by Piter Kehoma Boll
Here is a list of species described this month. It certainly does not include all described species. You can see the list of Journals used in the survey of new species here.
Bacteria
- 16 new actinobacteria: Agrococcus sediminis; Nonomuraea nitratireducens; Nonomuraea antri; Agromyces humi; Streptomyces apocyni; Streptomyces lycii; Streptomyces harenosi; Trebonia kvetii; Bifidobacterium canis; Gulosibacter macacae; Brevibacterium rongguiense; Planctomonas psychrotolerans; Arthrobacter yangruifuii, Arthrobacter zhaoguopingii; Actinomadura rubteroloni, Actinomadura macrotermitis;
- 8 new bacteroids: Hymenobacter artigasi; Hymenobacter fodinae, Hymenobacter metallicola; Algibacter marinivivus; Flavobacterium ichthyis; Agriterribacter humi; Salinimicrobium nanhaiense, Salinimicrobium oceani;
- 2 new cyanobacteria: Affixifilum floridanum, Neolyngbya biscaynensis;
- 3 new firmicutess: Alkalibaculum sporogenes; Paenibacillus algicola; Metabacillus sediminilitoris;
- 1 new hadobacterium: Deinococcus terrestris;
- 1 new kiritimatiellaeote: Tichowtungia aerotolerans;
- 2 new planctomycetes: Symmachiella dynata, Symmachiella macrocystis;
- 28 new proteobacteria: Intestinirhabdus alba; Ideonella livida; Terricaulis silvestris; Ketobacter nezhaii; Pseudomonas viciae; Pseudomonas karstica, Pseudomonas spelaei; Rhizobium rhizophilum; Rhizobium dioscoreae; Bradyrhizobium cosmicum; Paraburkholderia solitsugae, Paraburkholderia elongata; Rhodovarius crocodyli; Vibrio algicola; Nocardia macrotermitis, Nocardia aurantia; Halomonas rituensis, Halomonas zhuhanensis; Aquitalea aquatilis; Zoogloea dura; Amaricoccus solimangrovi; Yersinia artesiana, Yersinia proxima, Yersinia alsatic, Yersina vastinensis, Yersinia thracica, Yersinia occitanica; Kangsaoukella pontilimi;
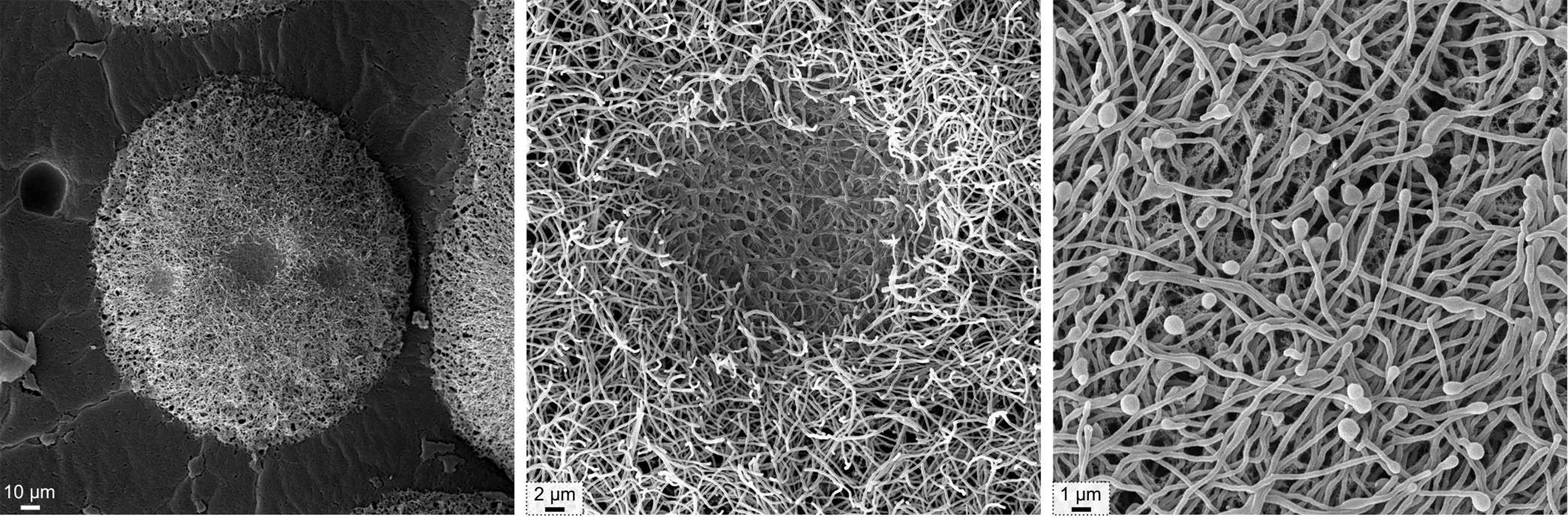
Archaeans
- 1 new euryarchaeote: Halomicroarcula amylolytica;
Excavates
- 5 new metamonads: Cononympha koidzumii, Cononympha monstrummogolloni, Cononympha skunkapei, Holomastigotoides bigfooti, Holomastigotoides batututi;
SARs
- 4 new ciliates: Prototapirella sabyinyo, P. sulcata, P. curiosa; Pseudokeronopsis parasongi;
- 1 new apicomplexan: Acroeimeria grismeri;
- 6 new ochrophytes: Nupela eremica; Brachysira aristidesii; Gyrosigma centropunctatum, G. dongzhaiense; Fistulifera alcalina; Aulacoseira umanai;
- 1 new oomycete: Periplasma isogametum;
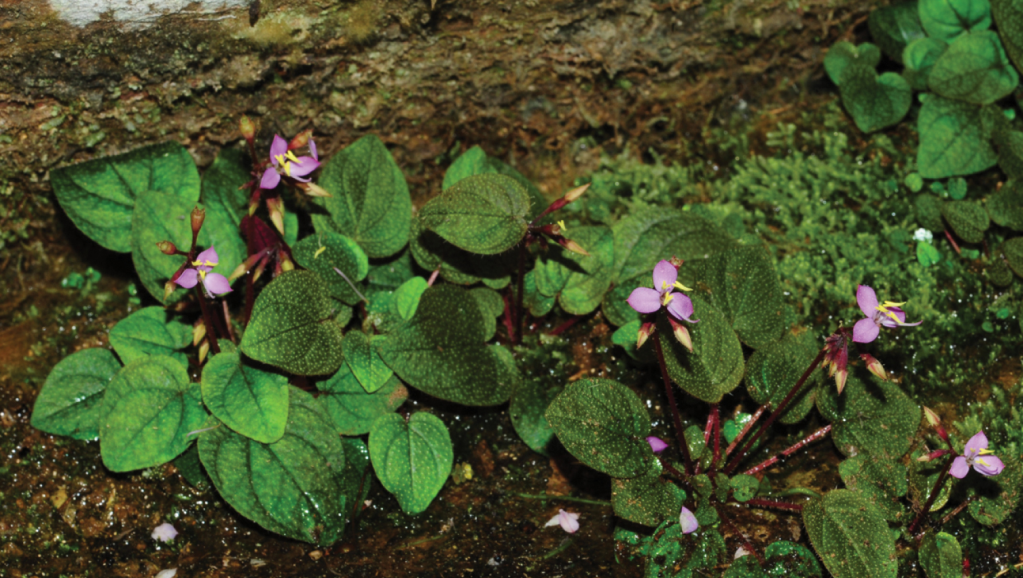
Plants
- 1 new marchantiophyte: Frullania amazonica;
- 1 new bryophyte: Brachymenium ayangannensis;
- 3 new pteridophytes: Hypodematium ryukyuense; Antrophyum nambanense; Hymenophyllum sp.;
- 1 new cycadophyte: Ceratozamia leptoceras;
- 4 new magnoliids: Magnolia poqomchi; Magnolia ottoi; Peperomia pasionana, Peperomia tancitaroana;
- 18 new monocots: Campylocentrum alvesii; Eremitis limae; Aloe vatovavensis, Aloe rakotonasoloi; Dendrochilum ignisiflorum; Lophiaris quichensis; Tillandsia itatiensis; Paepalanthus campanulatus; Peliosanthes nagalandensis, P. tobuensis; Anthurium siapidaarae; Laobambos calcareus; Cryptanthus cinereus; Hechtia elegans, H. ensifolia, H. medusae, H. platyphylla, H. pycnostachya;
- 52 new eudicots: Nepenthes putaiguneung; Microlicia windischii; Lippia krenakiana; Ditaxis grazielae; Phyllanthus pedicellatus; Begonia beijnenii; Cephalaria kurdistanica; Indigofera yuanjiangensis; Lactuca anatolica; Helosciadium milfontinum; Meriania microflora; Meriania ardyae, Meriania zunacensis; Stylosanthes minima, S. mitzii; Firmiana daweishanensis; Pinguicula tejedensis, P. casperiana; Schizanthus carlomunozii; Aglaia monocaula, Aglaia nyaruensis; Primula sunhangii; Geum sunhangii; Sonerila cardamomensis; Oreocharis eriocarpa, O. wenshanensis, O. fulva, O. lacerata; Oreocharis flavovirens; Oreocharis jasminina; Oreocharis wumengensis; Petrocosmea nanchuanensis; Didymocarpus lobulatus; Allocheilos maguanensis, A. rubroglandulosus; Petrocodon luteoflorus; Petrocodon rubiginosus; Petrocodon wenshanensis; Deinostigma fasciculatum; Paraboea myriantha, P. brevipedunculata; Neptunia windleriana; Cuscuta difficilis; Siphocampylus nebularis; Eugenia flavicarpa; Dactylaena boliviensis, D. zmarztyae; Plukenetia brevistyla, Plukenetia megastyla, Plukenetia chocoensis, Plukenetia sylvestris; Ipomoea bonsai; Randia heteromera;
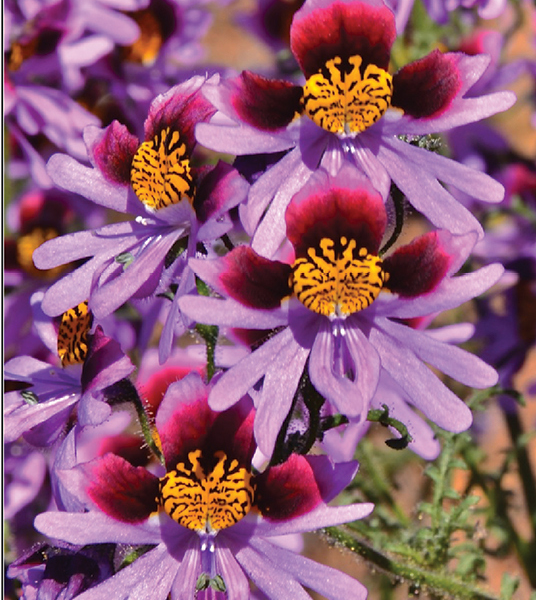
Fungi
- 1 new zygomycete: Cunninghamella guizhouensis;
- 16 new ascomycetes: Hypoxylon wujiangensis; Epicoccum endophytica; Aspergillus telluris; Arcopilus amazonicus; Muyocopron thailandica; Scheffersomyces jinghongensis, S. paraergatensis, S. anoplophorae; Dictyoarthrinium musae; Stylonectria qilianshanensis, Trichoderma gansuanum; Corylicola italica; Neoascochyta fuci, Paraconiothyrium salinum; Elaphomyces marmoratus, Elaphomyces fuscus;
- 25 new basidiomycetes: Lepiota condylospora; Lepiota cholistanensis; Rhodophana guandishanense; Ganoderma bambusicola; Amanita lacerosquamosa; Hebeloma sagarae; Fuscoporia ambigua; Junghuhnia austrosinensis, J. nandinae, J. subcollabens; Athelia termitophila; Hyphoderma fissuratum, H. mopanshanense; Colacogloea armeniacae; Phylloporia alyxiae, P. mori, P. murrayae, P. rubiacearum; Fomitiporia nubicola, F. conyana, F. murrilli; Phlebia fuscotuberculata, Ph. tomentopileata, Ph. tongxiniana; Clitopilus abprunulus;
Poriferans
- 5 new demosponges: Radiospongilla fungosa; Hyattella sulfurea, H. pedunculata, Amphimedon palmata, Phoriospongia mozambiquensis;
Cnidarians
- 2 new anthozoans: Blastopathes medusa; Anemonactis tohrui;
- 10 new hydrozoans: Zygophylax dispersa, Z. encarnae, Z. laertesi, Z. medeae, Z. niobae, Z. pseudoabietinella; Actinopluma mirifica, Schizoplumularia helicoidalis, Corhiza patula, Thamnopteros uniserius;
- 3 new myxozoans: Myxobolus vetuschicanus, Myxobolus mineirus; Sphaeromyxa scorpaena;
Flatworms
- 3 new triclads: Obama apiguara, Paraba tata, Piima ata;
- 8 new monogeneans: Microcotyle kasago; Urocleidoides digitabulum, Urocleidoides solarivaginatus, Urocleidoides falxus, Urocleidoides sapucaiensis, Urocleidoides tenuis, Urocleidoides sinus, Urocleidoides uncinus;
- 5 new trematodes: Gaevskajatrema balistes; Aenigmatrema undecimtentaculatum, A. inopinatum, A. grandiovum; Retroporomonorchis pansho;
- 1 new cestode: Polypocephalus barsami;

Mollusks
- 28 new gastropods: Caecum lightfootanum, C. draperi, C. shaskyi, C. galapagoense, C. spiculum; Amalda miriky, A. cacao; Kartvelobia sinuata, K. kinchkha, K. shishaensis, Imeretiopsis prometheus, I. gorgoleti, I. nakeralaensis, I. cameroni, I. iazoni, Caucasopsis letsurtsume, Caucasopsis olsavskyi, Caucasopsis egrisi, Caucasogeyeria gloeri, Caucasogeyeria ignidona, C. colchis, C. pseudocolchis, C. chrysomallos, Pontohoratia vinarskii, P. pichkhaiai, P. mapeli, Hausdorfenia shareula.
Nemerteans
- 2 new species: Euborlasia maycoli; Proamphiporus kaimeiae;
Annelids
- 14 new polychaetes: Gesaia csiro, Phalacrostemma timoharai; Syllis cambuk, Syllis hampirmenyatu, S. maganda; Aricidea (Acmira) filamentosa, Aricidea (Acmira) pearti, Aricidea (Acmira) bbkingi; Mauretanaspis longichaeta; Aricidea (Acmira) katzmanni, Aricidea (Acmira) pseudoassimilis; Acromegalomma sumbense, Claviramus olivager, Notaulax montiporicola;
- 2 new clitellates: Cataladrilus porquerollensis, Scherotheca portcrosana;
Bryozoans
- 1 new species: Smittoidea complexa;
Kinorhynchs
- 1 new species: Echinoderes vulcanicus;
Nematodes
- 7 new chromadoreans: Cobbionema brevispicula, Cobbionema acuminata; Rhabdochona iriomotensis, Spinitectus mirabilis; Contracaecum jorgei; Rhabdias kiri;
Tardigrades
- 1 new species: Crenubiotus revelator;
Arachnids
- 11 new mites: Cornigamasus allotritosternus; Eutegaeus parapapuaensis, Eutegaeus paralagrecai; Transeius conyzoides, Typhlodromus (Anthoseius) huangjiaensis; Cheladonta afshari, Neotrombicula tehranensis; Torrenticola pseudosiamis, T. anziensis, Monatractides sichuanensis; Mideopsis milankovici;
- 6 new harvestmen: Troglosiro dogny, T. pin, T. pseudojuberthiei, T. sharmai; Abaddon despoliator, Turonychus fadriquei;
- 2 new pseudoscorpions: Parobisium laevigatum, P.muchonggouense;
- 57 new spiders: Macrothele undata, M. arcuata, M. sanheensis; Spinirta jinyunshanensis, S. forcipata, S. sparsula, S. aurita, S. aviforma, S. quadrata, S. leigongshanensis, S. qizimeiensis, S. rugosa; Loxosceles tolantongo; Trilacuna changzi, T. hponkanrazi, T. triseta, T. zhigangi; Merizocera baoshan, M. betong, M. colombo, M. galle, M. hponkanrazi, M. kachin, M. kandy, M. mandai, M. krabi, M. kurunegala, M. lincang, M. mainling, M. nyingchi, M. peraderiya, M. phuket, M. putao, M. ranong, M. ratnapura, M. salawa, M. tak, M. tanintharyi, M. tengchong, M. thenna, M. uva, M. wenshan, M. wui, M. yala, M. yuxi; Heteroonops scapula, H. jurassicus, H. aylinalegreae, H. verruca, H. renebarbai, H. yuma, H. carlosviquezi, H. gabrielsantosi, H. solanllycarreroae, H. constanza; Ocrepeira klamt; Cybaeus daimonji;
Crustaceans
- 1 new anostracan: Phallocryptus fahimii;
- 1 new copepod: Rhinergasilus unguilongus;
- 3 new cumaceans: Cyclaspis goytacazes, Cyclaspis lilianae, Cyclaspis tesauna;
- 2 new amphipods: Carpentaria tropicalis; Pseudocrangonyx wonkimi;
- 5 new isopods: Baeticoniscus bullonorum; Fissarcturus argentinensis; Elthusa fistularia, Elthusa pseudorhombus, Elthusa uranoscopus;
- 16 new decapods: Arcotheres ocularius; Turleania rubriguttatus; Zoukaris festivus; Badistemon fulvum; Salmoneus rashedi; Caridina barakoma, C. choiseul, C. intermedia, C. maeana, C. nana, C. piokerai, C. pisuku, C. paratypus, C. poarae, C. sikipozo, C. turipi;
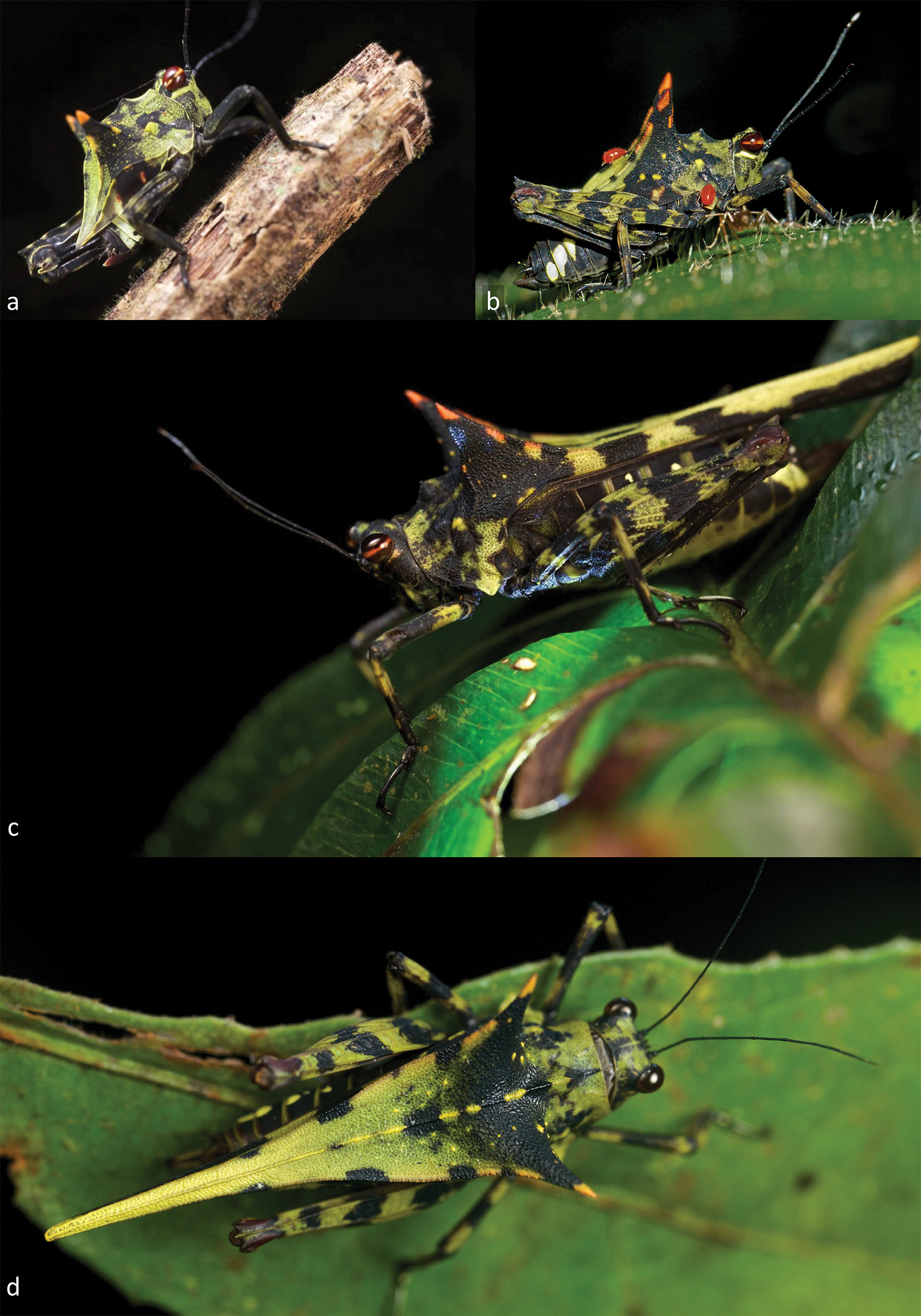
Hexapods
- 4 new collembolans: Hymenaphorura urbana, H. kalindera; Hylaeanura emiliae; Hypogastrura herrerosvelai;
- 3 new archaeognathans: Machilinus petrophilus, M. obscurus, Charimachilis rostoviensis;
- 2 new odonates: Coeliccia tongbiguan, Coeliccia yunnanensis;
- 2 new dictyopterans: Microphotina panguanensis; Rugitermes tinto;
- 9 new orthopterans: Sinohaplotropis amurensis; Tagasta mizoramensis; Boroseiyla tupeba, Caauara pinima, Capiguara trimaculata, Hyalipenna tetralineata, Tuaia panacarica; Gigagryllus omayrae; Holocerus devriesei;
- 2 new plecopterans: Sinacroneuria huzhengkuni, S. lii;
- 4 new thysanopterans: Podothrips etsukoae; Parabaliothrips betulaceae, P. brevisetosus, P. robustus;
- 87 new hemipterans: Pachymetopius anamikus, Yaontogonia unipuncta; Usanus xochipalensis, U. igualaensis; Spaltumtettix coloradus, Pseudonapo waorani, P. huanucensis, Goiattus reyesi; Acrogymnia alagoas, A. blanki, A. brevis, A. chapada, A. clypea, A. deulufeuti, A. fascia, A. krylita, A. listoni, A. pulla, D. australis, D. fernandezi, D. taegeri; Macropsis aselae; Calyria hyperochelabrys, Carineta trinidadensis, Herrera grammosticta; Pachyjassus alatus, Pachyjassus basifurcatus, Pachyjassus ranomafanensis, Petalojassus ochrescens, Phaiojassus acutus, Phaiojassus bispinosus, Phaiojassus constrictus, Phaiojassus grandis, Phaiojassus spatulatus, Phaiojassus undulatus, Phaiojassus unispinosus, Pictojassus kirindiensis, Pictojassus productus, Pictojassus tulearensis, Platyjassella ancora, Platyjassella ohahelensis, Platyjassella attenuata, Platyjassella cormorana, Platyjassella emarginata, Platyjassella immaculata, Platyjassula cyclura, Platyjassula heterofurca, Platyjassula isofurca, Platyjassula mahajangensis, Platyjassus acutus, Platyjassus asymmetricus, Platyjassus fisheri, Platyjassus griswoldi, Platyjassus harinhalai, Platyjassus irwini, Platyjassus pedistylus, Platyjassus pennyi, Platyjassus pictipennis, Platyjassus symmetricus, Platyjassus vestigius, Plerujassus brunnescens, Plexijassus caliginosus, Pseudocurtara minima, Pseudocurtara nigripicta, Pseudocurtara quadrata, Pseudocyrta hyalina, Pseudomarganana olivacea, Pseudomarganana rosea, Pulchrijassus anjozorobensis, Pulchrijassus eunsunae, Pulchrijassus pallescens, Pulchrijassus roseus, Pulchrijassus rubrilineatus, Pulchrijassus sindhuae, Pulchrijassus talatakelyensis, Pulchrijassus toamasinensis, Punctijassus circularis, Punctijassus compressus, Punctijassus ivohibensis; Betacixius latissimus, B. maguanensis; Indolipa fugongensis, I. longlingensis; Trioza turouguei; Mitjaevia shibingensis, Mitjaevia dworakowskae; Vittaliana reticulata;
- 122 new coleopterans: Anthobium formosanum, A. lackneri, A. kabateki, A. marci, A. convexior, A. auritum, A. kabaki, A. semenovi, A. inopinatum, A. bengalicum; Batriscenellus suthepensis, B. vietnamicus; Cephalocyclus iohannisgabrielis; Nogunius sokani, N. aogashimanus, N. kerri, N. fukuuzanus; Carpophilus (Myothorax) brunneus; Laccophilus leguyaderi, L. bergsteni, L. makay, L. rakouthae; Beckhoplia bicolor, B. caliginosa, B. castanea, B. dolichiocnemis, B. elkeae, B. fusca, B. gifbergensis, B. nigra, B. nigrofasciata, B. nigrosetosa, B. pallidibrunnea, B. pulchra, B. pumilla, B. setosa, B. suturalis; Strongylium purcharti; Accolabass monteithi, Alpestriscyphon bartlefrere, Al. spurgeon, Anocyphon lepus, Copiacyphon brindaleensis, C. cardinalis, C. dytikos, Pumiliocara peneparva, Ruborcara saintae, Saltuscyphon montanus, Sal. teraniaensis, Saprocyphon bithongensis, Sisyracyphon brisbanensis, S. bulburinensis, Spilotocyphon occidentalis, Sp. orientalis, Sp. zwicki, Vadumcyphon centralis, V. rugosus; Bubastes barkeri, B. deserta, B. dichroa, B. flavocaerulea, B. hasenpuschi, B. iridiventris, B. iris, B. macmillani, B. magnifica, B. michaelpowelli, B. pilbarensis, B. remota, B. viridiaurea; Leptopeltus trogloxenus; Elacatophora jambiana, E. kerinciana, E. thailandiana, E. indica; Dichotomius valoisae; Tapinomorphus angolanus, T. kudrnai, T. latipennis, T. verunkae; Zarcosia barlayi, Zarcosia bedosae, Zarcosia brunnea, Zarcosia deharvengi, Zarcosia gerstmeieri, Zarcosia ilonae, Zarcosia intermedia, Zarcosia lemairei, Zarcosia schawalleri, Zarcosia sinuata, Zarcosia spinifemur Zarcosia srilankaensis, Zarcosia sumatrensis, Zarcosia weigeli; Australonotha monteithi; Xylodromus schweiki; Ancystrocerus lueliangi, A. philippinus; Blakealtica fusca; Exocelina athesphatos, E. tsinga; Ivalia antennata, I. caligulata, Cangshanaltica mindanaoensis, C. luzonica; Nephus apolonia; Agabus anguluverpus, Agabus austellus, Agabus riberae, Agabus agulhas; Metapogonia snizeki; Anchylorhynchus chrysomeloides, Anchylorhynchus goiano, Anchylorhynchus imitator, Anchylorhynchus latipes, Anchylorhynchus multisquamis, Anchylorhynchus rectus; Liodessus altoperuensis, Liodessus caxamarca; Apatrobus hekosanus; Ceratanisus diyarbakiricus; Dilychnia dumasi;
- 3 new neuropterans: Austrochrysa lihongyui, Austrochrysa angusta; Nusalala peruana;
- 68 new hymenopterans: Trieces confusus; Synergus aurofacies; Odontomutilla sairandhriensis, O. fletcheri; Aivalykus microaciculatus; Cestrus itatiensis; Alastor (A.) brevicornis, Alastor (A.) carens, Alastor (A.) gestroides, Alastor (A.) moody, Alastor (A.) namibiensis, Alastor (A.) parilis, Alastor (A.) sabrinae, Alastor (A.) temperatus, Alastor (Alastorellus) planipunctus; Aulacus davidi, A. shizukii; Gynecaptera albicrus, Macroocula madinaensis; Dicamptus curvus; Lymaenon funiculus, L. ledongus, L. longitus, L. naiquanlini, L. radiculus, L. zhui; Megischus sp., Foenatopus spp., Parastephanellus spp.; Microstenus rufithorax; Norbanus persicus, Stenomalina delvarei; Aleiodes andinus, A. angustus, A. asenjoi, A. bahiensis, A. barrosi, A. brevicarina, A. coariensis, A. goiasensis, A. gonodontivorus, A. hyalinus, A. inga, A. joaquimi, A. lidiae, A. mabelae, A. maculosus, A. ovatus, A. santarosensis, A. taurus; Periclistus orientalis; Pheidole antsahabe, Pheidole atsirakambiaty, Pheidole clara, Pheidole flammea, Pheidole flavodepressa, Pheidole mantadioflava, Pheidole maro, Pheidole ovalinoda, Pheidole similis, Pheidole tenebrovulgaris, Pheidole uranus, Pheidole voreios, Pheidole zirafy; Coccobius islandicus; Sphinctus pereponicus;
- 117 new dipterans: Sarcophaga (Hoa) membranijuxta; Tanap cinar; Winnertzia acutistylus, W. angustistylus, W. arctostylus, W. bicolor, W. brachytarsus, W. dentata, W. egregia, W. ekdalensis, W. fraxinophila, W. grytsjoenensis, W. hamatula, W. hemisphaerica, W. imbecilla, W. incisa, W. inornata, W. lapponica, W. lobata, W. longicoxa, W. normalis, W. oelandica, W. ombergensis, W. parvidens, W. pilosistylus, W. pratensis, W. pustulatula, W. quercinophila, W. rickebasta, W. ruliki, W. serri, W. setosa, W. silvestris, W. smalandensis, W. sundini, W. tumidoides, W. upplandensis, W. panguan, W. warraensis; Coniceromyia spp.; Condylostylus comorensis, C. friedmani, C. gavryushini, C. kaplini, C. madagascarensis; Cheilosia candida, Paragus thracus, Psilota aegeae; Macropelopia pergrandis; L. anaphlecter, L. apoecus, L. asilomar, L. bitumineus, L. canningsi, L. condylophorus, L. esau, L. karli, L. nelsoni, L. odontotus, L. sierra, L. tumulicola, L. wilcoxi; Dasythereva penai, D. patagonia; Tephritis kutuki; Epiphragma amamiense; Atelestus turcicus, Atelestus ibericus; Massalongia nakamuratetsui; Colobaea deemingi; Oxysarcodexia alectoris, O. angulosa, O. ariozanoi, O. graminifolia, O. maiae, O. rimata; Schizomyia maesae;
- 55 new trichopterans: Cernotina longa; Agmina tuberosa, A. semiovale, A. rocheta, A. tenuisa, A. multidentata, A. cornuta, A. sagittata, A. circulata, A. digitata, A. longispina, A. magnahamata, A. longicordata, A. campanula, A. semicampanula, A. cunicula, A. cerritula, A. monstrosa, A. rectangulata, A. chela, A. piscaria, A. amplexa, A. caraffa,, A. rostrata, A. dathioensis, A. rougensis, A. viklundi, A. lata, A. falx, A. guttula, A. amieuensis, A. spina, A. complexa, A. dognyensis, A. mana, A. anterohamata, A. curvatacua, A. recurvata, A. taoensis, A. triangulata, A. bleuensis, A. touhoensis, A. wardi, A. parallela, A. christinae, A. brevis, A. ninguana, A. scopula; Ecnomus petchanaae, E. boonsawaengae, Polyplectropus proukaewi, Psychomyia srichanai; Machairocentron chorotegae, M. eugeniarguedasae, M. kalinae;
- 84 new lepidopterans: Dichomeris fascialobella, D. flavimaculata, D. infundibularis, D. lancea, D. longivalvata, D. marginalis, D. myriaodontosa, D. ochriella, D. quadratella, D. sclerospinula, D. spinosella, D. unilobata, Helcystogramma albimacularis; Abantiades cephalocorvus, Abantiades tembyi; Ypsolopha admirandella; Yelenka calciferella; Panca puri, Panca xavante; Lophocnema mackeyi, Oligophlebia insurgia, Micrecia capillaria, Micrecia kuukuyau, Micrecia hawkei, Lamellisphecia sanguinea, Specodoptera hiltoni, Aegerosphecia rufea, Melittia flanneryi, Melittia pyroptella, Archaeosesia milleri, Nokona kungkay, Nokona bipora, Pseudosesia muyuae, Pseudosesia sumoensis, Pseudosesia sepikensis, Paranthrenella terminalia, Paranthrenella brandti, Paranthrenella lelatensis; Epicrocis datianensis, E. maculata, E. flabelloformis; Leucinodella banthaensis; Exoasota pursatensis; Metallolophia taleensis; Meleonoma anisodonta, M. catenata, M. dilativalva, M. dorsibacilliformis, M. lanceolata, M. longaedeaga, M. longiprocessa, M. lunulata, M. papillata, M. parvissima, M. rugulosa, M. tenuiuncata, M. tianmushana, M. triacantha, M. ventrospinosa; Stathmopoda atrifusca, S. cellifaria, S. cornuta, S. digitiprocessa, S. dolichantha, S. falsistimulata, S. guangxiensis, S. ingena, S. liberata, S. purpurata, S. serrifasciaria, S. similatridorsalis, S. spinicornuta; Eidophasia assmanni; Epiparbattia multispinalis; Sacada dzonguensis, S. umtasorensis; Megacraspedus cottiensis; Nagiella bispina; Catoptria samnitica; Nokona vietnamica, Nokona cucphuongae; Phragmataecia effendii; Pseudodebis nakamurai, P. pieti;
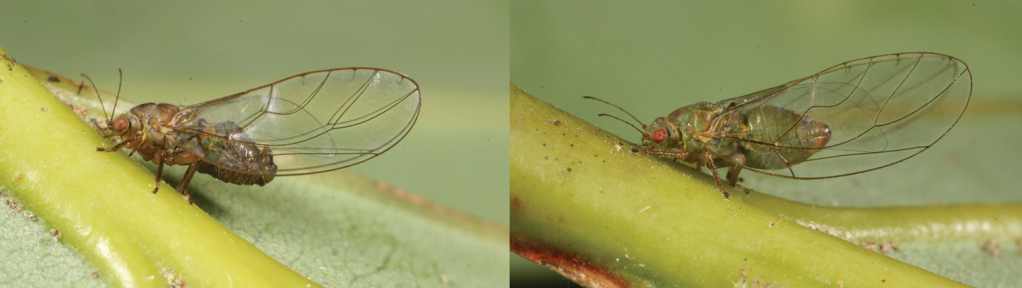
Echinoderms
- 4 new ophiuroids: Astrochlamys timoharai, Ophiosteira lissopatella, Ophiophyllum umbonatum, Soliophis bakeri;
- 1 new holothuroid: Elpidia soyoae;
Agnathans
- 1 new myxinid: Eptatretus albiderma;
Actinopterygians
- 1 new anabantiform: Parosphromenus barbarae;
- 1 new anguilliform: Xyrias anjaalai;
- 2 new characiforms: Bryconops sp.; Priocharax varii;
- 2 new cypriniforms: Paracobitis salihae; Rasbora adisi;
- 1 new gobiiform: Corcyrogobius pulcher;
- 3 new perciforms: Menticirrhus sp.; Upeneus floros; Etheostoma faulkneri;
- 1 new siluriform: Aspidoras azaghal;
- 1 new tetraodontiform: Canthigaster aziz;
Amphibians
- 1 new caudate: Bolitoglossa awajun;
- 12 new anurans: Platymantis sp.; Leptobrachella neangi; Pristimantis sp.; Pristimantis chamezensis; Adenomera spp.; Xenorhina salawati, X. waigeo;
Reptiles
- 10 new squamates: Hemiphyllodactylus nahangensis; Cyrtodactylus houaphanensis, Cyrtodactylus ngoiensis; Cyrtodactylus sp.; Cyrtodactylus amphipetraeus; Cnemaspis rishivalleyensis, Hemidactylus rishivalleyensis; Atheris hetfieldi; Acanthosaura liui; Dipsas bothropoides;
– – –
*
This work is licensed under a Creative Commons Attribution 4.0 International License.