This is why we can see how many of these foods are condiments, that is, they are added to other foods in order to improve their palatability (Bryant et al 2023).
This great diversity of fermented foods means that some very strange tastes and aromas are highly appreciated by some cultures and detested by others, as is the case with some very smelly cheeses, with volatile ammonia and sulphur compounds. There is a cultural specificity in its consumption. The same aromas that may signal “good” food in one culture may signal bad or stale food in another. The ability to “taste” acidic, sour, or bitter foods —tastes unusual in natural foods and absent in other animals— must have evolved in humans with the production of fermented foods (Frank et al 2022).
As seen before (Tables 1 and 2), the development of external food fermentation was linked to a significant loss of colon mass and of the energy produced there, and therefore this implies a reduction in quantity and diversity of the intestinal microbiota because these are not so necessary. This is evidenced when doing comparative analyses of the human microbiota with that of other hominids such as chimpanzees, bonobos or gorillas (Moeller et al 2014).
On the other hand, the preference of humans for fermented foods is also demonstrated by genetic analyses. For example, some olfactory receptor genes related to fermented products are positively selected in humans and not in chimpanzees, such as methyl octanoate, a fruity odour produced by winemaking yeasts, or methyl valeric acid, a key aroma in ripened cheeses.
The ability to metabolize the ethanol produced in alcoholic fermentation and therefore be able to consume it in moderation is due to gene variants that code for alcohol dehydrogenase (ADH7), which would logically have been promoted in the first humans with the first drinks obtained by fermentation. However, it seems that this ability would predate humans, because other great primates have it, and even other mammals such as some Chiropters. All these are consumers of fruits, which can be fermented spontaneously in nature, and therefore all these animals would have acquired this ability by consuming fruits that have been partially fermented (Janiak et al 2020). So, hominids would already be adapted to metabolize ethanol long before the first humans did it in a more targeted way (Carrigan et al 2015).
———————————————————–
CONCLUSION
I believe that this hypothesis of external food fermentation as a key element in the brain expansion observed in the evolution from Australopithecus to humans is very plausible. Food fermentation in many cases is almost spontaneous, it initially requires very little technology and knowledge, and with a minimal selection of the resulting products after fermentation, more digestible foods are obtained, which are better preserved, and which have tastes or new and interesting textures.
This development of fermented foods allowed not being necessary to have a considerable volume of colon with its diverse microbiota, to acquire nutrients that can be consumed by preparing them beforehand. By reducing the caloric needs of the colon, the “leftover” energy could be devoted more and more to the brain, facilitating its expansion. Logically and in parallel, or in some cases later, the other factors discussed such as the consumption of meat, new hunting technologies, socialization, and fire, allowed even more this enlargement of the brain, until reaching Homo sapiens.
Finally, I just want to comment that I particularly have liked this work because it puts together three of the topics that most attract me scientifically:
1) Fermented foods, or as Bryant et al’s article calls it, “external fermentation.” In fact, this external designation surprised me, since I had never thought of calling “internal fermentation” the set of processes of modification or degradation, or synthesis of compounds carried out by the intestinal microbiota. But ok, it’s true. In any case, fermented foods and aspects of the benefits of microorganisms (“the good microbes”) have always been my primary topic of research work, and teaching, and my interest since I finished my bachelor’s degree in biology 50 years ago.
2) Intestinal microbiota. It has been a topic that has interested me for several years. Although I haven’t worked on it directly at a research level, I have been getting to know it, and I touch at a teaching level. As we have seen in recent years, the role of the gut microbiota in the healthy maintenance of the body is much more important than we thought, although there is still much to be learned. Curiously and somewhat disappointed, I have discovered with this work, that humans have reduced the gut microbiota compared to other primates, precisely with the development of this “external fermentation”.
3) Origin and human evolution. Of course, this topic is of great interest to me, as I suppose to everyone. With a certain knowledge of living beings and admiring how all biological evolution works, knowing more about how our species appeared and those close to us, is exciting.
———————————————————–
BIBLIOGRAPHY
Alexander C, Swanson KS, Fahey GC, Garleb KA (2019) Perspective: physiologic importance of short-chain fatty acids from nondigestible carbohydrate fermentation. Adv Nutr 10, 576–589
Amato KR, Chaves OM, Mallott EK et al (2021) Fermented food consumption in wild nonhuman primates and its ecological drivers. Am J Phys Anthropol 175, 513–530
Bryant KL, Hansen C, Hecht EE (2023) Fermentation technology as a driver of human brain expansion. Commun Biol 6, 1190
Bunn HT, Ezzo J (1993) Hunting and Scavenging by Plio-Pleistocene Hominids: Nutritional Constraints, Archaeological Patterns, and Behavioural Implications. J Archaeol Sci 20, 365-398
Carrigan MA, Uryasev O, Frye CB et al (2015) Hominids adapted to metabolize ethanol long before human-directed fermentation. Proc Natl Acad Sci USA 112, 458–463
Cordain L, Eaton S, Miller J et al. (2002) The paradoxical nature of hunter-gatherer diets: meat-based, yet non-atherogenic. Eur J Clin Nutr 56, S42–S52
Dyer R (2023) Food preserving technique may have sparked human brain growth, scientists say. Science Alert – Humans, 3/12/2023
Frank HER, Amato K, Trautwein M et al. (2022) The evolution of sour taste. Proc. Biol. Sci. 289, 20211918
Goren-Inbar N, Alperson N, Kislev ME et al. (2004) Evidence of Hominin Control of Fire at Gesher Benot Ya`aqov, Israel. Science 304,725-727
Hlubik S, Cutts R, Braun DR et al (2019) Hominin fire use in the Okote member at Koobi Fora, Kenya: New evidence for the old debate. J Human Evol 133, 214-229
Janiak MC, Pinto SL, Duytschaever G et al (2020) Genetic evidence of widespread variation in ethanol metabolism among mammals: revisiting the ‘myth’ of natural intoxication. Biol. Lett. 16, 20200070
Mann N (2000) Dietary lean red meat and human evolution. Eur J Nutr 39, 71–79 (2000)
Marean C, Bar-Matthews M, Bernatchez J. et al (2007) Early human use of marine resources and pigment in South Africa during the Middle Pleistocene. Nature 449, 905–908
McClave SA, Snider HL (2001) Dissecting the energy needs of the body. Curr Opin Clin Nutr Metab Care 4(2):143-7
Miller IF, Barton RF, Nunn CL (2019) Quantitative uniqueness of human brain evolution revealed through phylogenetic comparative analysis. eLife 8:e41250
Moeller AH, Li Y, Ngole EM et al (2014) Rapid changes in the gut microbiome during human evolution. Proc Natl Acad Sci USA 111, 16431–16435
O’Hara AM, Shanahan F (2006) The gut flora as a forgotten organ. EMBO Rep 7, 688–693
Padmaja G, Steinkraus KH (1995) Cyanide detoxification in cassava for food and feed uses. Crit Rev Food Sci Nutr 35, 299–339
Ponce de León MS, Bienvenu T, Marom A et al. (2021) The primitive brain of early Homo. Science 372, 165-171
Ohland CL, Macnaughton WK (2010) Probiotic bacteria and intestinal epithelial barrier function. Am J Physiol Gastrointest Liver Physiol 298, G807–19
Sandhu KS, Punia S, Kaur M (2017) Fermentation of cereals: a tool to enhance bioactive compounds. Plant biotechnology: Recent advancements and developments 157, 157–170
Speth JD (2019) Neanderthals, vitamin C, and scurvy. Quat. Int. 500, 172–184
Wrangham RW, Jones JH, Laden G et al. (1999) The Raw and the Stolen: Cooking and the Ecology of Human Origins. Curr Anthrop 40:5, 567-594
Alternative meat: eating microbial protein and others
18th December 2022
Translated from the original article in Catalan (10th December 2022)
PROBLEMS OF ANIMAL PROTEIN PRODUCTION
Most of the protein that we humans consume is meat protein of animal origin produced by industrial livestock farming, be it birds or mammals, and of these mainly ruminants such as beef, whose global production has doubled in the last 50 years. Industrial farm systems, both intensive and extensive, have very negative environmental consequences. As we see schematically in Figure 1, the main disadvantages are:
The area of land occupied by cattle breeding is increasing. Counting the pastureland and vegetable crops to feed the animals —cereals and soybeans in particular—, almost 30% of the land surface is reached, much more than that devoted to crops for human consumption, 13%. In the case of soy, for example, 3/4 of its production is dedicated to feed, and an important part comes from regions of Brazil that were previously jungle or savannah (Hooper 2022).
The fresh water used for the manufacture of any product is called the water footprint (Hidrofilic 2017). In the case of livestock, which includes the cultivation of vegetable feed, water footprint is much greater than any other agricultural crop: 15000 L of water are needed for each kg of beef, 9000 L per kg of sheep meat, 6000 L per kg of pork, while only 300 L is needed for kg of vegetables or 900 L per kg of fruits or about 4000 L per kg of legumes, even less than for animals (Mekonnen & Hoekstra 2010). As we can see (Figure 1), it is estimated that in the USA 30% of the total water footprint is due to industrial livestock.
A third of global greenhouse gas (GHG) emissions are caused by the food system, and within this livestock farming is the main contributor, especially due to the methane gas expelled by ruminants, a result of the metabolism of methanogenic archaea, the final step of rumen fermentation. As a GHG, methane emissions from cattle cause the most environmental impact by far, being about 100 kg of methane for every 100 g of protein produced (Poore & Nemecek 2018).
Finally, it is necessary to take into account the repercussions of land degradation and erosion caused by industrial livestock farming, as well as soil acidification, contamination by antibiotics and eutrophication due to the excessive use of fertilizers (Humpenöder et al. 2022).
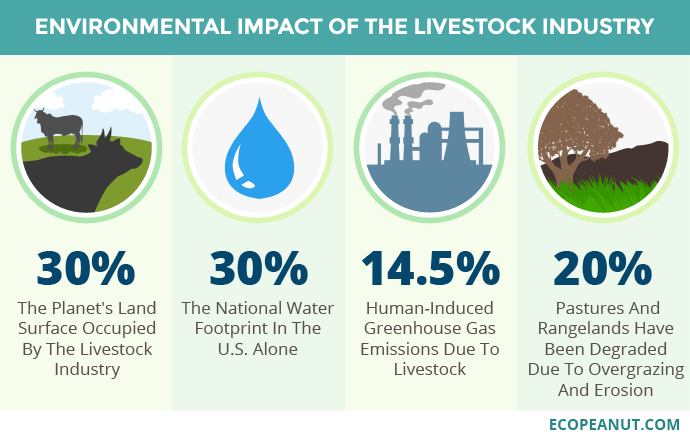
Figure 1. Environmental impact of the livestock industry. Image taken from Ecopeanut.com.
It is estimated that by 2050 the world’s human population will be almost 10,000 million (now in 2022 we are 7,800 million), for which we will need about 400 million tons of meat and 800 of dairy products per year, an amount that cannot be achieved by the low efficiency of vegetable protein in feed to animal protein, which is 6 kg of vegetable for 1 kg of animal (Ritala 2017).
All in all, some very concerned environmental activists like George Monbiot believe that agricultural and livestock exploitations lead to destruction, exploitation and economic senselessness that are killing the planet. However, he also believes that there is hope for a more sustainable and healthier world that would go through a consumption of microbes instead of animals (Hooper 2022).
Therefore, this medium-term situation is not environmentally sustainable, and alternatives must be found to replace much of the animal protein with other sources, such as microbial and others.
———
THE VEGETABLE ALTERNATIVE
The vegetable protein alternative is well known and forms part of our usual diet (Figure 2). Taking it to the extreme, vegetarians base their diet only on vegetables and exclude meat foods. In India they are 1/3 of the population, but in the rest of the world vegetarians are a minority, 5% in Europe. The reasons for vegetarians – and vegans, who are more strict – are very diverse, such as ethical (animal sensitivity), health, religious, political, fashion, aesthetic, economic, but also increasingly there are the reasons of environmental and sustainability awareness.
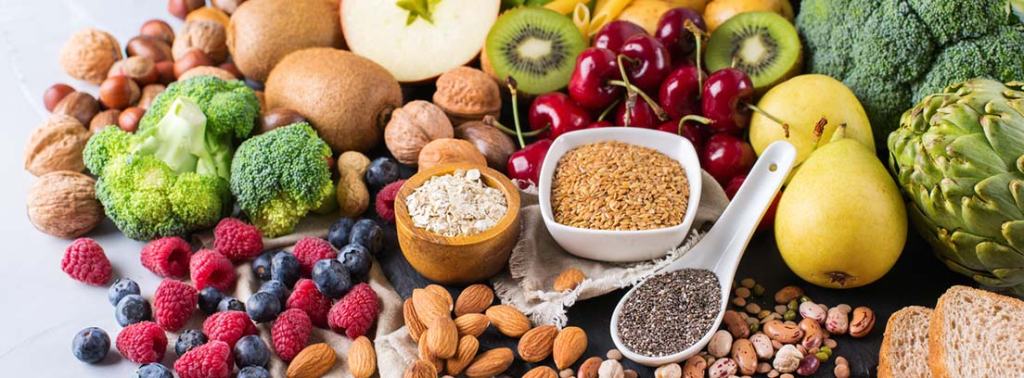
Figure 2. Vegetarian diet. Image taken from Salud Blogs Mapfre.
Vegetable protein sources are nutritionally valuable, since they contain fibre —almost non-existent in meat — and antioxidants, but their protein content is always lower than that of meat, which is 45% of the dry extract The vegetable foods with the most protein are soy (35% of dry extract) and legumes such as peas, chickpeas or beans (20-25%). In contrast, wheat, rice and other cereals or potatoes only contain 10% protein. Milk contains 25% and eggs 40% of the dry extract.
The main disadvantage of vegetable protein compared to animal, in addition to the lower total protein content, is the lower nutritional quality in terms of essential amino acids and lower digestibility, with which it is necessary to increase 10-20% of protein if only plant foods are consumed (Petrusán et al 2016). Another disadvantage is the environmental one, partly like the animal protein, due to the needs of large areas of land and a lot of water (Ritala et al. 2017).
———-
EATING INSECTS ?
Another alternative is the consumption of insect protein, or entomophagy. The protein content of the dry extract of insects is very high, between 35% for termites and 60% for crickets and locusts, and most edible insects have high contents of essential amino acids, and of fibre, minerals and vitamins. Insect “farms” are very suitable for sustainability and the environment, as they require no land, require little water, emit very few GHGs, and are economically advantageous. Therefore, they have very good potential as quality food (Lange & Nakamura 2021). However, consumer acceptance is a major hurdle, especially in Western countries. Instead, they are common in sub-Saharan Africa, Southeast Asia, Australia, and some Ibero-American countries such as Mexico, where “escamoles” are larvae of the Liometopum apiculatum ant very popular since pre-Hispanic times (Figure 3).
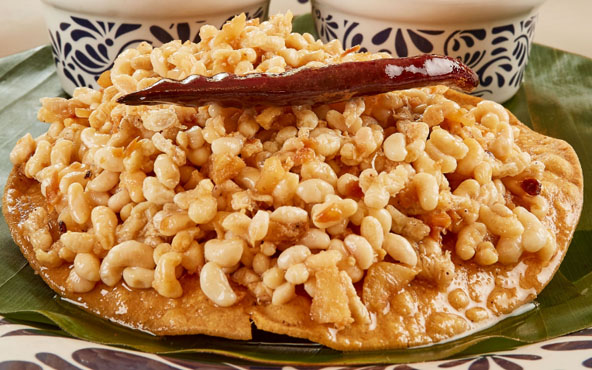
Figure 3. Dish of Mexican escamoles. Image taken from Lideresmexicanos.com.
When insects are produced industrially, it is necessary to consider and control possible sources of food safety risks, such as allergens, pathogenic microorganisms that can be transmitted via insects, or mycotoxins from fungi that contaminate insects (Lange & Nakamura 2021).
Currently, most investments in the production of edible insects are for animal feed. It is being produced as a protein powder for domestic animals, in aquaculture and is starting to be introduced as a supplement to livestock feed. In addition, the droppings of insects in the productive phase can be used as fertilizer (Godwin 2021).
————–
CULTURED OR SYNTHETIC MEAT
Also called “in vitro” meat or “clean” meat or “laboratory meat”, it consists of growing animal muscle tissue in laboratory cultures from stem cells. With this method, which is faster and more efficient than obtaining traditional meat, there is no need to sacrifice animals, nor are there all the mentioned drawbacks of livestock farming.
To obtain cultured meat, a series of requirements summarized in these steps are needed that must work well both from a biological and commercial point of view:
1. Obtain skeletal muscle samples from the appropriate animal
2. Separate the stem cells from the other muscle components and sometimes other cell types
3. Inducing the growth and proliferation of myoblasts in the appropriate physico-chemical conditions and medium, with growth factors
4. Inducing myoblasts to form multinucleated myotubes, in a framework or scaffolding structure, such as collagen
5. Achieve the continued growth of new myoblasts and the differentiation of myotubes into muscle fibres
6. Ensure continued growth throughout scale-up, introducing other components such as adipocytes, with lipids providing palatability
7. Process the resulting product by adding fats, flavourings and other compounds, and shape its physical appearance, all in order to mimic conventional meat products, which is easier with processed products such as minced meat or bacon (Figure 4) (Kadim et al. 2015).
Cultured meat can be just as good and nutritious as conventional meat from farm animals, in addition to the advantages of a drastically lower effect on the environment and on the animal in question. In addition, the yield is clearly greater, since with just one sample of tissue the same amount of meat can be produced as with 80 cows (Bingham 2020).
Although preliminary results so far are very promising, this technology is not yet developed enough for large-scale production, especially in terms of culture media, and consumer acceptance and trust is still very low (Kadim et al. 2015).
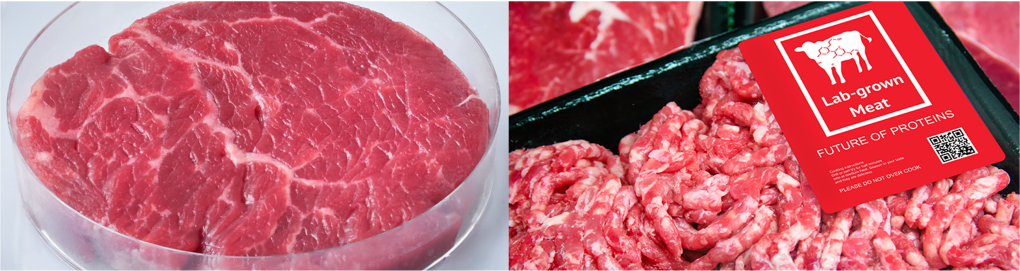
Figure 4. “Culturized” meats, made from animal cell cultures, simulating beef fillet (left) or processed meat (right). Images taken from Bingham (2020).
In a similar way, the production of fish fillets and seafood meat with cell cultures is also being developed, this is what is called cell aquaculture. The progressive replacement of caught fish by “farmed fish” would significantly reduce overfishing, eliminate illegal fishing and destruction of marine ecosystems, and these foods would not have potential pathogens or common contaminants such as methylmercury or particulate microplastics.
These farmed fish and shellfish products also have the organoleptic qualities of wild or farmed marine products but with the advantage of being more sustainable, safer, and healthier. Some of the companies that are developing them use techniques similar to organoid cultures or mini organs, which have been used for about 10 years for the study and treatment of diseases and tumours. Cultures are made in three-dimensional structures allowing the cells to form a natural composition of fat and muscle equivalent to that of the animal (VelSid 2022).
——————-
MICROBIAL PROTEIN
It is also known as Single Cell Protein (SCP) because many were originally single-cell microbes such as most bacteria —including cyanobacteria—, yeasts and some single-celled microalgae, but some filamentous fungi and some multicellular algae are also included. Logically, the microbes that have been studied the most in this regard are the ones that contain the most protein. We have a good review of the types, the process of obtaining and applications in the work of Junaid et al. (2020).
We see in Table 1 a summary of the main products that have been developed, most of them since the 1970s, when, with the first energy and environmental crises, alternatives to the production of meat protein were sought, although most of these products have not had great industrial and/or commercial development.
Table 1. Single Cell Protein products, all with at least 45% protein of dry extract. Adapted from Ritala et al. (2017).
Microorganism | Type | Use | Substrates | Companies (country) | Problems |
Methylophilus,Methylococcus | Methylotroph and methanotroph bacteria, proteobacteria | Animal feed | Methanol, methane | ICI (UK) “Pruteen” 1970s, Calista Inc. (UK) “FeedKind” from biogas | High content of RNA and DNA (>10%), needs processing |
Azonexus, Comamonadaceae | H2-oxidizing bacteria, proteobacteria | In perspective, food or feed | H2, CO2, O2, N2 | Startups: Air Protein (USA), Solar Foods (FI), Deep Branch (UK) | Still in R&D |
Arthrospira maxima, A. platensis | Previously “Spirulina”, bacteria: Cyanobacteria (“microalgae”) | Dietary supplement, food of Aztecs and towns of Chad | CO2, light | BlueBio Tech (D), Cyanotech (Hawaii USA), FEBICO (Taiwan), Parry Nutraceuticals (India) | Possible contamination with toxins (microcystins) from other cyanobacteria, and by heavy metals |
Aphanizomenon flos-aquae(AFA) | Bacteria: Cyanobacteria | Dietary supplement, positive effects on health | CO2, light | Blue Green Foods (USA), E3Live (USA), Klamath Valley (USA) | Some toxic strains |
Chlorella | Green microalgae: Chlorophyta | Dietary supplement | CO2, light | TerraVia (USA), Roquette Klötze (D), FEBICO (Taiwan), BlueBioTech (D) | Expensive production: carbonated water. Indigestible cell wall |
Saccharomyces cerevisiae | Single-celled fungi (yeasts), ascomycetes | Yeast extract: dietary supplement | Molasses, cereals hydrolysate | Bega Cheese (AUS), Flint Hills Resources (USA) | Occasional: tyramine migraines, irregular digestions, intolerance in patients with irritable bowel * |
Torula utilis | Cyberlindnera jadinii (sin. Candida utilis), yeasts, ascomycetes | Flavouring supplement, alternative to glutamate | Methanol, molasses | Phillips Petroleum Co (USA) until 2002, Provesteen Process (USA) until 1990 | Low profitability of the process |
Fusarium venenatum | Filamentous fungi, ascomycetes | “Mycoprotein”, cell wall rich in glucans (fibre), positive health effects | Glucose from starch, salts, inorganic N | Marlow Foods Ltd “QuornTM” (UK), Atlastfood (USA), Nature’s Fynd (USA) and others, in operation | Occasional appearance of mutants with highly branched mycelium, which make it necessary to stop the continuous culture every 6 weeks. |
Paecilomyces varioti | Filamentous fungi, ascomycetes | Animal feed | Sugars from lignocellulosic waste | Finnish paper factories, “Pekilo”, 1970-1990 | It wasn’t profitable, now reviving: https://www.eniferbio.fi/product/ |
As we can see, there are a few products that are microalgae or cyanobacteria, all of them photosynthetic microorganisms, which are interesting for their low production cost as they only need light and a little CO2 and some salts. Of these, we should highlight those known as Spirulina, which are cyanobacteria and have been a food source since the time of the Aztecs and other peoples of Central America, as well as the peoples around Chad. Today it is used as a human food supplement, in the form of tablets or powders (Figure 5) and is also used as a food supplement in the aquaculture and poultry industries. One of the main benefits is the high content of vitamin B12. However, it is necessary to monitor the product well as there may occasionally be contamination with cyanotoxins or the presence of pesticides and other toxic compounds, especially if it is consumed regularly (Grosshagauer et al., 2020).
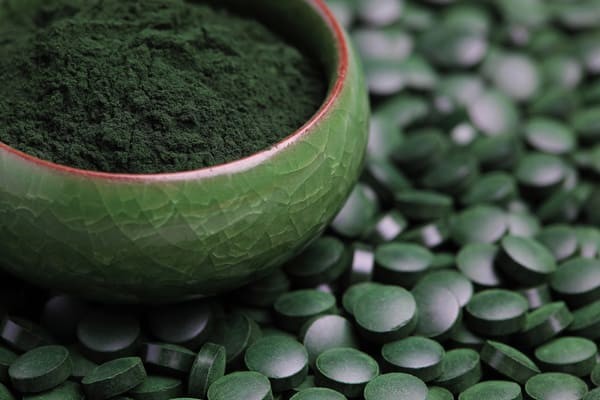
Figure 5. Supplements in powders and tablets based on “Spirulina”, cyanobacteria. Image taken from Iswari.com.
Many different bacteria, apart from cyanobacteria, have been assayed, such as some Bacillus, Corynebacterium and Rhodopseudomonas, but at an industrial level those that have been most successful as SCP are the methylotrophs and/or methanotrophs (Table 1), which contain a lot of protein (50-80% of the dry weight), are rich in essential amino acids such as methionine, and with relevant amounts of lipids and vitamins. Already in the 1970s, ICI (UK) developed the product “Pruteen” with Methylophilus methylotrophus from methanol, and currently Calysta Inc. (“FeedKind” product) and other companies are producing Methylococcus and other methanotrophs by converting methane, surplus on farms, into bacterial protein (Ritala et al. 2017).
The main problem with bacteria – and fungi – is their high content of nucleic acids, especially RNA, due to their rapid growth and protein synthesis, which requires high rates of transcription and translation. This is not the case with photosynthetics (microalgae and cyanobacteria) which grow more slowly. Ingestion of RNA-derived purines increases plasma uric acid concentration, which can lead to gout and kidney stones. Therefore, it is necessary to partially remove these nucleic acids during the SCP preparation process. The most common method is the combination of mild heat treatment with the use of ribonucleases (Ritala et al. 2017).
A promising case of bacteria are hydrogen-oxidizing chemolithotrophs that are also nitrogen (N2) fixers, such as Azonexus and the Comamonadaceae. They are known as “air-munching microbes” (De Sousa 2021) because they can grow only with N2, O2, CO2 and H2 because they oxidize hydrogen with O2 and fix atmospheric N2 (Figure 6). But since H2is almost non-existent in the atmosphere, it is necessary to provide it, obtaining it by hydrolysis of water with green energies. The main advantage of being N2 fixers is the saving of ammonium, the production of which requires a lot of energy, apart from the yield in protein (Hu et al., 2020).
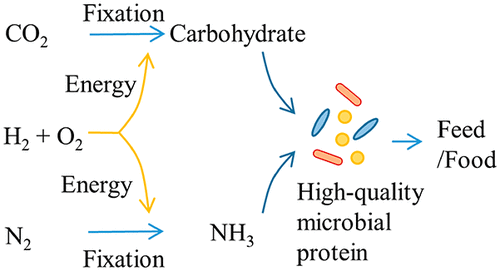
Figure 6. Scheme of the metabolism of nitrogen-fixing hydrogen-oxidizing bacteria. Taken from Hu et al. (2020).
Fungi and yeasts dominate the global SCP market for human consumption, as yeasts in particular have a long history of acceptance, especially in the form of extracts. Logically, most yeasts marketed as SCP are Saccharomyces cerevisiae, but there are also Torula utilis, Candida and Kluyveromyces. Yeasts and other fungi also have the drawback of nucleic acid content (10%), lower than bacteria, but which also requires processing to reduce them.
The mycelial fungus most used as SCP is undoubtedly Fusarium venenatum, mostly marketed under the name QuornTM by Marlow Foods Ltd. since 1985. In fact, it is the only SCP product used exclusively for human consumption. Like other fungi, apart from the high content of protein (mycoprotein), it is a good source of essential amino acids, vitamins and especially glucans, which contribute to the contribution of fibre to the diet. It is an ascomycete, considered a microfungus (Figure 7) due to the absence of macroscopic fruiting bodies, as are also Penicillium, Aspergillus and many other non-mushroom filamentous fungi.
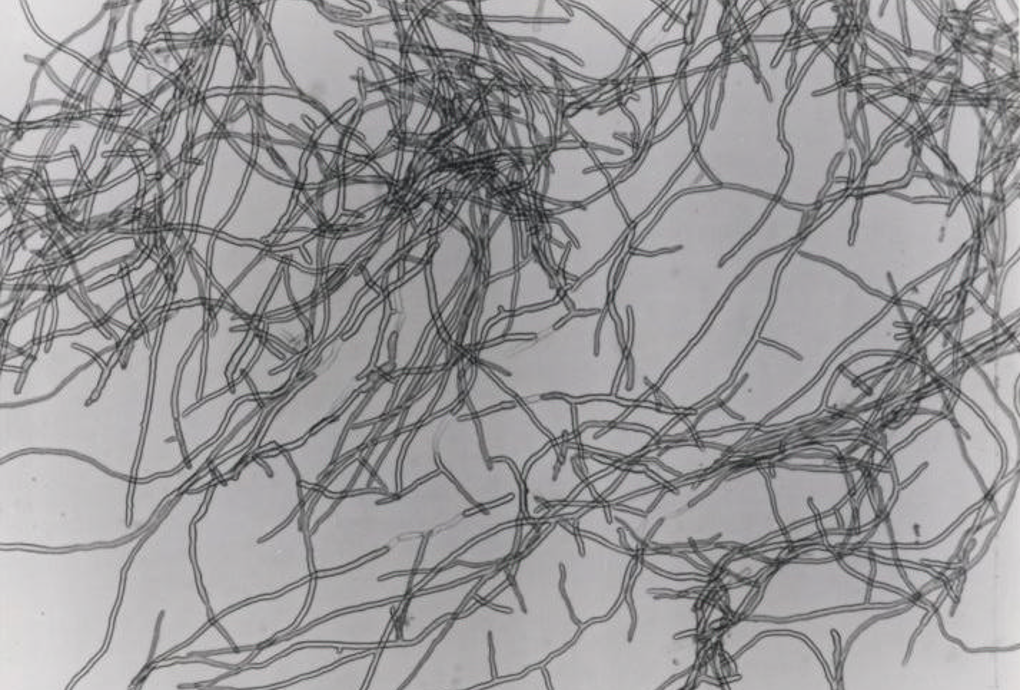
Figure 7. Electron micrograph (350 x) of the mycelium of Fusarium venenatum on the surface of Quorn product. Image taken from Ugalde & Castrillo (2002).
The production of F. venenatum is carried out in continuous bioreactors with aeration in an aqueous medium with glucose obtained by starch hydrolysis, a source of N, vitamins and minerals. The resulting mycelium is extracted (Figure 8) and treated to remove RNA, and dried.
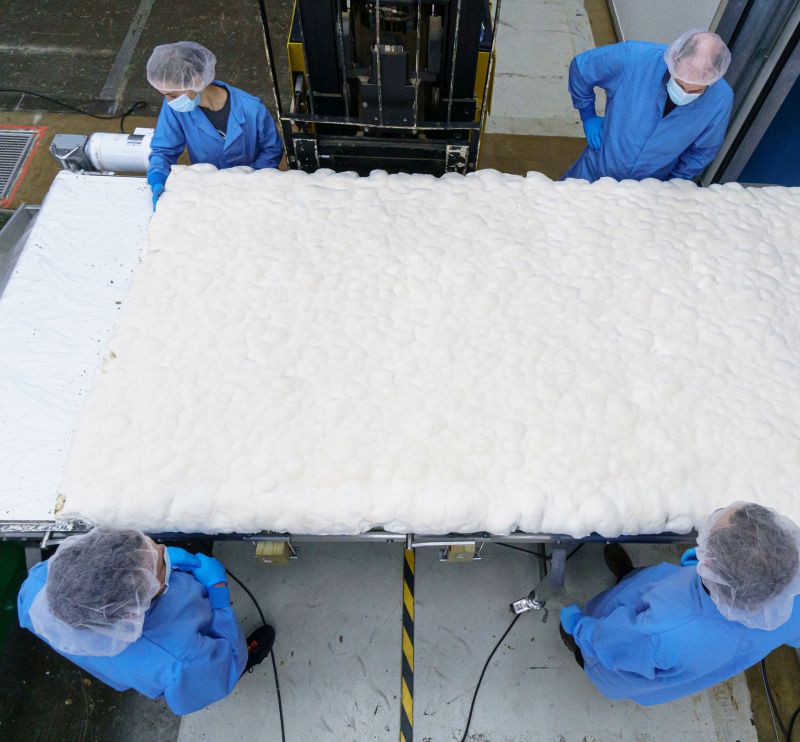
Figure 8. Preparing a Fusarium mycelium layer. Photo by MyForest Foods Co, taken from Dietrich (2022).
Products with mycoprotein such as Quorn in particular are intended mainly as a substitute for meat and are sold mainly in the form of prepared dishes, with an enormous variety of tastes and textures (Figure 9), logically adding additives. As a curiosity, see Quorn’s advertising website (www.quorn.co.uk), full of recipes and suggestions. Most products contain egg albumin in addition to the fungus, which acts as a binder. Vegan formulations replace the egg with potato.
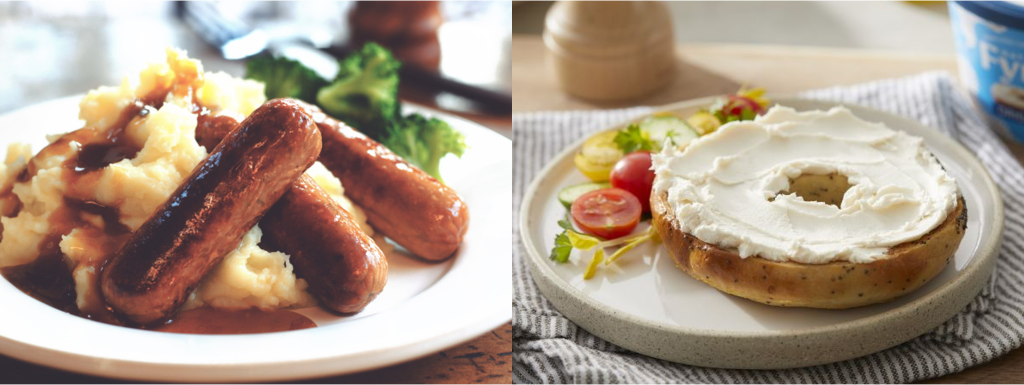
Figure 9. Examples of products made with F. venenatum: Quorn pseudosausages and Nature’s Fynd non-dairy cream cheese. Images courtesy of quorn.co.uk and Dietrich 2022.
Interest in SCP is growing, and related research and development is increasing greatly, especially in China, where 70% of the world’s SCP patents since 2001 have been registered, often related to the exploitation of agricultural waste such as methane (Ritala et al. 2017).
In any case, microbial protein must be considered as one more of the elements to take into account, in addition to the others mentioned, in the necessary transformation of the agri-food system, combining it with the reduction of food waste, the incentive to eat more healthy, and the marketing of products with less environmental impact than the current ones (Carrington 2022).
—————–
BIBLIOGRAPHY
Berkheiser K (2019) Four potential side effects of nutritional yeast. Nutrition, Healthline, 8 aug 2019.
Carrington D (2022) Swapping 20% of beef for microbial protein “could halve deforestation” The Guardian News website, Food, 4 May 2022.
Bingham L (2020) Cultured meat: better than the real thing ? Foodunfolded, 10 march 2020.
De Sousa A (2021) How air-munching microbes could grow the fake meat of the future. Bloomberg Europe edition website, 11 October 2021.
Dietrich T (2022) Microbes and mushrooms -the future of Earth-friendly food. National Science Foundation website, 20 January 2022.
Godwin R (2021) If we want to save the planet, the future of food is insects. The Guardian News website, The Observer Food, 8 May 2021.
Grosshagauer S, Kraemer K, Somoza V (2020) The true value of Spirulina. J Agric Food Chem 68:4109.
Hidrofílic (2017) Petjada hídrica. Aigües.net, 13/01/2017.
Hooper R (2022) Farming is the most destructive human activity ever (Interview with George Monbiot). New Scientist website, 19 May 2022.
Hu X, Kerckhof FM, Ghesquière J, Bernaerts K, Boeckx P, Clauwaert P, Boon N (2020) Microbial Protein out of Thin Air: Fixation of Nitrogen Gas by an Autotrophic Hydrogen-Oxidizing Bacterial Enrichment. Environ Sci Tech 54:3609
Humpenöder, F., Bodirsky, B.L., Weindl, I. et al. (2022) Projected environmental benefits of replacing beef with microbial protein. Nature 605, 90–96.
Junaid F, Khawaja LA, Sikander A (2020) Single cell protein as a potential meat substitute: a critical review. World J Pharmac Res 9:141.
Kadim IT, Mahgoub O, Baqir S, Faye B, Purchas R (2015) Cultured meat from muscle stem cells: a review of challenges and prospects. J Integr Agric 14, 222–233.
Lange KW, Nakamura Y (2021) Edible insects as future food: chances and challenges. J Future Foods, 1, 38-46
Mekonnen MM, Hoekstra AY (2010) The green blue and grey water footprint of farm animals and animal products. Value of Water Research Report Series n. 48, UNESCO-IHE
Petrusán JI, Rawel H, Huschek G (2016) Protein-rich vegetal sources and trends in human nutrition: A review. Curr Topics Pept Prot Res 17, 1-19
Poore J, Nemecek T (2018) Reducing food’s environmental impacts through producers and consumers. Science 360, 987–992
Quorn: https://www.quorn.co.uk
Ritala A, Häkkinen ST, Toivari M, Wiebe MG (2017) Single cell protein —State-of-the-Art, Industrial landscape and Patents 2001-2016. Front Microbiol 8, 2009
Ugalde UO, Castrillo JL (2002) Single cell proteins from fungi and yeasts. Appl Mycol Biotech 2:123
Velsid (2022) Producción de filetes de pescado a partir de la técnica para cultivar organoides. Gastronomía y Cía, República. 8 set 2022
Lactic acid bacteria of beers: the bad guys and the good ones
28th October 2018
It is not easy to “live” in the beer
In principle, lactic acid bacteria (LAB) and many other bacteria and generally most microorganisms, do not have it easy to survive in beer or other alcoholic beverages such as wine. This is one of the main reasons why wines and beers have been from ancient times the safest ways to drink hygienically something similar to water and that it was not contaminated, apart from boiled waters, such as tea and other herbal infusions.
The reasons for the difficult survival of microorganisms in beer are ethanol, the pH quite acidic (around 4), the lack of nutrients due to the fact that the yeasts have assimilated them, the little dissolved oxygen, the high concentration of carbon dioxide (0.5% by weight / volume) and the presence of humulone derived compounds (Figure 1) of hops: iso-alpha-acids, up to 50 ppm, which are microbiocides. All these obstacles make it very difficult for any microorganism to thrive. The most susceptible beers of unwanted microbial growth are those where some of the mentioned obstacles are dampened: beers with a higher pH of 4.5, or with little ethanol or little CO2, or with added sugars – which are nutrients -, or with little amount of compounds derived from hops (Vriesekoop et al 2012).
Figure 1. Humulone (left) of the hop is degraded during beer elaboration to isohumulone (right) and other iso-alpha-acids, which are compounds bitter and microbiocides (Wikipedia; Sakamoto & Konings 2003)
The acid pH of the beer (slightly higher than the wine) inhibits many of the best-known pathogens (Figure 2). And the cases we see that could grow at this pH near 4 are inhibited by other factors such as ethanol.
Figure 2. Range of acid pH for the growth of various bacteria, compared to the typical beer pH (Menz et al 2009).
The “bad” lactic acid bacteria of beer
Despite what we have just seen, some bacteria, particularly some LAB, have been able to adapt evolutionarily to the strict beer conditions, and they can survive and spoil them. In particular, the most frequent harmful species against the quality of beers are Lactobacillus brevis and Pediococcus damnosus (Figure 3). The first is the most frequent, and it can give tastes and undesired aromas, as well as turbidity to the final product. P. damnosus has the advantage of growing at low temperatures, and it can also produce undesired aromas, such as diacetyl (Vriesekoop et al 2012). Some Pediococcus and Lactobacillus may adhere to yeast, inducing them to sediment, which delays fermentation (Suzuki 2011).
Figure 3. Lactobacillus brevis (left) and Pediococcus damnosus (right) at the electronic scanning microscope.
Some Pediococcus may also be responsible for the appearance of biological amines in some beers, at risk for the consumer. Amines in a certain concentration are toxic, they may be present in some fermented foods such as cheese, cold meat and alcoholic beverages such as wines and beers, and are produced by decarboxylation of amino acids by LAB. The level of tyramine and other amines has been used as a measure of quality in some Belgian beers made with LAB (Loret et al 2005).
Apart from these LAB, other bacteria related to problems of beer contamination are acetic acid bacteria such as Acetobacter, typically associated with oxygen intake in packaging or distribution. Other harmful bacteria are some enterobacteria, such as Shimwellia pseudoproteus or Citrobacter freundii, which proliferate in the early stages of fermentation, and produce butanediol, acetaldehyde and other unwanted aromatic compounds (Vriesekoop et al 2012). Other harmful bacteria for beer, especially when bottled, are Pectinatus and Megasphaera, which are strict anaerobes, of the clostridial family, and can produce hydrogen sulphide and short chain fatty acids, all of them unpleasant (Suzuki 2011 ).
The “good” lactic acid bacteria of beer
LAB are well known for being some of the microbes that most benefits contribute to the food production, on the one hand as an economic means of preserving food, and on the other hand to improve their quality and organoleptic characteristics. That’s why they are the main agents of fermented foods, along with yeasts. We have seen some of the LAB’s food benefits in other posts in this blog: prehistoric cheeses, or breast milk microbiota, and even wine bacteria.
Therefore, LAB also have a good role in the production of beers: in particular, as we will see below, in the production of acidified malt, and in some peculiar styles of beer such as the Belgian Lambic and the Berliner Weissbier.
As you know, malt is the raw material for making beer. The cereal is subjected to the malting process, where cereal grains, mainly barley, are germinated, the enzymes hydrolyse the starch into sugars, and all of this is then heated obtaining the must, the substrate solution which will be fermented by the yeasts ferment, producing ethanol and carbon dioxide.
The acidification of the malt, that is, with a lower pH, has the advantages of activating many important enzymes in malting, giving a lower viscosity to the malt and therefore to the final beer. Although adding mineral acids or commercial lactic acid can achieve acidification, it is often recommended or legislated a biological acidification, which is achieved by adding LAB. The use of LAB starter cultures is a relatively new process and in addition to the commented benefits on the quality of the malt, it has been shown to also inhibit unwanted molds that are a real problem in malting and that can give mycotoxins. The compounds produced by LAB that can inhibit the fungi are the same lactic acid and the consequent pH drop, bacteriocins, hydrogen peroxide, and other compounds not well known as perhaps some peptides (Lowe & Arendt 2004).
The most commonly LAB strains used to acidify malt are Lactobacillus amylolyticus previously isolated from the same malt. These strains are moderately thermophile, resistant to compounds derived from humulone, and they have the advantage of being amylolytic in addition to producing lactic acid, which lowers the pH (Vriersekoop et al 2012).
Beers with LAB participating in the fermentation, such as Lambic and Berliner Weissbier styles, belong to the type of spontaneous fermentation beers. The other types of controlled fermentation beers are the best-known Ale and Lager, both inoculated with specific yeasts. Ale beers are those of high fermentation, where Saccharomyces cerevisiae yeast used tends to remain on the surface and the fermentation temperature is above 15-20ºC. Lager ones are those of low fermentation, originally from Bavaria, where yeast S. pastorianus (S. carlsbergensis) tends to settle at the bottom of the fermenter and the temperature is between 7 and 13ºC.
Belgian Lambic beer
Traditional Belgian beers (in Dutch lambiek or lambik) are known for their sensorial characteristics due to LAB activity. They are traditional in Brussels itself and in the neighbouring region of Pajottenland, in the Zenne river valley, in the Flemish Brabant on the SW of the Belgian capital. One of the villages in this valley is Lembeek, which could be the origin of the name of this beer.
These beers of spontaneous fermentation represent the oldest style of making beer in the developed world, for some centuries. For a few years now (since around 2008), similar beers are made in the USA, called “American coolship ales” (Ray 2014).
Lambic beer is made with barley malt and a minimum of 30% of non-malted wheat. The cones of a special hops, completely dried and aged for 3 years, are added to the must. They are added not for their aroma or bitterness, but rather as antimicrobial, to prevent above all, the growth of gram-positive pathogenic bacteria in the fermentation broth.
Also to avoid these contaminants and to promote the microbiota typical of the Lambic fermentation, these beers are brewed only between October and May, since in summer there are too many harmful microorganisms in the air that could spoil the beer, and it is necessary to lower the temperature after boiling. Boiling of the must is done intensively, with an evaporation of 30%.
After boiling, the broth is left in open deposits, and in this way the microorganisms of the air present in the fermentation rooms of the brewery (usually at the top of the building) are acquired, and of the outside air, since the tradition says that the windows must be left open. It is assumed that the captured microbes are specific to the Zenne Valley. These open deposits are the koelschip in Dutch (coolship in English), like swimming pools (Figure 4). Being well open, with a lot of surface (about 6 x 6 m) and shallow depth (about 50 cm), they favour the collection of microbes from the room and from the outside. Another purpose of this form is the fastest cooling of boiled broth to start fermentation. They can be made of wood, copper, or stainless steel more recently.
Figure 4. Koelschip (in Dutch) or coolship in English, the open deposits, as swimming-pools, where the Lambic beer process begins (Brasserie Cantillon, Brussels).
The “inoculated” broth in this spontaneous way is left only one night in the coolship, and on the following day this must is pumped into fermentation tanks where there will stay a year, during which the sugar content will go down, up to about 30 g/L. Then it is transferred to oak barrels, previously used for sherry or port, and there it can be left for another two years, at temperatures of 15-25ºC. Some barrels are the same used since 100 years ago. The final product is a cloudy beer, with a pale yellow, very little CO2, dry, acidic, with about 6-8º of ethanol. It reminds a bit like the sherry and especially the cider, and with a slightly bitter taste (Jackson 1999).
In this long process of fermentation, up to 3 years, of course there is a diversity in the composition of the microbial population. In a first phase there is a certain predominance of Kloeckera yeasts and especially enterobacteria during the first month. After 2 months, Pediococcus damnosus and Saccharomyces spp. predominate, and alcoholic fermentation begins. After 6 months of fermentation the predominant yeast is Dekkera bruxellensis (Spitaels et al 2014), or what is the same, Brettanomyces (Kumara & Verachtert 1991), of which Dekkera is the sexual form.
Figure 5. Species of isolates in MRS and VRBG agar media, for lactic acid bacteria and enterobacteria respectively, during the process of making a Lambic beer. The number of isolates is given between brackets (Spitaels et al 2014).
We see (Figure 5) as in particular after 2 months the predominant bacterium is the LAB P. damnosus. It was appointed in the first studies as “P. cerevisiae“, but this name was finally not admitted because it included other species. The count of these in MRS is 104UFC per mL until the end of fermentation. Acidification seems to be rapidly taking place in the transition from the first stage to that of maturation, coinciding with the growth of P. damnosus, which produces lactic acid, although Dekkera/Brettanomyces and acetic acid bacteria also contribute to the acidification (Spitaels et to 2014).
In other trials with the American coolship ales (ACA) of Lambic style, Lactobacillus spp. have also been found, and in a metagenomic study (Bukolich et al 2012) of these ACA, DNA of several Lactobacillales has been detected. At the end of the process, a predominance of Pediococcus (Figure 6, panel C) was also observed. In the same figure in panel A we observe how the predominant unicellular fungus is also Dekkera/Brettanomyces.
Figure 6. TRFLP analysis (polymorphisms of lengths of PCR-amplified terminal restriction fragments) of total DNA extracted from the fermentation samples of ACA beers (similar to Lambic) during 3 years, using primers for: ITS1/ITS4 of 26S rDNA for yeasts (panel A), 16S rDNA for bacteria (panel B), and specific ones for LAB (panel C). Samples marked with * did not give amplification (Bukolich et al 2012).
Lambic derived beers: Gueuze, Faro, fruity and others
The basic Lambic, which is difficult to purchase, is only found in a few Brussels cafes and the production area. In fact, Lambic is the basis for elaborating the others, much more common to consume:
The Faro is a Lambic sweetened with brown sugar and sometimes with spices.
The fruity Lambic are those that have been added whole fruits or fruit syrup. They can be with bitter cherry (kriek), which are the most traditional, or with raspberry, peach, grapes, strawberry, and sometimes also apple or pineapple or apricot or other.
And finally, the Gueuze, which are sparkling and easy to find. They are made by mixing young Lambics (from 6 months to 1 year) with other more mature ones (2-3 years) in thick glass bottles similar to those of champagne or cava and left for a second fermentation with the remaining sugars from the young Lambic. This would have been begun by a mayor of Lembeek in 1870 that owned a brewery and applied the fermentation techniques in the bottle that had been successful in the Champagne some years before (Cervesa en català 2012). The word Gueuze can have the same etymological origin as gist(yeast in Flemish) and it could also refer to the fact that it produces bubbles of CO2, that is, gas (Jackson 1999). However, another historical version would be that this beer was called “Lambic de chez le gueux” (Welsh from poor people) because the mentioned mayor of Lembeek had similar socialist ideas to those of the “Parti des Gueus” founded by the Calvinists from Flanders in the 16th century to fight against the Spanish empire. And since beer is feminine in French, the gueuxfeminine is gueuze, here it is.
In this refermentation in the bottle the populations of Dekkera/Brettanomycesand LAB are maintained, although other unicellular fungi such as Candida, Hansenula, Pichia or Cryptococcus (Verachtert & Debourg 1999) appear in limited numbers.
Figure 7. Several beer Gueuze and fruity Lambic, mostly Belgian (from www.swanbournecellars.com.au/).
The Berliner Weissbier (Figure 8) is another beer relatively similar to Lambic ones. It is also brewed with an important part of wheat must, it is cloudy, acidic and with 3% ethanol. It is traditional in Berlin and the north of Germany, made from the s. XVI and the most popular alcoholic beverage in Berlin until the end of the s. XIX. It was called the “northern champagne” by the Napoleon’s soldiers. Spontaneous fermentation of must involves a mixture of Dekkera/Brettanomyces, Saccharomycesand hetero-fermentative Lactobacillus.
Figure 8. Berliner Weisse beer (from G-LO, @boozedancing wordpress).
Beers similar to Lambic brewed in Spain
In the same way that the commented American Coolship Ales, Lambic style beers are also made in many other countries and, in the case of Spain, coinciding with the boom of artisanal beers, they are also elaborated, especially the fruity Lambic ones. According to the Birrapedia website, 6 of these are currently being processed, all of which are cherries. Two of them are made in Lleida, one in Barcelona, one in Alicante, one in the Jerte valley, and another in Asturias.
Resistance of lactic acid bacteria from beer to hop compounds
Lactobacillus and Pediococcus, both bad and good we have seen, and other contaminating bacteria of beers, have the ability to withstand hop compounds, which, as we have seen, are natural microbiocides. This resistance can be due to various defence systems, both active and passive (Sakamoto & Konings 2003). The active systems include efflux pumps, such as HorA and HorC, which carry the iso-alpha-acids (Figure 1) out the cell. HorA does it with ATP consumption, and HorC using the proton driving force (Figure 9). The corresponding genes horA and horC were originally found in L. brevis, but later they were also found in L. lindneri, L. paracollinoides and in the best known P. damnosus(Suzuki et al., 2006).
Curiously, HorA shows a resemblance of 54% to OmrA, a membrane transporter of Oenococcus oeni, related to the tolerance of this bacterium from wine to ethanol and other stressors (Bourdineaud et al 2004) (See some more about O. oeni in my post on the bacteria of the vine and the wine). Therefore, it is probable that HorA also has functions of exclusion of other compounds aside from those of the hops. It has been seen that these horAand horC resistance genes and their flanking regions are well preserved and have sequences almost identical to the different species that have them. Therefore, it is very likely that some have been acquired from others by means of horizontal gene transfer, by plasmids or transposons, as is usual in many other bacteria (Suzuki 2011).
Figure 9. Mechanisms of resistance to hop compounds in Lactobacillus brevis (Suzuki 2011).
As we see in Figure 9, protons are pumped out by an ATPase, and the consumption of ATPs is compensated by forming it thanks to the consumption of substrates such as citrate, malate, pyruvate or arginine. Another mechanism of resistance, passive in this case, is the modification of the composition of membrane fatty acids, with the addition of more saturated ones, such as C16:0, which reduces the membrane fluidity and makes it difficult the entrance of the hop compounds. This also reminds us of the changes in membrane of O. oeni related to the resistance to ethanol (Margalef-Català et al 2016). The cell wall also changes its composition in the presence of the hop alpha-iso-acids, increasing the amount of high molecular weight lipoteichoic acid, which would also be a barrier. We also see (Figure 9) how hop compounds can lower the intracellular levels of Mn2+, and then a greater synthesis of Mn-dependent proteins is observed, and a greater capture of Mn2+ from outside. Finally, cells of L. brevis reduce their size when they are in beer (Figure 10), probably in order to decrease the extracellular surface, thus minimizing the effect of external toxic compounds (Suzuki 2011).
Figure 10. Effects of beer adaptation (left) in the size of Lactobacillus brevis cells compared to well grown cells in rich media MRS (right). The bars are 5 mm (Suzuki 2011).
All these mechanisms have been studied in L. brevis strains harmful to beer, but it is assumed that the resistance of beneficial bacteria from Lambic and others would be due to the same mechanisms, since they are of the same bacterial species.
As a conclusion to all said, we see that LAB have outstanding roles as beneficial in various aspects of brewery and malting, despite their most known role of harmful in the processing of the most common beers.
Bibliography
Birrapedia (seen 18 august 2018) Cervezas de tipo Fruit Lambic elaboradas en España. https://birrapedia.com/cervezas/del-tipo-fruit-lambic-elaboradas-en-espana
Bokulich NA et al (2012) Brewhouse resident microbiota are responsible for multi-stage fermentation of American Coolship Ale. PLoS One, 7, e35507
Bourdineaud J et al (2004) A bacterial gene homologous to ABC transporters protect Oenococcus oeni from ethanol and other stress factors in wine. Int J Food Microbiol 92, 1-14.
Cervesa en català (2012) Fitxes de degustació – Timmermans Gueuze Tradition http://cervesaencatala.blogspot.com.es/2012/06/fitxes-de-degustacio-timmermans-gueuze.html
Jackson, Michael (1999) Belgium’s great beers. Beer Hunter Online, July 30, 1999
Kumara HMCS & Verachtert H (1991) Identification of Lambic super attenuating micro-organisms by the use of selective antibiotics. J Inst Brew 97, 181-185
Loret S et al (2005) Levels of biogenic amines as a measure of the quality of the beer fermentation process: data from Belgian samples. Food Chem 89, 519-525
Lowe DP & Arendt EK (2004) The use and effects of lactic acid bacteria in malting and brewing with their relationships to antifungal activity, mycotoxins and gushing: a review. J Inst Brew 110, 163-180
Margalef-Català et al (2016) Protective role of glutathione addition against wine-related stress in Oenococcus oeni. Food Res Int 90, 8-15
Menz G et al (2009) Pathogens in beer, in Beer in Health and Disease Prevention, (Preedy, V. R. Ed.), 403–413, Academic Press, Amsterdam
Ray AL (2014) Coolships rising: the next frontier of sour beers in the U.S. First we feast 27 feb 2014
Sakamoto K & Konings WN (2003) Beer spoilage bacteria and hop resistance. Int J Food Microbiol 89, 105-124
Spitaels F et al (2014) The microbial diversity of traditional spontaneously fermented lambic beer. PLOS One 9, 4, e95384
Suzuki K et al (2006) A review of hop resistance in beer spoilage lactic acid bacteria. J Inst Brew 112, 173-191
Suzuki K (2011) 125th Anniversary Review: microbiological instability of beer caused by spoilage bacteria. J Inst Brew 117, 131-155
The Beer Wench (2008) My obsession with wild beers. Nov. 20, 2008 https://thecolumbuswench.wordpress.com/tag/lambic/
Verachtert H & Debourg A (1999) The production of gueuze and related refreshing acid beers. Cerevisia, 20, 37–41
Vriesekoop F et al (2012) 125th Anniversary review: Bacteria in brewing: the good, the bad and the ugly. J Inst Brew 118, 335-345
Bacillus as probiotics
12th August 2017
The probiotics
Probiotics are living microorganisms that, when ingested in adequate amounts, can have a positive effect on the health of guests (FAO / WHO 2006; World Gastroenterology Organization 2011, Fontana et al., 2013). Guests can be humans but also other animals. Lactic acid bacteria, especially the genus Lactobacillus and Bifidobacterium, both considered as GRAS (Generally recognized as safe), are the microbes most commonly used as probiotics, but other bacteria and some yeasts can also be useful. Apart from being able to be administered as medications, probiotics are commonly consumed for millennia as part of fermented foods, such as yoghurt and other dairy products (see my article “European cheese from 7400 years ago..” “December 26th, 2012). As medications, probiotics are generally sold without prescription, over-the-counter (OTC) in pharmacies.
I have already commented on the other posts of this blog the relevance of probiotics (“A new probiotic modulates microbiota against hepatocellular carcinoma” August 24th, 2016), as well as the microbiota that coexists with our body (“Bacteria in the gut controlling what we eat” October 12th, 2014; “The good bacteria of breast milk” February 3rd, 2013) and other animals (“Human skin microbiota … and our dog” December 25th, 2015; “The herbivore giant panda …. and its carnivore microbiota” September 30th, 2015).
Besides lactic acid bacteria and bifidobacteria, other microorganisms that are also used to a certain extent as probiotics are the yeast Saccharomyces cerevisiae, some strains of Escherichia coli, and some Bacillus, as we will see. Some clostridia are also used, related to what I commented in a previous post of this blog by March 21st, 2015 (“We have good clostridia in the gut ...”).
The Bacillus
In fact, Bacillus and clostridia have in common the ability to form endospores. And both groups are gram-positive bacteria, within the taxonomic phylum Firmicutes (Figure 1), which also includes lactic acid bacteria. However, bacilli (Bacillus and similar ones, but also Staphylococcus and Listeria) are more evolutionarily closer to lactobacillalles (lactic acid bacteria) than to clostridia ones. The main physiological difference between Clostridium and Bacillus is that the first are strict anaerobes while Bacillus are aerobic or facultative anaerobic.
Figure 1. Phylogenetic tree diagram of Gram-positive bacteria (Firmicutes and Actinobacteria). Own elaboration.
Bacterial endospores (Figure 2) are the most resistant biological structures, as they survive extreme harsh environments, such as UV and gamma radiation, dryness, lysozyme, high temperatures (they are the reference for thermal sterilization calculations), lack of nutrients and chemical disinfectants. They are found in the soil and in the water, where they can survive for very long periods of time.
Figure 2. Endospores (white parts) of Bacillus subtilis in formation (Image of Simon Cutting).
Bacillus in fermented foods, especially Asian
Several Bacillus are classically involved in food fermentation processes, especially due to their protease production capacity. During fermentation, this contributes to nutritional enrichment with amino acids resulting from enzymatic proteolysis.
Some of these foods are fermented rice flour noodles, typical of Thailand and Burma (nowadays officially Myanmar). It has been seen that a variety of microorganisms (lactic acid bacteria, yeasts and other fungi) are involved in this fermentation, but also aerobic bacteria such as B. subtilis. It has been found that their proteolytic activity digests and eliminates protein rice substrates that are allergenic, such as azocasein, and therefore they have a beneficial activity for the health of consumers (Phromraksa et al. 2009).
However, the best-known fermented foods with Bacillus are the alkaline fermented soybeans. As you know, soy (Glycine max) or soya beans are one of the most historically consumed nourishing vegetables, especially in Asian countries. From they are obtained “soy milk”, soybean meal, soybean oil, soybean concentrate, soy yogurt, tofu (soaked milk), and fermented products such as soy sauce, tempeh, miso and other ones. Most of them are made with the mushroom Rhizopus, whose growth is favoured by acidification or by direct inoculation of this fungus. On the other hand, if soy beans are left to ferment only with water, the predominant natural microbes fermenting soy are Bacillus, and in this way, among other things, the Korean “chongkukjang” is obtained, “Kinema” in India, the “thua nao” in northern Taiwan, the Chinese “douchi”, the “chine pepoke” from Burma, and the best known, the Japanese “natto” (Figure 3). Spontaneous fermentation with Bacillus gives ammonium as a by-product, and therefore is alkaline, which gives a smell not very good to many of these products. Nevertheless, natto is made with a selected strain of B. subtilis that gives a smoother and more pleasant smell (Chukeatirote 2015).
These foods are good from the nutritional point of view as they contain proteins, fibre, vitamins, and they are of vegetable or microbial origin. In addition, the advertising of the commercial natto emphasizes, besides being handmade and sold fresh (not frozen), its probiotic qualities, saying that B. subtilis (Figure 4) promotes health in gastrointestinal, immunologic, cardiovascular and osseous systems (www.nyrture.com). They say the taste and texture of natto are exquisite. It is eaten with rice or other ingredients and sauces, and also in the maki sushi. We must try it !
Figure 3. “Natto”, soybeans fermented with B. subtilis, in a typical Japanese breakfast with rice (Pinterest.com).
Figure 4. Coloured electronic micrograph of Bacillus subtilis (Nyrture.com).
Bacillus as probiotics
The endospores are the main advantage of Bacillus being used as probiotics, thanks to their thermal stability and to survive in the gastric conditions (Cutting 2011). Although Clostridium has also this advantage, its strict anaerobic condition makes its manipulation more complex, and moreover, for the “bad reputation” of this genus due to some well-known toxic species.
Unlike other probiotics such as Lactobacillus or Bifidobacterium, Bacillus endospores can be stored indefinitely without water. The commercial products are administered in doses of 10^9 spores per gram or per ml.
There are more and more commercial products of probiotics containing Bacillus, both for human consumption (Table 1) and for veterinary use (Table 2). In addition, there are also five specific products for aquaculture with several Bacillus, and also shrimp farms are often using products of human consumption (Cutting 2011).
For use in aquaculture, probiotic products of mixtures of Bacillus (B. thuringiensis, B. megaterium, B. polymixa, B. licheniformis and B. subtilis) have been obtained by isolating them from the bowel of the prawn Penaeus monodon infected with vibriosis. They have been selected based on nutrient biodegradation and the inhibitory capacity against the pathogen Vibrio harveyi (Vaseeharan & Ramasamy 2003). They are prepared freeze-dried or microencapsulated in sodium alginate, and it has been shown to significantly improve the growth and survival of shrimp (Nimrat et al., 2012).
As we see for human consumption products, almost half of the brands (10 of 25) are made in Vietnam. The use of probiotic Bacillus in this country is more developed than in any other, but the reasons are not clear. Curiously, as in other countries in Southeast Asia, there is no concept of dietary supplements and probiotics such as Bacillus are only sold as medications approved by the Ministry of Health. They are prescribed for rotavirus infection (childhood diarrhoea) or immune stimulation against poisoning, or are very commonly used as a therapy against enteric infections. However, it is not clear that clinical trials have been carried out, and they are easy-to-buy products (Cutting 2011).
Table 1. Commercial products of probiotics with Bacillus, for human consumption (modified from Cutting 2011).
Product | Country where it is made | Species of Bacillus |
Bactisubtil ® | France | B. cereus |
Bibactyl ® | Vietnam | B. subtilis |
Bidisubtilis ® | Vietnam | B. cereus |
Bio-Acimin ® | Vietnam | B. cereus and 2 other |
Biobaby ® | Vietnam | B. subtilis and 2 other |
Bio-Kult ® | United Kingdom | B. subtilis and 13 other |
Biosporin ® | Ukraine | B. subtilis + B. licheniformis |
Biosubtyl ® | Vietnam | B. cereus |
Biosubtyl DL ® | Vietnam | B. subtilis and 1 other |
Biosubtyl I and II ® | Vietnam | B. pumilus |
Biovicerin ® | Brazil | B. cereus |
Bispan ® | South Korea | B. polyfermenticus |
Domuvar ® | Italy | B. clausii |
Enterogermina ® | Italy | B. clausii |
Flora-Balance ® | United States | B. laterosporus * |
Ildong Biovita ® | Vietnam | B. subtilis and 2 other |
Lactipan Plus ® | Italy | B. subtilis * |
Lactospore ® | United States | B. coagulans * |
Medilac-Vita ® | China | B. subtilis |
Nature’s First Food ® | United States | 42 strains, including 4 B. |
Neolactoflorene ® | Italy | B. coagulans * and 2 other |
Pastylbio ® | Vietnam | B. subtilis |
Primal Defense ® | United States | B. subtilis |
Subtyl ® | Vietnam | B. cereus |
Sustenex ® | United States | B. coagulans |
* Some labelled as Lactobacillus or other bacteria are really Bacillus
Table 2. Commercial products of probiotics with Bacillus, for veterinary use (modified from Cutting 2011).
Product | Animal | Country where it is made | Species of Bacillus |
AlCare ® | Swine | Australia | B. licheniformis |
BioGrow ® | Poultry, calves and swine | United Kingdom | B. licheniformis and B. subtilis |
BioPlus 2B ® | Piglets, chickens, turkeys | Denmark | B. licheniformis and B. subtilis |
Esporafeed Plus ® | Swine | Spain | B. cereus |
Lactopure ® | Poultry, calves and swine | India | B. coagulans * |
Neoferm BS 10 ® | Poultry, calves and swine | France | B. clausii |
Toyocerin ® | Poultry, calves, rabbits and swine | Japan | B. cereus |
The Bacillus species that we see in these Tables are those that really are found, once the identification is made, since many of these products are poorly labelled as Bacillus subtilis or even as Lactobacillus (Green et al. 1999; Hoa et al. 2000). These labelling errors can be troubling for the consumer, and especially for security issues, since some of the strains found are Bacillus cereus, which has been shown to be related with gastrointestinal infections, since some of them produce enterotoxins (Granum & Lund 1997; Hong et al. 2005)
The probiotic Bacillus have been isolated from various origins. For example, some B. subtilis have been isolated from the aforementioned Korean chongkukjang, which have good characteristics of resistance to the gastrointestinal tract (GI) conditions and they have antimicrobial activity against Listeria, Staphylococcus, Escherichia and even against B. cereus (Lee et al. 2017).
One of the more known probiotics pharmaceuticals is Enterogermina ® (Figure 5), with B. subtilis spores, which is recommended for the treatment of intestinal disorders associated with microbial alterations (Mazza 1994).
Figure 5. Enterogermina ® with spores of Bacillus subtilis (Cutting 2011)
Bacillus in the gastrointestinal tract: can they survive there ?
It has been discussed whether administered spores can germinate in the GI tract. Working with mice, Casula & Cutting (2002) have used modified B. subtilis, with a chimeric gene ftsH-lacZ, which is expressed only in vegetative cells, which can be detected by RT-PCR up to only 100 bacteria. In this way they have seen that the spores germinate in significant numbers in the jejunum and in the ileum. That is, spores could colonize the small intestine, albeit temporarily.
Similarly, Duc et al. (2004) have concluded that B. subtilis spores can germinate in the gut because after the oral treatment of mice, in the faeces are excreted more spores that the swallowed ones, a sign that they have been able to proliferate. They have also detected, through RT-PCR, mRNA of vegetative bacilli after spore administration, and in addition, it has been observed that the mouse generates an IgG response against bacterial vegetative cells. That is, spores would not be only temporary stagers, but they would germinate into vegetative cells, which would have an active interaction with the host cells or the microbiota, increasing the probiotic effect.
With all this, perhaps it would be necessary to consider many Bacillus as not allochthonous of the GI tract, but as bacteria with a bimodal growth and sporulation life cycle, both in the environment and in the GI tract of many animals (Hong et al. 2005).
Regarding the normal presence of Bacillus in the intestine, when the different microorganisms inhabiting the human GI tract are studied for metagenomic DNA analysis of the microbiota, the genus Bacillus does not appear (Xiao et al., 2015). As we can see (Figure 6), the most common are Bacteroides and Clostridium, followed by various enterobacteria and others, including bifidobacteria.
Figure 6. The 20 bacterial genera more abundant in the mice (left) and human (right) GI tract (Xiao et al. 2015).
In spite of this, several species of Bacillus have been isolated from the GI tract of chickens, treating faecal samples with heat and ethanol to select only the spores, followed by aerobic incubation (Barbosa et al. 2005). More specifically, the presence of B. subtilis in the human microbiota has been confirmed by selective isolation from biopsies of ileum and also from faecal samples (Hong et al. 2009). These strains of B. subtilis exhibited great diversity and had the ability to form biofilms, to sporulate in anaerobiosis and to secrete antimicrobials, thereby confirming the adaptation of these bacteria to the intestine. In this way, these bacteria can be considered intestinal commensals, and not only soil bacteria.
Security of Bacillus as probiotics
The oral consumption of important amounts of viable microorganisms that are not very usual in the GI treatment raises additional doubts about safety. Even more in the use of species that do not have a history of safe use in foods, as is the case of sporulated bacteria. Even normal bowel residents may sometimes act as opportunistic pathogens (Sanders et al. 2003).
With the exception of B. anthracis and B. cereus, the various species of Bacillus are generally not considered pathogenic. Of course, Bacillus spores are commonly consumed inadvertently with foods and in some fermented ones. Although Bacillus are recognized as GRAS for the production of enzymes, so far the FDA has not guaranteed the status of GRAS for any sporulated bacteria with application as a probiotic, neither Bacillus nor Clostridium. While Lactobacillus and Bifidobacterium have been the subject of numerous and rigorous tests of chronic and acute non-toxicity, and a lot of experts have reviewed data and have concluded that they are safe as probiotics, there is no toxicity data published on Bacillus in relation to their use as probiotics. When reviewing articles on Medline with the term “probiotic” and limited to clinical studies, 123 references appear, but Bacillus does not appear in any of them (Sanders et al. 2003).
Instead, there are some clinical studies where Bacillus strains have been detected as toxigenic. All this explains that some probiotic Bacillus producers refer to them with the misleading name of Lactobacillus sporogenes, a non-existent species, as can be seen from NCBI (https://www.ncbi.nlm.nih.gov/taxonomy/?term = Lactobacillus + sporogenes).
Finally, we should remember the joint report on probiotics of FAO (United Nations Food and Agriculture Organization) and WHO (World Health Organization) (FAO / WHO 2006), which suggests a set of Guidelines for a product to be used as a probiotic, alone or in the form of a new food supplement. These recommendations are:
- The microorganism should be well characterized at the species level, using phenotypic and genotypic methods (e.g. 16S rRNA).
- The strain in question should be deposited in an internationally recognized culture collection.
- To evaluate the strain in vitro to determine the absence of virulence factors: it should not be cytotoxic neither invades epithelial cells, and not produce enterotoxins or haemolysins or lecithinases.
- Determination of its antimicrobial activity, and the resistance profile, including the absence of resistance genes and the inability to transfer resistance factors.
- Preclinical evaluation of its safety in animal models.
- Confirmation in animals demonstrating its effectiveness.
- Human evaluation (Phase I) of its safety.
- Human evaluation (Phase II) of its effectiveness (if it does the expected effect) and efficiency (with minimal resources and minimum time).
- Correct labelling of the product, including genus and species, precise dosage and conservation conditions.
Conclusions
The use of Bacillus as probiotics, especially in the form of dietary supplements, is increasing very rapidly. More and more scientific studies show their benefits, such as immune stimulation, antimicrobial activities and exclusive competition. Their main advantage is that they can be produced easily and that the final product, the spores, is very stable, which can easily be incorporated into daily food. In addition, there are studies that suggest that these bacteria may multiply in GI treatment and may be considered as temporary stagers (Cutting 2011).
On the other hand, it is necessary to ask for greater rigor in the selection and control of the Bacillus used, since some, if not well identified, could be cause of intestinal disorders. In any case, since the number of products sold as probiotics that contain the sporulated Bacillus is increasing a lot, one must not assume that all are safe and they must be evaluated on a case-by-case basis (Hong et al. 2005).
Bibliography
Barbosa TM, Serra CR, La Ragione RM, Woodward MJ, Henriques AO (2005) Screening for Bacillus isolates in the broiler gastrointestinal tract. Appl Environ Microbiol 71, 968-978.
Casula G, Cutting SM (2002) Bacillus probiotics: Spore germination in the gastrointestinal tract. Appl Environ Microbiol 68, 2344-2352.
Chukeatirote E (2015) Thua nao: Thai fermented soybean. J Ethnic Foods 2, 115-118.
Cutting SM (2011) Bacillus probiotics. Food Microbiol 28, 214-220.
Duc LH, Hong HA, Barbosa TM, Henriques AO, Cutting SM (2004) Characterization of Bacillus probiotics available for human use. Appl Environ Microbiol 70, 2161-2171.
FAO/WHO (2006) Probiotics in food. Health and nutritional properties and guidelines for evaluation. Fao Food and Nutrition Paper 85. Reports of Joint FAO/WHO expert consultations.
Fontana L, Bermudez-Brito M, Plaza-Diaz J, Muñoz-Quezada S, Gil A (2013) Sources, isolation, characterization and evaluation of probiotics. Brit J Nutrition 109, S35-S50.
Granum, P. E., T. Lund (1997) Bacillus cereus and its food poisoning toxins. FEMS Microbiol. Lett. 157:223–228.
Green, D. H., P. R. Wakeley, A. Page, A. Barnes, L. Baccigalupi, E. Ricca, S. M. Cutting (1999) Characterization of two Bacillus probiotics. Appl Environ Microbiol 65, 4288–4291.
Hoa, N. T., L. Baccigalupi, A. Huxham, A. Smertenko, P. H. Van, S. Ammendola, E. Ricca, A. S. Cutting (2000) Characterization of Bacillus species used for oral bacteriotherapy and bacterioprophylaxis of gastrointestinal disorders. Appl Environ Microbiol 66, 5241–5247.
Hong HA, Dic LH, Cutting SM (2005) The use of bacterial spore formers as probiotics. FEMS Microbiol Rev 29, 813-835.
Hong HA, Khaneja R, Tam NMK, Cazzato A, Tan S, Urdaci M, Brisson A, Gasbarrini A, Barnes I, Cutting SM (2009) Bacillus subtilis isolated from the human gastrointestinal tract. Res Microbiol 160, 134-143.
Lee S, Lee J, Jin YI, Jeong JC, Hyuk YH, Lee Y, Jeong Y, Kim M (2017) Probiotic characteristics of Bacillus strains isolated from Korean traditional soy sauce. LWT – Food Sci Technol 79, 518-524.
Mazza P (1994) The use of Bacillus subtilis as an antidiarrhoeal microorganism. Boll Chim. Farm. 133, 3-18.
Nimrat S, Suksawat S, Boonthai T, Vuthiphandchai V (2012) Potential Bacillus probiotics enhance bacterial numbers, water quality and growth during early development of white shrimp (Litopenaeus vannamei). Veterinary Microbiol 159, 443-450.
Phromraksa P, Nagano H, Kanamaru Y, Izumi H, Yamada C, Khamboonruang C (2009) Characterization of Bacillus subtilis isolated from Asian fermented foods. Food Sci Technol Res 15, 659-666.
Sanders ME, Morelli L, Tompkins TA (2003) Sporeformers as human probiotics: Bacillus, Sporolactobacillus, and Brevibacillus. Compr Rev Food Sci Food Safety 2, 101-110
Vaseeharan, B., P. Ramasamy (2003) Control of pathogenic Vibrio spp. by Bacillus subtilis BT23, a possible probiotic treatment for black tiger shrimp Penaeus monodon. Lett Appl Microbiol 36, 83–87
World Gastroenterology Organisation Global Guidelines (2011) Probiotics and Prebiotics.
Xiao et al. (2015) A catalogue of the mouse gut metagenome. Nature Biotechnol 33, 1103-1108.
Bacteria in the gut are controlling what we eat
It seems to be so: the microbes in our gastrointestinal tract (GIT) influence our choice of food. No wonder: microbes, primarily bacteria, are present in significant amounts in GIT, more than 10 bacterial cells for each of our cells, a total of 1014 (The human body has about 1013 cells). This amounts to about 1-1.5 kg. And these bacteria have lived with us always, since all mammals have them. So, they have evolved with our ancestors and therefore they are well suited to our internal environment. Being our bodies their habitat, much the better if they can control what reaches the intestine. And how can they do? Then giving orders to the brain to eat such a thing or that other, appropriate for them, the microbes.
Figure 1. “Command centre of the gastrointestinal tract” (own assembly, Albert Bordons)
Well, gone seriously, there is some previous work in this direction. It seems there is a relationship between preferences for a particular diet and microbial composition of GIT (Norris et al 2013). In fact, it is a two-way interaction, one of the many aspects of symbiotic mutualism between us and our microbiota (Dethlefsen et al 2007).
There is much evidence that diet influences the microbiota. One of the most striking examples is that African children fed almost exclusively in sorghum have more cellulolytic microbes than other children (De Filippo et al 2010).
The brain can also indirectly influence the gut microbiota by changes in intestinal motility, secretion and permeability, or directly releasing specific molecules to the gut digestive lumen from the sub epithelial cells (neurons or from the immune system) (Rhee et al 2009).
The GIT is a complex ecosystem where different species of bacteria and other microorganisms must compete and cooperate among themselves and with the host cells. The food ingested by the host (human or other mammal) is an important factor in the continuous selection of these microbes and the nature of food is often determined by the preferences of the host. Those bacteria that are able to manipulate these preferences will have advantages over those that are not (Norris et al 2013).
Recently Alcock et al (2014) have reviewed the evidences of all this. Microbes can manipulate the feeding behaviour of the host in their own benefit through various possible strategies. We’ll see some examples in relation to the scheme of Figure 2.
Figure 2. As if microbes were puppeteers and we humans were the puppets, microbes can control what we eat by a number of marked mechanisms. Adapted from Alcock et al 2014.
People who have “desires” of chocolate have different microbial metabolites in urine from people indifferent to chocolate, despite having the same diet.
Dysphoria, id est, human discomfort until we eat food which improve microbial “welfare”, may be due to the expression of bacterial virulence genes and perception of pain by the host. This is because the production of toxins is often triggered by a low concentration of nutrients limiting growth. The detection of sugars and other nutrients regulates virulence and growth of various microbes. These directly injure the intestinal epithelium when nutrients are absent. According to this hypothesis, it has been shown that bacterial virulence proteins activate pain receptors. It has been shown that fasting in mice increases the perception of pain by a mechanism of vagal nerve.
Microbes can also alter food preferences of guests changing the expression of taste receptors on the host. In this sense, for instance germ-free mice prefer more sweet food and have a greater number of sweet receptors on the tongue and intestine that mice with a normal microbiota.
The feeding behaviour of the host can also be manipulated by microbes through the nervous system, through the vagus nerve, which connects the 100 million neurons of the enteric nervous system from the gut to the brain via the medulla. Enteric nerves have receptors that react to the presence of certain bacteria and bacterial metabolites such as short chain fatty acids. The vagus nerve regulates eating behaviour and body weight. It has been seen that the activity of the vagus nerve of rats stimulated with norepinephrine causes that they keep eating despite being satiated. This suggests that GIT microbes produce neurotransmitters that can contribute to overeating.
Neurotransmitters produced by microbes are analogue compounds to mammalian hormones related to mood and behaviour. More than 50% of dopamine and most of serotonin in the body have an intestinal origin. Many persistent and transient inhabitants of the gut, including E. coli, several Bacillus, Staphylococcus and Proteus secrete dopamine. In Table 1 we can see the various neurotransmitters produced by GIT microbes. At the same time, it is known that host enzymes such as amine oxidase can degrade neurotransmitters produced by microorganisms, which demonstrates the evolutionary interactions between microbes and hosts.
Table 1. Diversity of neurotransmitters isolated from several microbial species (Roschchina 2010)
Neurotransmitter | Genera |
GABA (gamma-amino-butyric acid) | Lactobacillus, Bifidobacterium |
Norepinephrine | Escherichia, Bacillus, Saccharomyces |
Serotonin | Candida, Streptococcus, Escherichia, Enterococcus |
Dopamine | Bacillus, Serratia |
Acetylcholine | Lactobacillus |
Some bacteria induce hosts to provide their favourite nutrients. For example, Bacteroides thetaiotaomicron inhabits the intestinal mucus, where it feeds on oligosaccharides secreted by goblet cells of the intestine, and this bacterium induces its host mammal to increase the secretion of these oligosaccharides. Instead, Faecalibacterium prausnitzii, a not degrading mucus, which is associated with B. thetaiotaomicron, inhibits the mucus production. Therefore, this is an ecosystem with multiple agents that interact with each other and with the host.
As microbiota is easily manipulated by prebiotics, probiotics, antibiotics, faecal transplants, and changes in diet, controlling and altering our microbiota provides a viable method to the otherwise insoluble problems of obesity and poor diet.
References
Alcock J, Maley CC, Aktipis CA (2014) Is eating behavior manipulated by the gastrointestinal microbiota? Evolutionary pressures and potential mechanisms. BioEssays 36, DOI: 10.1002/bies.201400071
De Filippo C, Cavalieri D, Di Paola M, Ramazzotti M, et al (2010) Impact of diet in shaping gut microbiota revealed by a comparative study in children from Europe and rural Africa. Proc Natl Acad Sci USA 107:14691–6
Dethlefsen L, McFall-Ngai M, Relman DA (2007) An ecological and evolutionary perspective on human-microbe mutualism and disease. Nature 449:811-818
Lyte M (2011) Probiotics function mechanistically as delivery for neuroactive compounds: Microbial endocrinology in teh design and use of probiotics. BioEssays 33:574-581
Norris V, Molina F, Gewirtz AT (2013) Hypothesis: bacteria control host appetites. J Bacteriol 195:411–416
Rhee SH, Pothoulakis C, Mayer EA (2009) Principles and clinical implications of the brain–gut–enteric microbiota axis. Nature Reviews Gastroenterology and Hepatology 6:306-314
Roschchina VV (2010) Evolutionary considerations of neurotransmitters in microbial, plant, and animal cells. In Lyte M, Freestone PPE, eds; Microbial Endocrinology: Interkingdom Signaling in Infectious Disease and Health. New York: Springer. pp. 17–52
The good bacteria of breast milk
Breast milk, besides being very nutritious, provides bioactive constituents that favor the development of the infant immune system and prevent diseases. From this point of view, the best known compounds are maternal immunoglobulins, immunocompetent cells and various antimicrobials. It also contains prebiotic substances, ie, several molecules such as oligosaccharides, which stimulate the growth of specific bacteria in the gut of the child.
However, other important constituents of breast milk, unsuspected until few years ago, are the bacteria. In fact, milk is not sterile, it contains microorganisms, primarily beneficial bacteria that help to establish the intestinal microbiota of the newborn, and which are the first to settle there. Although artificial milk are made to resemble the breast milk, they remain distinct and do not contain bacteria. And for this reason, the intestinal microbiota of breast-fed infants is different than those fed with artificial breast milk.
Lactobacilli (image from AJC1Flickr) and suckling baby (© Photos.com)
Just a few weeks ago was published a work ( Cabrera-Rubio et al., 2012 ) in the American Journal of Clinical Nutrition that had a good coverage in media, blogs and networks ( click here for an example), because it shows the great diversity of bacteria present in the breast milk.
Although this work done by Valencian researchers (Cavanilles Institute, University of Valencia and CSIC-IATA) with Finnish researchers is not the first study that examines this issue, this study shows that bacteria are from very diverse species.
One of the novelties of this paper is the method used, taking advantage of the latest molecular biology: they studied the microbiome in breast milk, that is, the analysis of all possible bacteria present in the samples, by DNA sequencing, without the traditional isolation of living bacteria in plates. To do so, from the aseptically collected milk, DNA is extracted and the gene fragments of bacterial 16S rRNA are amplified by PCR. These amplified genes are sequenced by pyrosequencing (454 Roche GS-FLX), the most innovative and rapid sequencing technology: a machine of this allows about 400 million base pairs (bp) of DNA in 10 hours. From the rRNA gene of each possible bacteria some 500 bp are sequenced. Thus, in this study about 120,000 sequences have been analyzed, corresponding to 2600 sequences per milk sample.
By comparing these sequences with the databases and applying statistical methods conclusions can be drawn on what taxonomic groups (genera and species) bacteria are present and in what proportion.
Predominant genera of bacteria in breast milk (Cabrera-Rubio et al., 2012)
As shown in the figure above, Cabrera et al. found in the milk of healthy mothers that the predominant genera are Leuconostoc, Weissella, Lactococcus and Staphylococcus, of which the first three are lactic acid bacteria. Although these are predominant in colostrum and milk during the first months, then other bacteria are increasing their numbers, such as Veillonella Leptotrichia (anaerobic gram-negative bacteria), which are typical commensal of the oral cavity. In total, about 1000 species have been found, that vary depending on the mother. Curiously, there are significant variations on whether delivery had been vaginal or cesarean, and on the obesity of the mother. The reasons for this are not yet clear.
And where the bacteria in breast milk come from ?
Besides the identifications made in this study of Cabrera et al. (2012) on the basis of DNA present, it has been observed by making viable counts that the total number of bacteria in breast milk is between 2·104 and 3·105 per ml (Juan Miguel Rodríguez), that is, a quantity not negligible . What is its origin?
The study of the microbiome of Cabrera et al. also concluded that the composition of different bacteria is somewhat different from that of other bacterial communities in the human body (the human bacterial niches: skin, mouth, digestive system, vagina, etc), and therefore the milk microbiome is not a particular subset of one of these niches.
The group Probilac from Universidad Complutense de Madrid, whose head is Juan Miguel Rodriguez, a friend and colleague of Red BAL (Spanish network of lactic acid bacteria) is working in this area for years (ex: Martin et al 2003 , Martin et al 2004).
As discussed in a recent review published by this group (Fernández et al 2012), the bacteria present in the breast milk would come from three possible sources (figure below): skin bacteria from the same breast, the oral cavity of the infant, and the most surprising, commensal bacteria of the maternal gut that pass to milk by the entero-mammary pathway.
Potential sources of bacteria present in human colostrum and milk, including the transit of intestinal commensal bacteria to the milk by the entero-mammary pathway (Fernández et al., 2012). DC: dendritic cells.
Indeed, several studies had shown that dendritic cells cross the intestinal epithelium (between enterocytes) and may take commensal bacteria of the gut lumen, incorporating them by endocytosis, but keeping them alive. See details in the following diagram.
Dendritic cell capturing gut bacteria (Scheme of J.M. Rodríguez, group Probilac, Univ. Complutense de Madrid).
These dendritic cells travel through the circulatory system, reaching the mammary glands, where it seems that include bacteria to milk. This is the the entero-mammary pathway.
In this breast microbiota, bacteria from breast skin and from oral cavity of the child also would be incorporated. Some of these bacteria the child’s oral cavity are actually related to those of its gastrointestinal tract. As the first bacteria inhabiting this tract are those of the vaginal microbiota during birth (and intestinal if delivery is cesarean), this would explain the phylogeny of certain bacteria in the milk of these microbiota.
In summary, we see as the “good” bacteria (lactic acid bacteria, but also bifidobacteria and other) from maternal gut, by different ways, arrive to breast milk, and the reach the child’s gut, developing there the child’s microbiota, and helping to complete the neonatal immune system.
Bibliography
Cabrera-Rubio R, MC Collado, K Laitinen, S Salminen, E Isolauri, A Mira (2012) The human milk microbiome changes over lactation and is shaped by maternal weight and mode of delivery. American J Clinical Nutrition 96, 544–51
Grupo Probilac (Juan Miguel Rodríguez Gómez) Microbiota de la leche humana en condiciones fisiológicas: http://www.ucm.es/info/probilac/microbiota2.htm, Departamento de Nutrición, Bromatología y Tecnología de los Alimentos, Facultad de Veterinaria, Universidad Complutense de Madrid
Fernández L, S Langa, V Martín, A Maldonado, E Jiménez, R Martín, JM Rodríguez (2012) The human milk microbiota: Origin and potential roles in health and disease. Pharmacological Research http://dx.doi.org/10.1016/j.phrs.2012.09.001
Hunt KM JA Foster, LJ Forney, UME Schütte, DL Beck, Z Abdo, LK Fox, JE Williams, MK McGuire, MA McGuire (2011) Characterization of the diversity and temporal stability of bacterial communities in human milk. PLoS ONE 6:e21313.
Martín R, S Langa, C Revriego, E Jiménez, ML Marín, J Xaus, L Fernández, JM Rodríguez (2003) Human milk is a source of lactic acid bacteria for the infant gut. J Ped. 143, 754-758.
Martín R, S Langa, C reviriego, E Jiménez, ML Marín, M Olivares, J Boza, J Jiménez, L fernández, J Xaus, JM Rodríguez (2004) The commensal microflora of human milk: new perspectives for food bacteriotherapy and probiotics. Trends Food Sci Technol 15:121–7.
Other references
Adlerberth I (2006) Reduced enterobacterial and increased staphylococcal colonization of the infantile bowel: an effect of hygienic lifestyle. Pediatric Res 59, 96-101.
Albesharata R et al (2011) Phenotypic and genotypic analyses of lactic acid bacteria in local fermented food, breast milk and faeces of mothers and their babies. Syst App Microb 34, 148–155
Domínguez-Bello MG et al (2010) Delivery mode shapes the acquisition and structure of the initial microbiota across multiple body habitats in newborns. Proc Natl Acad Sci USA;107:11971–5.
Huurre A et al (2008) Mode of delivery—effects on gut microbiota and humoral immunity. Neonatology;93:236–40
LeBouder E et al (2006) Modulation of neonatal microbial recognition: TLRmediated innate immune responses are specifically and differentially modulated by human milk. J Immunol;176:3742–52.
Martín R et al (2009) Isolation of bifidobacteria from breast milk and assessment of the bifidobacterial population by PCR-denaturing gradient gel electrophoresis and quantitative real-time PCR. Appl Environ Microbiol 75:965–9.
Pérez PF et al (2007) Bacterial imprinting of the neonatal immune system: lessons from maternal cells? Pediatrics 119: 724–732.
Rescigno M et al (2001) Dendritic cells shuttle microbes across gut epithelial monolayers. Immunobiology 204:572–81.
Stockinger S et al (2001) Establishment of intestinal homeostasis during the neonatal period. Cell Mol Life Sci;68: 3699–712.
European cheese from 7400 years ago, and “yoghourt” in the Sahara 7000 years ago
One month ago (12th December 2012) a work (Salque et al. 2012) was published online in Nature, which provides archaeological evidence of cheese making in present Poland about 5400 years before Christ (BC). And in last June was also published another study in Nature (Dunne et al. 2012) which evidenced the production of fermented milk products like yoghourt in northeastern Sahara (now Libya) about 5000 BC.
Using the milk of other animals
Agriculture, that is, the domestication of plants by humans, began between 10000 and 5000 BC mainly in the Middle East (the Fertile Crescent, from the Nile to the Euphrates), but also independently in other regions, such as India, China and various parts of America and Africa. The Neolithic agricultural revolution led to the establishment of sedentary populations and the subsequent birth of cities and civilizations. At the same time, in these sites there were domesticated also animals, but it is likely that the domestication of cattle, sheep and goats have already occurred before, in nomad populations. The use of these animals brought some important advances, using them for secondary uses without killing them (the primary use is the flesh) such as traction, wool, and milk and dairy products.
Nomad Qashqai (Persia) milking a sheep. Photo: M. Kiani
The first pictorial and written records of the use of the milk of domestic animals are from Egypt and Mesopotamia around 3000 BC. But recently, the first clear evidence for previous organic waste stored in ceramic remains has been found, by analyzing the values of δ 13C (ratio between the isotopes 13C and 12C) of the main fatty acids from fat of milk. This technique, from Dudd and Eversheds (1998), is based on the differences between the values of δ 13C of stearic acid (C 18:0) in milk and adipose tissue of the same body of animal, due to the higher proportion of carbon derived from carbohydrates in the diet used in the biosynthesis of C 18:0 in body fat compared to milk, where 40% of C 18:0 derived from unsaturated fats.
This technique of δ 13C has shown the use of milk in the 4th millennium BC in Britain, in the 6th millennium in Eastern Europe, and recently (Eversheds et al. 2008) has been shown that in the 7th millennium BC, 9000 years ago, there was milking in the Middle East and Southeast Europe, particularly in Anatolia.
But when the adults began to drink human milk?
As you know, the lactose of milk is not tolerated by many adults, especially of Asian, Native Americans and many Africans. The enzyme lactase that hydrolyzes lactose into glucose and galactose is present in all the babies, but like all other mammals, when they become older, the gene for lactase is not expressed. The exception is those people that maintain the production of lactase in adulthood and so they can drink milk without problems. For those who do not tolerate milk, the reason is due to lactose fermentation by bacteria in the gut, which gives rise to diarrhea, flatulence and other disorders.
Percentages of human populations not tolerant to lactose. Map made by Rainer Zenz.
The humans more tolerant to lactose are of European origin and those in regions nearby the Sahara and the Middle East. In Europe there is a gradient from high to low tolerance northwest towards the southeast. Molecular biology studies have shown that tolerance to lactose appeared by mutation of a single nucleotide at different times and places, between 8000 and 3000 years ago, in pastoral peoples of northern Europe and Arabia (Swallow 2003, Enattah et al. 2008, Tellam 2012). This genetic characteristic was selected due to its positive nutritional benefits, and also because in the desert milk is a source of water, and also in northern Europe milk can replace the lack of calcium due to low solar radiation and therefore short synthesis of vitamin D needed for calcium absorption.
Cheese and fermented milk products for lactose intolerants
Cheese is the curdled milk from which is extracted, in part or all, the whey, id est, the milk with water soluble components, which are mostly lactose. The remaining precipitate is the cheese, which contains fat and milk protein but very little lactose. Therefore, for people lactose-intolerant cheese is a food nutritionally equivalent to milk, but without the inconvenience of lactose. In addition, cheese is kept longer than milk and takes many different tastes and textures, depending on the curdling process and on microorganisms involved in their maturation. In fermented milk such as yoghourt and other (Kefir, Kumi, Leben, etc.) microorganisms are involved, especially lactic acid bacteria, which consume part of lactose and produce lactic acid, which favors conservation. The content of lactose in these fermented milks is not as low as in cheese but they can be consumed by most lactose intolerant people.
For this reason, the use of various types of cheese and / or fermented milk is almost universal to humans, regardless of whether or not they are tolerant to lactose and probably it existed in various nomad peoples, with the first domesticated animals, and surely this was the first way to use the milk of these animals.
Evidence of cheese made in Europe about 7400 years
As said earlier, a work (Salque et al 2012) has been recently published online in Nature which provides archaeological evidence of cheese making in today’s Poland about 5400 years before Christ (BC).
At the beginning of the Neolithic sites (about 8000 years) from various parts of Europe containers with small holes appear, with shaped sieve, that have been thought for years as strainers cheese, similar to those used today in some regions. The milk is placed in the container, the rennet is added (from the stomach of ruminants, containing protease), and the precipitated curdled is squeezed, separating out the whey through the holes, to get the cheese (Subbaraman 2012).
Drawing representing a reconstructed vessel (left) and a portion of an actual piece of this container (right) with holes as a sieve, from a site of Kuyavia region (in central Poland). Image from Salque et al. (2012).
Salque et al. (2012) have shown by the above mentioned technique δ 13C (in addition to analyze by gas chromatography the composition of lipids) that the remains of fatty acids found at the site of vessel Kuyavia (north of Warsaw) were coming from milk. The fat composition and δ 13C values of these vessels strainers are different from those found in other containers like pots where probably meat of different animals was cooked. Therefore, they demonstrate that these containers were used to make cheeses strainers, specifically about 7400 years ago. The authors emphasize the importance of this type of pottery in the processing of dairy products, indicating in particular the importance for lactose-intolerant prehistoric communities.
Evidence of fermented milk (yoghourt ?) in the Sahara 7000 years ago
As said above, last June another study was published in Nature (Dunne et al. 2012), which evidenced the production of fermented milk in northeastern Sahara (now Libya) about 7000 years ago.
In contrast to the well known process of early Neolithic settlements and agriculture in the Middle East, in the Sahara the pastoralism with cows, sheep and goats began long before the domestication of plants. Seeing the present desert of Sahara, so arid and inhospitable, it seems impossible that human communities prospered there with large herds, but this region enjoyed a very favorable climatically wet period that began some 10.000 years ago and there is plenty of evidence that 8000 years ago there proliferated all types of wildlife in the savannas of the current Sahara. Groups of hunters and gatherers who lived there already used the pottery to preserve food, and gradually, with the increase of the drought, had become more dependent on livestock.
A demonstration of these nomad livestock are the remarkable paintings and rock carvings found in the desert of southwest Libya (Wadi Teshuinat or Takarkori Acacus mountains, or in the area of Wadi Tiksatin Messak) from some 7000 years ago, possibly the most important concentration of prehistoric art in the world, with many scenes of daily life. In these representations it can be seen the importance of livestock for these humans, with drawings of obvious milking cows. However, there is no reliable dating of these prints.
Schematic drawings of Wadi Teshuinat cave, southwest Libya. Figure taken from Dunne et al. (2012).
The group of Julie Dunne and Richard Eversheds at the University of Bristol with the group of Savino di Lernia from University of Sapienza, studied the remains of fat present in the pottery of Takarkori site by gas chromatography coupled with mass spectrometry, and the aforementioned technical isotopes (δ 13C). Their results show that these potteries were used to produce fermented milk products like yoghourt, between 7000 and 4800 years ago. In addition, they found that milk fat came from a variety of plants from different places, which suggests that people were migrating with their herds, depending on the season. This work confirms that the economy of dairy products derived from domesticated cattle was active during this period, probably to compensate for lactose intolerant.
Murzuq ceramics, from the site of Takarkori, Libya. Photo: Savino di Lernia
Following this work, some scientists (Callaway 2012) have suggested that subsequent to this period, the lactose tolerance mutation arose in Europe and Arabia and spread through North Africa due to its advantages. In the increasingly arid climate of the desert, to drink fresh and uncontaminated milk should lead to a better hydration respect to other people which had the tolerance gene not activated. Thus, there was a strong selective pressure for the spread of lactose tolerance in north Africa.
Bibliography
Arjamaa O, T Vuorisalo (2010) Genes, cultura y dieta. Investigación y Ciencia, 405, june 2010, 69-77
Callaway E (2012) Pottery shards put a date on Africa’s dairying. North Africans may have been making yoghurt 7,000 years ago. Nature News, 20 june 2012-12-22
Dudd SN, RP Evershed (1998) Direct Demonstration of Milk as an Element of Archaeological Economies. Science 282, 1478-1481
Dunne J et al (2012) First dairying in green Saharan Africa in the fifth millennium bc. Nature 486, 390–394 (21 June 2012) doi:10.1038/nature11186
Enattah NS et al. (2008) Independent introduction of two lactase-persistence alleles into human populations reflects different history of adaptation to milk culture. Am J Human Genetics 82, 57-72.
Evershed RPet al. (2008) Earliest date for milk use in the Near East and southeastern Europe linked to cattle herding. Nature 455, 528-531 (25 September 2008), doi:10.1038/nature07180
Salque, M. et al. (2012) Earliest evidence for cheese making in the sixth millennium BC in northern Europe. Nature http://dx.doi.org/10.1038/nature11698
Subbaraman, N (2012) Art of cheese-making is 7,500 years old. Neolithic pottery fragments from Europe reveal traces of milk fats. Nature News, 12 dec 2012
Swallow DM (2003) Genetics of lactase persistence and lactose intolerance. Annu. Rev. Genet. 37, 197-219
Tellam R (2012) How dairying shaped the human genome. International Milk Genomics Consortium