Previous research (2016-2023)
Summary of previous research (2016-2023)
Previous research focused on:
- identifying which of the many natural enemies that attack flaxleaf fleabane in the native range are the most appropriate candidate biological control agents for this weed,
- undertaking comprehensive testing to demonstrate that they do not pose a threat to non-target plants and,
- if approved by the authorities, releasing the agents across the range of the weed in Australia.
This research was undertaken as part of two projects led by AgriFutures Australia and primarily supported by funding from the Australian Government Department of Agriculture, Water and the Environment as part of its Rural R&D for Profit programme rounds 2 and 4:
- Round 2: Biocontrol solutions for sustainable management of weed impacts to agricultural profitability (2016-2020)
- Round 4: Underpinning agricultural productivity and biosecurity by weed biological control (2019-2023)
More information can be found here: https://agrifutures.com.au/wp-content/uploads/2022/04/AgriFutures_Weeds-newsletter_Autumn-2022.pdf
The overlapping Round 2 and 4 projects were designed to complement each other and included the following main activities:
- Define management goals
- what stakeholders would like biological control to achieve.
- Undertake literature searches
- to gather information on the target weed, its distribution and its known natural enemies.
- Nominate flaxleaf fleabane as a target for biological control
- In Australia, a weed must be endorsed as a target for biological control by the national Environment and Invasives Committee before permission to release a biological control agent is sought.
- Identify appropriate region(s) to source candidate biological control agents
- Bioclimatic modelling; the climate of the native and invaded ranges of the target weed are characterised and compared using a distribution modelling tool. By comparing meteorological data from the different regions, specific area(s) of the native range can be identified where potentially best climatically suited candidate agents will be found.
- Genetic study; molecular characterisation of the target weed is used to determine the weed genetic structure which helps identify the area(s) of the native range where the weed originates from.
- Conduct native range surveys to identify candidate agents
- Some level of quantification of the damage caused by the natural enemies of interest is performed while surveying in order to assist selection of the most promising agents for further investigations.
- Test the host-specificity of promising candidate agents
- Host-specificity testing is necessary to determine the potential range of plants (hosts) which will be attacked by the candidate agent in Australia. Testing methods are always tailored to the particular agent and seek to understand the potential range of plants that would be accepted by the agent after its release in the field. Testing follows a centrifugal phylogenetic approach that concentrates on closely related plant species to the target weed.
- Prepare and submit applications for release of suitable candidate agent(s) to the relevant authorities, provided testing results demonstrate that non-target plants in Australia would not be at risk.
- Establish monitoring plots and gather baseline vegetation data prior to the release of agents.
- Once permission to release an agent is obtained, mass-rear and release the agent into the field across key areas to identify the most effective release method that leads to establishment of the agent.
- Partner with relevant stakeholder networks (agriculture) to facilitate large-scale releases of the agent across the range of the weed in Australia. Wherever possible, measure the impact of the agent on the weed populations and flow-on effects on vegetation to quantify benefits of the project.
Previous work also projected climatic suitability modelling using CLIMEX, and identified significant areas of Queensland, New South Wales, South Australia and Western Australia as either optimal or suitable environments for growth of flaxleaf fleabane. In the absence of further management, the model predicts that the distribution and abundance of this weed is likely to increase in these states.
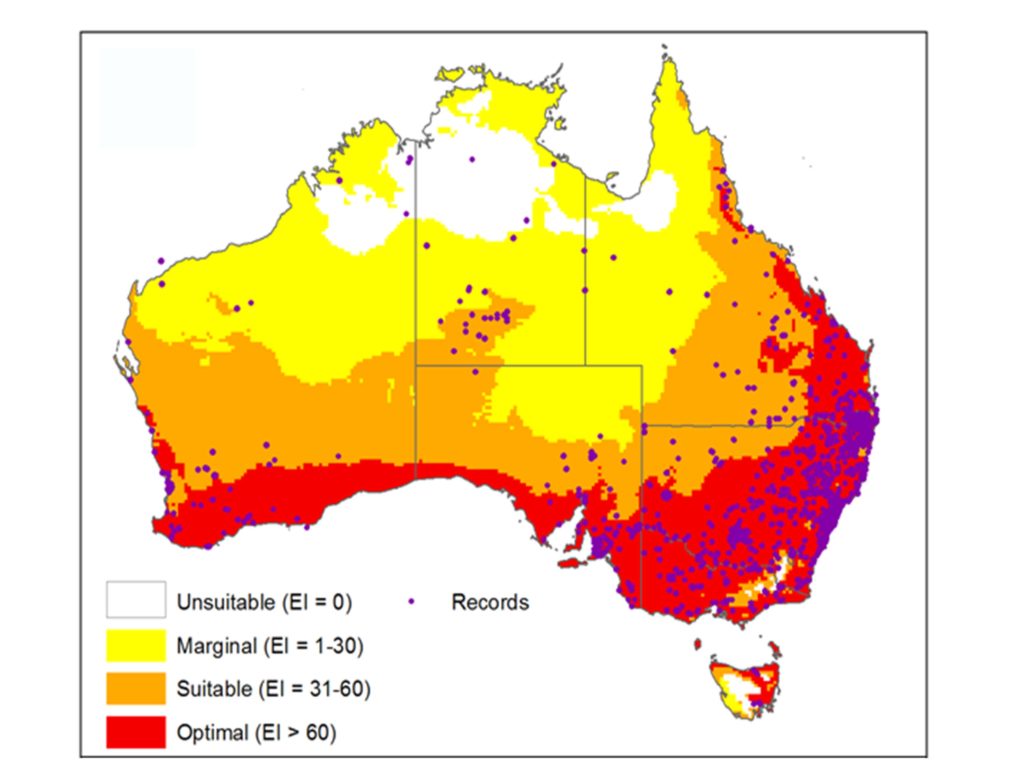
Projected climatic suitability model and observed distribution for flaxleaf fleabane in Australia. Current records of flaxleaf fleabane are indicated by the purple dots. Areas in red have been identified as having the optimal climate for flaxleaf fleabane. Reproduced from Scott JK, Yeoh PB, Michael PJ (2016) Methods to select areas to survey for biological control agents: An example based on growth in relation to temperature and distribution of the weed Conyza bonariensis. Biological Control, 97: 21-30.
Achievements of Rural R&D for Profit round 2 project: Biocontrol solutions for sustainable management of weed impacts to agricultural profitability (2016-2020)
Undertook a literature review on taxonomy and distribution of African boxthorn and known natural enemies of the weed in the introduced and native ranges
Taxonomy
Conyza is mostly a New World genus in the tribe Conyzinae of the family Asteraceae (or Compositae), the largest of all plant families (c. 25,000 species worldwide). There are three main species of Conyza in Australia – Conyza bonariensis (flaxleaf fleabane), Conyza canadensis (Canadian fleabane) and Conyza sumatrensis (tall fleabane). Conyza bonariensis is the most widespread, occurring in all states and territories, followed by C. sumatrensis and C. canadensis. There are four other Conyza species present in Australia, but their distribution is limited ‒ C. bilbaoana, C. parva, and C. primulifolia (PlantNET 2020). It is noteworthy that the curator of the Australian National Herbarium has doubts as to the circumscription and recognition of Conyza bilbaoana in Australia.
Conyza bonariensis has the narrowest leaves at the rosette stage when compared to other Conyza species (Thébaud and Abbott 1995). It has a more compact stature, with many short branches and bearing large capitula, while C. canadensis is essentially a single-stemmed taxon with few long branches and with small and elongated capitula. Conyza bonariensis is a genetic allopolyloid (arose through hybridisation; hexaploid (2n=54)) and strictly semelparous (has a single reproductive episode before death) (Thébaud and Abbott 1995). It is self-compatible and seems not to be pollinated by insects (Thébaud et al. 1996).
Evidence of genetic variation in several morphological traits of C. bonariensis was found in a common garden experiment conducted by Thébaud and Abbott (1995) in Europe. Hybrids of unknown fecundity, originating from crosses between C. bonariensis and C. canadensis and between C. bonariensis and C. sumatrensis, have been reported in Europe (McClintock and Marshall 1988). However, a subsequent isozyme survey of five Conyza species in Europe failed to find intermediate individuals (Thébaud and Abbott 1995). Zelaya et al. (2007) highlighted that loss of vigour in Conyza hybrids is apparently a common trait. They speculated that ploidy differences may be a significant barrier determining successful hybridisation between Conyza spp., e.g. more compatible hybrids would be expected from crosses between allopolyploids such as C. sumatrensis and C. bonariensis compared to crosses with the diploid (2n = 18) C. canadensis. The extent of genetic diversity in C. bonariensis and existence of hybrids in Australia are unknown.
Distribution
Conyza bonariensis is present in all states of Australia, occurring predominantly in temperate and Mediterranean coastal regions, and with restricted distributions in semi-arid to arid central regions (GBIF.org 2nd November 2018). It is native to warm temperate South America, (Michael 1977). It is considered widespread in Argentina, Uruguay, Paraguay and Brazil, and has been recorded in coffee plantations in Colombia and Venezuela (Mangolin et al. 2012).
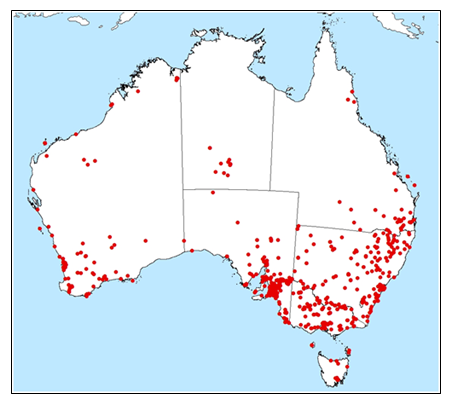
Current distribution Conyza bonariensis (flaxleaf fleabane) in Australia (GBIF.org 24th July 2018).
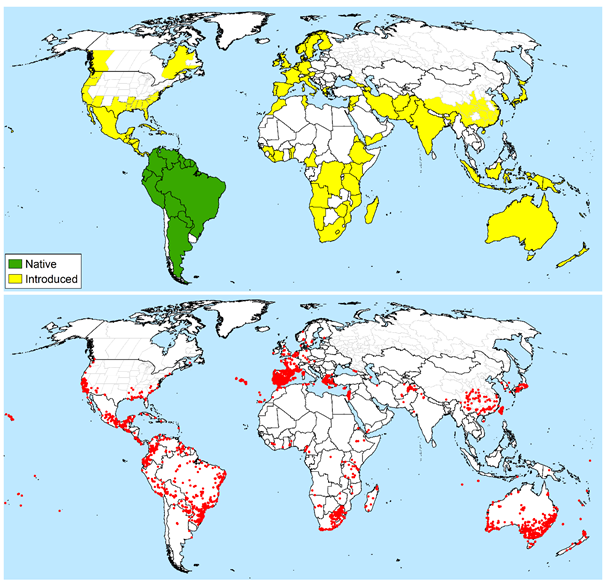
Global distribution of Conyza bonariensis. (a) Administrative level distribution assigned to native or introduced status at the national or provincial level (when not widely distributed across a whole country), modified from Scott et al. (2016) and updated to reflect (b) point distribution data records from GBIF.org (2nd November 2018).
Defined goals for management of Flaxleaf fleabane
Between May and July 2017, the online platform SurveyGizmo was used to survey key stakeholders in the grains industry affected by C. bonariensis and Sonchus oleraceus, about the impacts and desired management goals for these weeds. Both weeds were included in the same survey because they are problems in the similar areas and land uses. for the A total of 60 responses were received; 51 complete (85% answered all questions), and 9 partial responses. Respondents identified as either agricultural landholders (55.2%) or agricultural land manager, agronomist or extension officer (48.3%).
Respondents indicated that most of the agricultural impacts listed for these weeds were relevant as four of the six impact statements received > 50% response (respondents were able to select more than one impact statement). The leading impact statement was that these weeds are “difficult to control” (79.2%) followed by “reduces stored water supplies in fallow” (66%).
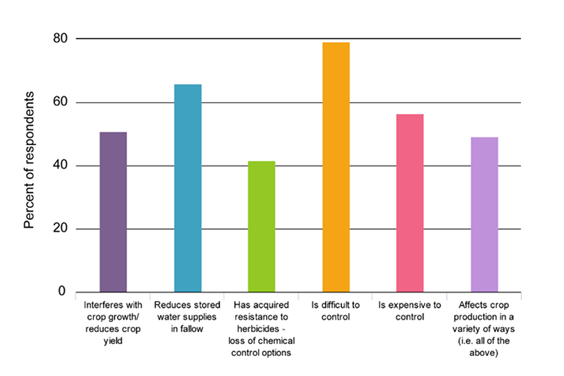
The percent of respondents who selected the various impact options for Conyza bonariensis (flaxleaf fleabane) and Sonchus oleraceus (sowthistle) provided in the survey.
Eighty-seven percent of respondents indicated that they felt that a new tool such as biocontrol would be useful in the management of fleabane and sowthistle (11.1% neutral, 1.9% disagreed). Fallow, roadsides and fence lines were considered by most respondents to be areas in which biocontrol could contribute to management as they received >50% responses.
The respondents selected the following top four management objectives to which biocontrol needs to contribute to be considered successful were:
- Decrease weed management cost and effort,
- Reduce herbicide inputs required,
- Decrease the need for follow-up control, and
- Reduce the occurrence of new infestations.
A review on the costs of weed to the grain industry (Llewellyn et al. 2016), stated that C. bonariensis and S. oleraceus are high impact summer fallow weeds responsible for revenue losses of $43.2 and $4.9 million per year respectively. These two cropping weeds are also responsible for $3.6 (C. bonariensis) and $1.3 (S. oleraceus) million annually in additional herbicide costs due to the development of herbicide resistance in populations these species. The economic and chemical inputs required to control these weeds are set to increase if herbicide resistance increases in frequency and distribution. Based on the outputs of the management objectives’ survey, these fiscal impacts serve as a baseline against which the economic and management success of any introduced biocontrol agents can be judged in the future.
Nominated Flaxleaf fleabane as a biocontrol candidate
The project prepared the documentation to support the nomination of C. bonariensis as a target for biocontrol. The documentation was submitted to the IPAC (now EIC), by the Queensland Department of Agriculture and Fisheries in May 2017 and endorsed by the Committee in November 2017 (Rafter and Morin 2017).
Conducted genetic analysis on samples of Flaxleaf fleabane from different regions in Australia and the native range
A total of 375 putative individuals of C. bonariensis: 239 individuals from 18 sites in Australia, 60 individuals from 8 sites in Brazil, 9 individuals from 1 site in Argentina and 67 herbarium specimens from the Americas were analysed with Diversity Arrays Technology (DArT)seq. DArTseq Single Nucleotide Polymorphism (SNP) data for these samples consisted of 100,629 loci which was reduced to 18,110 loci following filtering of DArT parameters.
Due to challenges with collecting fresh specimens in the native range for this study, the dataset comprised samples from herbarium specimens. This is a newly emerging approach to supplement sampling in cases where it is difficult to collect fresh material from the field. It is noteworthy that 50% of the 137 samples from putative C. bonariensis herbarium specimens processed were successfully extracted and sequenced.
Phylogenetic trees using the whole data set were generated using both Geneious (https://www.geneious.com/geneious/) and SplitsTree (http://splitstree.org/) softwares. These trees indicated the presence of two groups of samples, with no evidence of hybridisation between them. A further phylogenetic tree was generated in Geneious using a reduced dataset comprising three randomly selected plants from each Australian sites where fresh material was collected and all other samples in the dataset. We also excluded from this analysis the six West Mackay samples previously found to cluster with Group 1, as this indicated that sampling at this site was performed on more than one Conyza species.
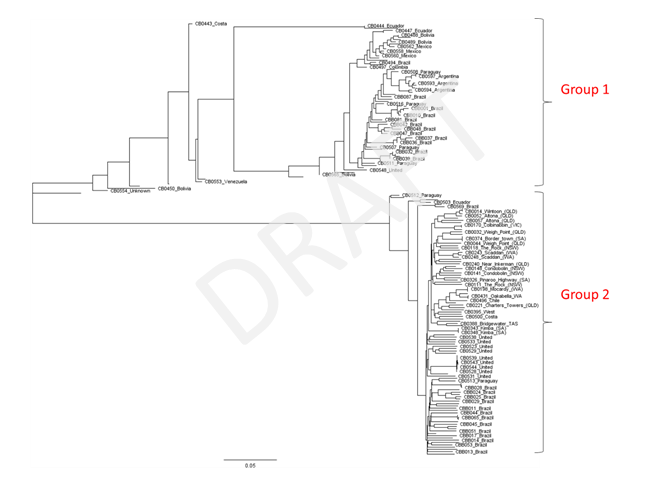
Phylogenetic tree of a reduced DArTseq dataset of putative Conyza bonariensis samples produced using Geneious.
Group 1 comprised samples primarily from South and Central America, with just one sample from the United States (close-up figure of Group 1 available on request). Group 2 comprised all Australian samples and the remaining 18 United States samples as well as samples from Brazil and a small number of other samples from South and Central America. Group 2 thus corresponded to C. bonariensis, as it is known in Australia. In contrast, Group 1 indicated that several of the herbarium samples included in the analysis had been misidentified as C. bonariensis. Furthermore, the analysis revealed that not all fresh samples collected from putative C. bonariensis plants at the same sites in Brazil were in the same group, indicating that plants from more than one Conyza species were sampled by our collaborators. This analysis indicated that C. bonariensis collected in Australia were more closely related to herbarium samples from Chile, Costa Rica and Guatemala, followed by a sample from Bahamas and a subset of samples from the United States.
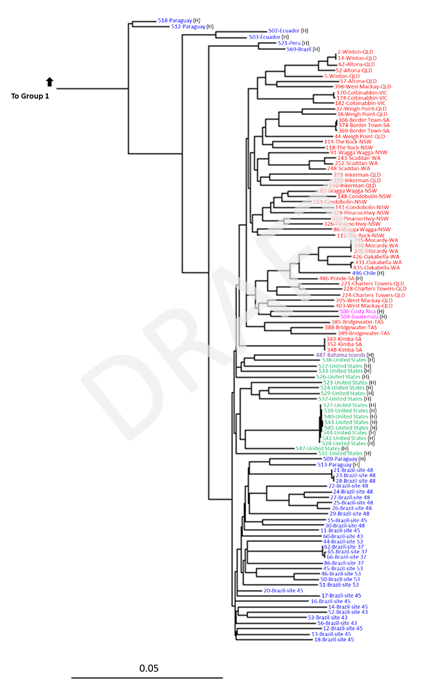
Close-up of Group 2 from the phylogenetic tree presented in Fig. 4, which has been re-labelled. Samples names are comprised of ID number and country (site name and state indicated for Australian samples, and site number indicated for field-collected samples from Brazil). Colour-coding: blue = South America, pink = Central America, purple = Caribbean, green = North America (USA); red = Australia. Samples that originate from herbarium specimens are indicated by H.
The Bayesian clustering program STRUCTURE and the Poppr R package were subsequently used to assess the extent of population genetic structure among samples in Group 2. Since this group was dominated by Australian samples, we undertook a different approach that firstly determined how many genetic clusters (K) occurred within the invaded Australian range without any prior knowledge of population affinities. The analysis showed that two genetic clusters were most likely present within Australia and that two populations from Western Australia (Mocardy; H and Oakabella; O) were distinct to the other Australian populations. Assignment of native range samples revealed that they were all admixed and that some samples in the United States had higher levels of admixture with the genetic cluster that characterised the two different Western Australian populations. The levels of admixture in native range samples makes it challenging to determine where Australian samples have originated from, although there is a suggestion that the two Western Australian populations have come from the United States.
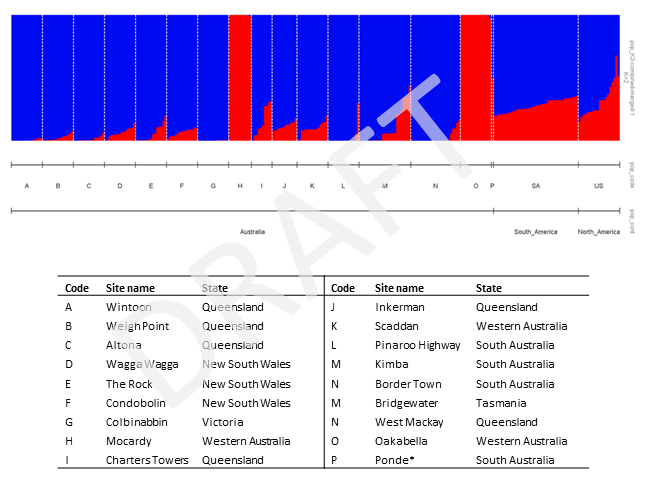
Bar plot representation of results from the STRUCTURE analysis of samples from the Group 2 only, which represents Conyza bonariensis as it is known in Australia. Genotypes are best described by two genetic clusters (blue and red).
Undertook bioclimatic modelling to identify optimal locations and conduct native range surveys and host-specificity tests for potential biocontrol agent(s) and imported at least one potential agent in quarantine
Bioclimatic modelling
The approaches taken for the bioclimatic modelling of C. bonariensis were like those used for Lycium ferocissimum; Match Climates and Compare Locations models developed using the CLIMEX package (Kriticos et al. in preparation). An experiment was also conducted to measure the growth rate of C. bonariensis at different temperatures.
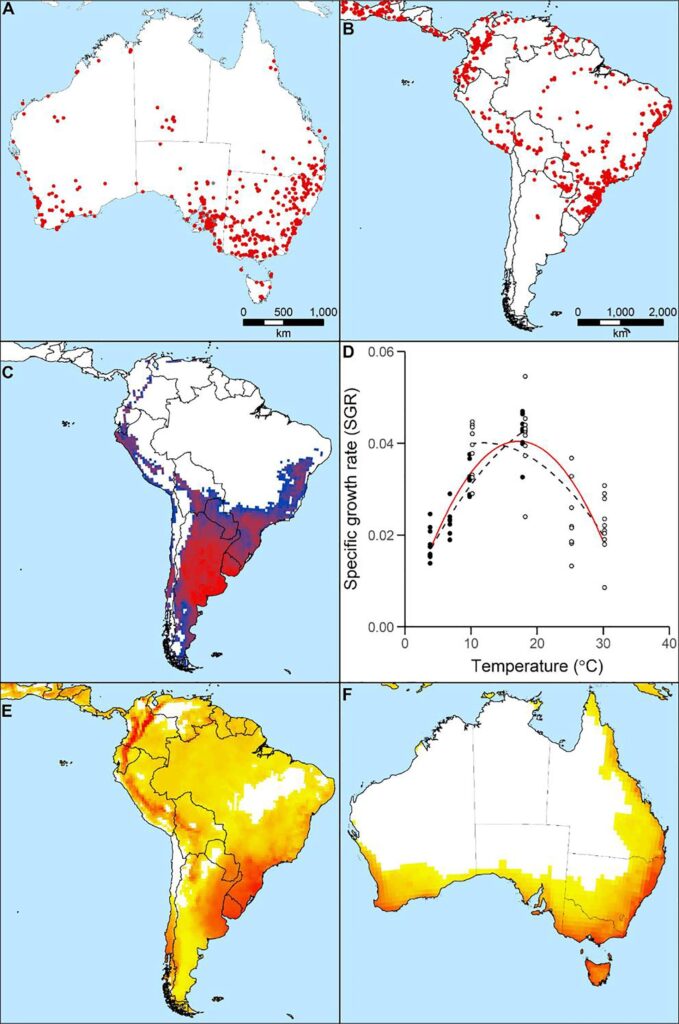
Conyza bonariensis’ known distribution in (A) Australia and (B) the native range of South America. Preserved and living specimen records (red points) projected atop observational records (grey points) (GBIF.org 2nd November 2018). (C) CLIMEX Match Climates model, as projected for South America. (D) Temperature response curve. Filled and unfilled points indicate two separate experimental runs, with associated fitted polynomial models as dashed black lines. Final fitted polynomial model incorporating both datasets shown as a red line. Projected climatic suitability from the CLIMEX Compare Locations model projected for South America (E) and Australia (F) (Kriticos et al. 2021)
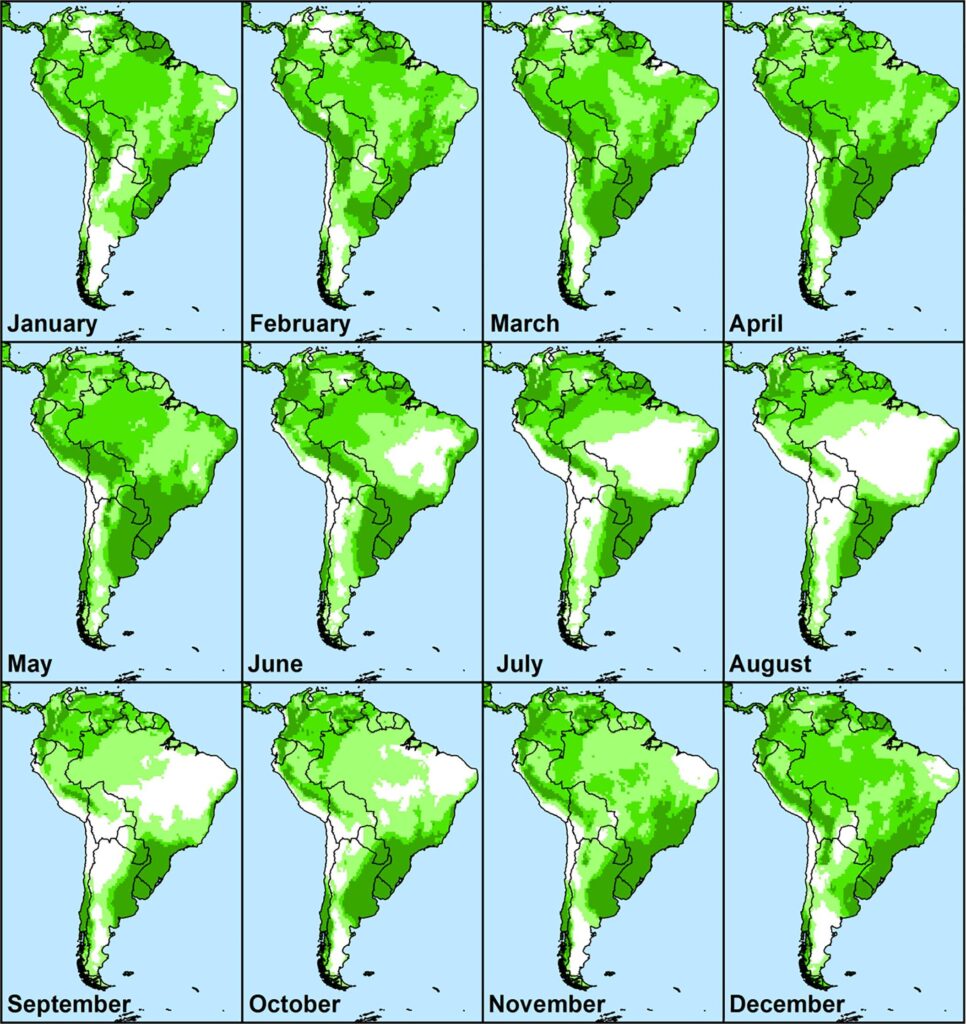
Monthly Growth Index values in native range for guiding when and where to survey for natural enemies on Conyza bonariensis. Values are averaged across five years from 2012 to 2017. Increased intensity of green colour indicates higher climatic suitability (Kriticos et al. 2021)
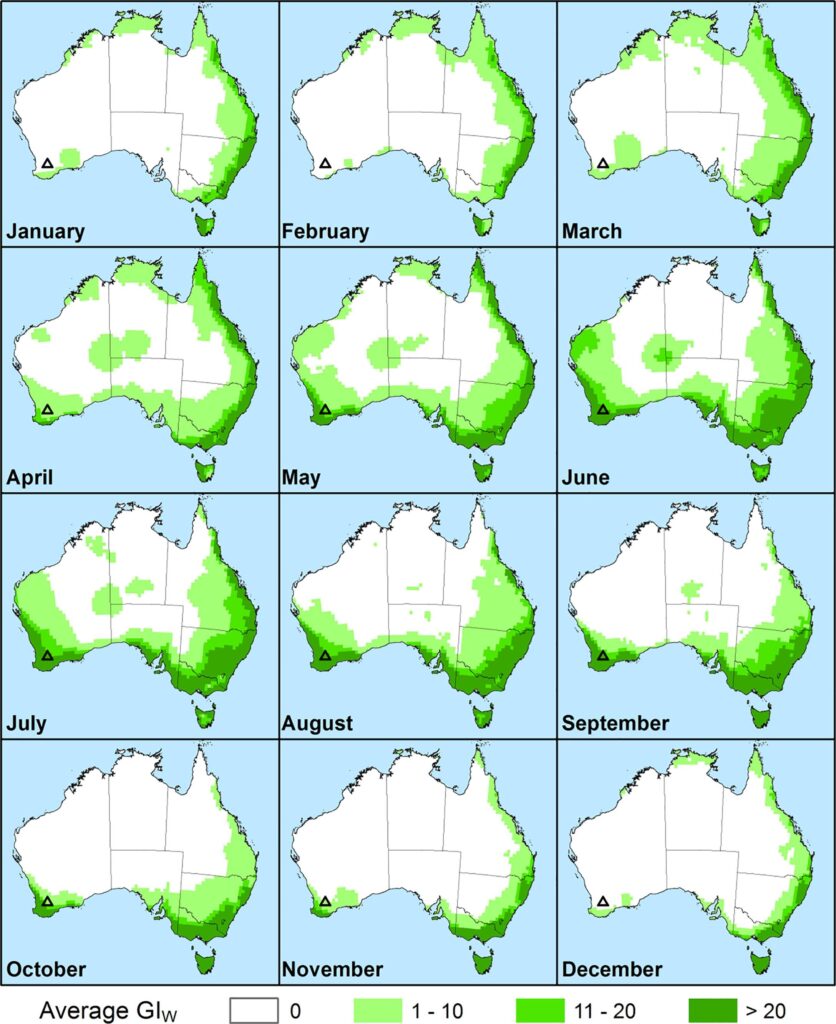
Monthly Growth Index values in Australia for guiding when and where to release biocontrol agents on Conyza bonariensis. Values are averaged across five years from 2012 to 2017. Agents would only be deployed in areas in which the Ecoclimatic Index was most positive, indicating potential for year-round survival (Kriticos et al. 2021)
Native range surveys
Pathogens
Surveys for pathogens on Conyza species were performed in different departments of Colombia between November 2017 and May 2019. Surveys concentrated on Colombia because several rust fungi had only been recorded from Conyza sp. in this country in South America. A total of 136 Conyza sp. samples with disease symptoms were collected across all surveys during that period. High morphological diversity in Conyza species was observed in the field and it was difficult to categorically identify plants to species level. Consequently, plant tissue was collected from representative specimens and DNA extracted and sequenced to obtain a more reliable identification. Although some collections were made on C. bonariensis, most were made on C. sumatrensis. Collaborators in Colombia had the opportunity to present a few of their Conyza sp. herbarium specimens to Dr John Pruski was visiting the plant herbarium in Medellín during winter 2019. Dr Pruski is an expert taxonomist on the Asteraceae family based at the Missouri Botanical Garden in St. Louis, MO, USA. He identified each of the five herbarium specimens presented to him as either C. sumatrensis var. leiotheca or C. sumatrensis var. sumatrensis.
Disease symptoms observed during the field surveys ranged from chlorotic or necrotic lesions on leaves or stems, mildew and rust. Several fungi were recovered from the symptoms, including Basidiophora entospora, Oidium sp., Cercosporella sp., Septoria sp., Wentiomyces sp., Diaporthe sp. (confirmed with sequencing) and Alternaria sp. Two rust fungi were also found: a species with aecia on Conyza sp. and the microcyclic species identified as Puccinia cnici-oleracei. The former rust fungus was later confirmed in a cross-inoculation study to be an heteroecious, macrocyclic rust species, with Cyperus sp. as its main host and Conyza sp., thus, unsuitable for biocontrol.
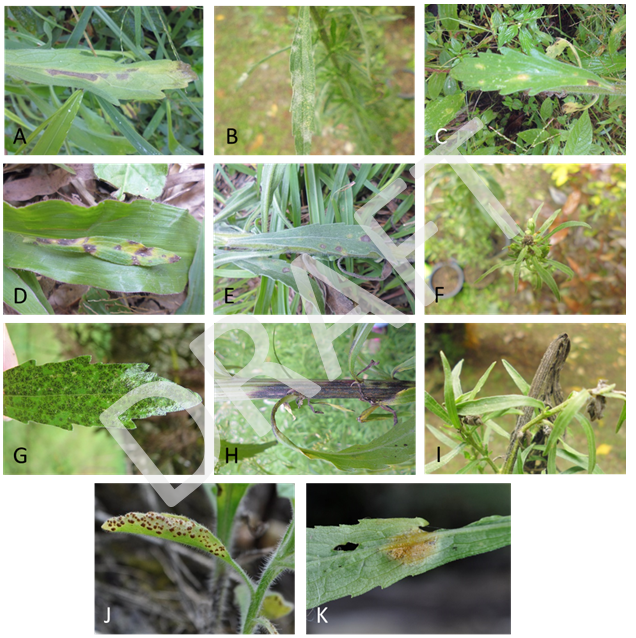
Some of the fungi associated with symptoms observed on Conyza sp. during field surveys in Colombia. A: Basidiophora entospora, B: Oidium sp., C. Cercosporella sp., D. Cercosporoid fungus E: Septoria sp., F: Periconia sp., G: Wentiomyces sp., H: Diaporthe sp., I. Alternaria sp., J: Puccinia cnici-oleracei, K: Puccinia cyperi (aecium). Photos Universidad Nacional de Colombia, Medellín.
Insects
Surveys for insects have been undertaken on Conyza species in Argentina, Paraguay, Brazil, Colombia and the southern USA (Louisiana, Texas, Alabama) between November 2017 and February 2020. These surveys have identified herbivorous insects from some 14 families (Agromyzidae; Cecidomyiidae; Cerambycidae; Curculionidae; Lixidae; Membracidae; Miridae; Mordellidae; Pseudococcidae; Pterophoridae; Tephritidae; Tingidae; Tortricidae; Coccidae) associated with Conyza spp. in the native range. Some 35 species/morphospecies have been identified to date, and among these the most promising are two species of gall flies (Trupanea bonariensis that forms stem galls; an unindentified fly that forms leaf-blister galls), a weevil (Lixus sp., a stem- and root-feeder) and two species of moths (both unidentified, both leaf rollers). These species have all been recorded in Argentina and Brazil, in areas of strong bioclimatic similarities to where C. bonariensis occurs as a weed in Australia. Several of the insect species in the native range are new to science and are in the process of being identified by taxonomic experts.
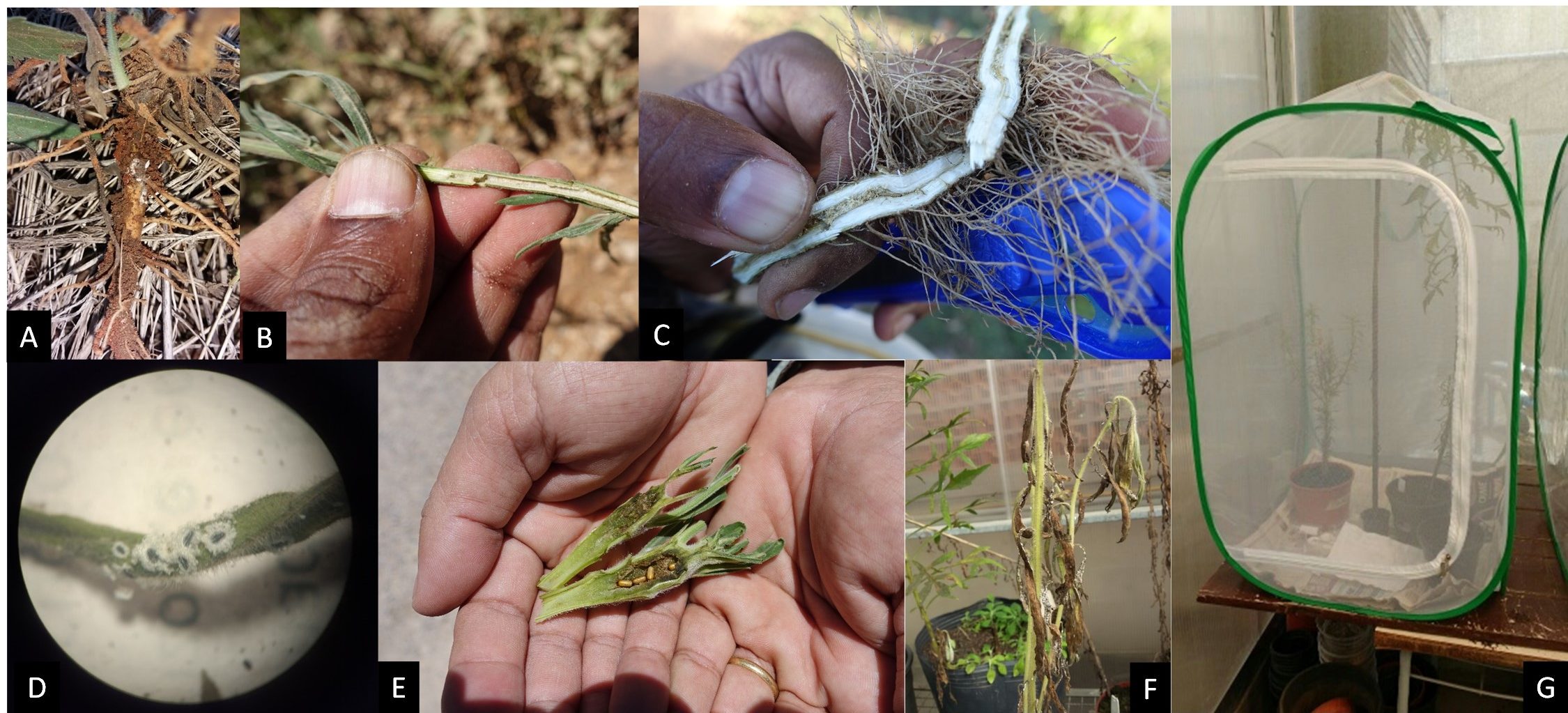
Herbivorous insects recorded on Conyza sp. as part of surveys; A. Root-feeding scale insect, B. Stem borer; C. Root-feeding beetle; D. Leaf-feeding scale insect; E. Stem galling fly; F. Impact of scale insect (A) of Conyza sp.; G. laboratory colony of stem galling fly (E) in Brazil
Importation of candidate agents into quarantine
Pathogens
Puccinia cnici-oleracei (ex. Conyza) was deemed the most promising candidate biocontrol agent to investigate. The necessary export permit was obtained from the relevant Colombian authorities. Concurrently, a permit to import the fungus in the CSIRO quarantine facility in Canberra was obtained from DAWE. The fungus was imported on 20 November 2018. A single-telium isolate was generated from the material imported and a culture established in quarantine.
Insects
Trupanea bonariensis, the stem gall forming tephritid fly, was imported in a quarantine facility in Australia in November 2019 and February 2020. Colonies of this fly were in the process of being established as a precursor to detailed biological studies and host-specificity testing. This work was undertaken as part of the following Rural R&D for Profit project (Agrifutures Australia Project number: PRJ-12377).
Host-specificity tests for candidate biocontrol agents
The proposed list of non-target species for host-specificity testing of candidate biocontrol agents for C. bonariensis was submitted to DAWE in December 2018 for posting on their website for feedback (Hunter et al. 2018).
Pathogens
A cross-inoculation experiment was performed in Colombia to obtain an initial indication of the specificity of P. cnici-oleracei. The experiment included accessions of P. cnici-oleracei recovered from Conyza sp. and Emilia sonchifolia, which were growing in proximity at the same site. Both Conyza (=Erigeron) and Emilia species are recorded as hosts of P. cnici-oleracei, although the fungus on Emilia is also referred to as Puccinia emiliae by some authors (Farr and Rossman 2020). Results demonstrated that the rust accessions were capable of only infecting the host species they originated from. Plants of Conyza sp., but not of E. sonchifolia, developed disease symptoms when exposed to rust-infect Conyza sp., and vice versa. Based on these results, we concluded that the fungus from Conyza sp. was probably highly specific and thus decided to refer to it as P. cnici-oleracei (ex. Conyza).
Comprehensive host-specificity testing in quarantine began in February 2019 and only one experiment remained to be performed. The test list comprises a total of 50 closely related, non-target species in the subfamily Asteroideae of the family Asteraceae that occur in Australia (ornamental, weed and native). Most species have been tested in at least two separate experiments using different accessions of each plant species, with C. bonariensis plants used as positive controls in all experiments.
Our results thus far showed that P. cnici-oleracei (ex. Conyza) is highly host specific to C. bonariensis. The fungus successfully developed and produced telia only on the nine Australian accessions of C. bonariensis tested. While Conyza sumatrensis and Bidens pilosa developed necrotic flecks and in some instances a few large necrotic blotches, the fungus never produced any telia on these species. Chlorotic flecks developed on one accession of Calendula officinalis, and a few, rare pin-sized telia were observed on one replicate of Eschenbachia leucantha. Inoculation of C. bonariensis using these pin-sized telia did not result in any infection. All other non-target plant species tested did not develop any visible symptoms and were rated as either immune or highly resistant based on microscopic examinations of the development of the fungus on these species.
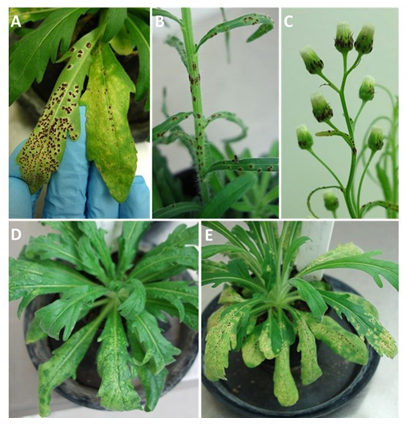
Conyza bonariensis infected by Puccinia cnici-oleracei. Telia on (A) abaxial and adaxial surfaces of leaves, (B) stems and (C) flower heads at four weeks after inoculation. Symptoms on whole plants at two (D) and four (E) weeks after inoculation.
Insects
Field observations of host-specificity have been made on insects recorded to date. As part of these field surveys, assessments were made on co‐occurring Asteraceae species (including species in the genera Eupatorium, Chromolaena, Ageratrum, Senecio, Bidens and Baccharis) to see if the insects being recorded on Conyza species are also found on these species. Trupanea bonariensis that forms stem galls, an unindentified fly that forms leaf-blister galls, a stem- and root-feeder weevil Lixus sp. and two species of moths (both unidentified, both leaf rollers) show promise for biocontrol. They cause significant damage and are seldom seen on co-occurring species in the Asteraceae in the field. Colonies of these species have been established in Brazil to elucidate their biology prior to importation into a quarantine facility in Australia to undergo detailed host-specificity testing (as part of a new Rural R&D for Profit project (Agrifutures Australia Project number: PRJ-12377; 2019-2022)).
Pending risks to non-target plants are acceptable, submitted application to the Commonwealth regulators seeking approval to release at least one potential agent. Upon receiving approval, released biocontrol agent(s)
Host-specificity testing with the rust fungus P. cnici-oleracei (ex. Conyza) was completed at the end of April 2020. A draft of the release application was prepared and submitted (Morin et al. 2020) to DAWE.
Explored options for integration of biocontrol with other management techniques
Conyza spp. (incl. all three exotic Conyza spp.) and S. oleraceus are similar in terms of their impacts in grain production systems. So, the options for integration of biocontrol with other management techniques are somewhat analogous.
Both weeds are principally managed through chemical and cultural control techniques in agricultural/cropping systems during the growing season (Wu 2007; Widderick 2014; Widderick & van der Meulen 2016; Widderick et al. 2004). This involves a combination of chemical control using postemergence herbicides of two herbicides (double-knock; either as a mix or applied sequentially) for in-crop control, and the use of a residual herbicide in fallows. The use of this single tactic has resulted in herbicide resistance in both weeds. Cultural tactics include decreased row spacing for in-crop weed management (Wu & Walker 2004), and the strategic use of tillage for burial of weed seed to below 2 cm depth in fallows (Werth & Walker 2007); the latter disrupts the benefits of minimum tillage farming.
Chemical approaches can manage both weeds effectively at a cost, but there is an opportunity for integration with biocontrol to possibly reduce costs and to preserve the utility of the effective herbicides by delaying resistance. Biocontrol agents (e.g. like Puccinia cnici-oleracei (ex. Conyza) being developed as part of this project) could serve as chronic stressors to the weed in fallows and outside cropped areas and limit reproductive output of the weed, thereby reducing the risk of seedbank build-up in fallows and also the risk of spreading into fields from surrounded non-cropped areas. The utility of biocontrol suppressing weed performance in unmanaged contexts (i.e. beyond crop fields and fallows) could further limit the rate of in-crop incursion of weed seeds within the growing season.
Integration of biocontrol with chemical and cultural control tactics will require coordination among land managers and consultants recommending/deploying management tactics for Conyza spp. and S. oleraceus.
Achievements of Rural R&D for Profit round 4 project: Underpinning agricultural productivity and biosecurity by weed biological control (2019-2023)
Pre-release monitoring of vegetation at selected future release sites of biocontrol agent(s)
Optimising sampling methods for flaxleaf fleabane infestations
During March-April 2020, we developed a sampling method to allow rapid assessment of fleabane population conditions at the long-term monitoring sites. This involved harvesting 126 reproductively mature fleabane plants from a variety of microhabitats throughout the ACT, including roadsides, drainage ditches, native grassy woodlands, stream embankments, urban footpaths and other urban green spaces. Statistical analyses revealed that a combination of (i) plant canopy volume (calculated from height, canopy diameter and foliage cover), (ii) number of inflorescences, which can be rapidly and non-destructively measured in the field and are strong, robust and positive predictors of plant biomass (a measure of plant productivity) and (iii) number of capitulae (a measure of plant reproductive effort) was the most effective assessment method.
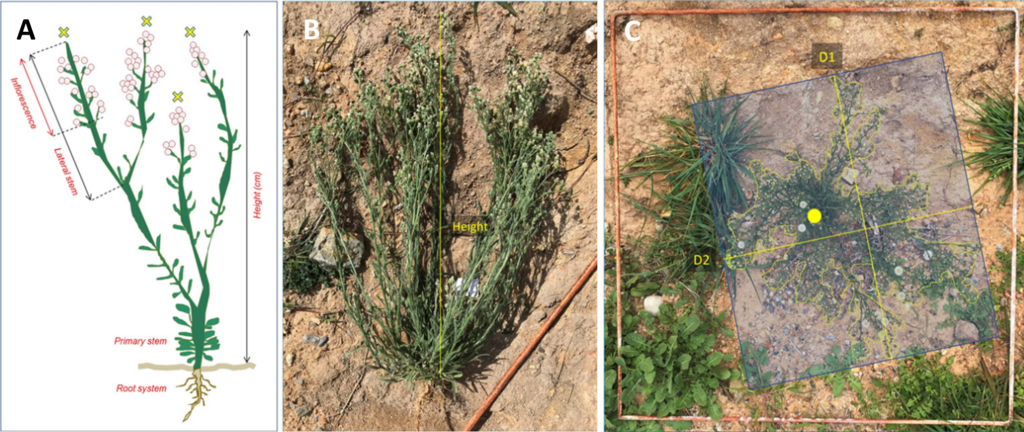
Schematic diagram showing (A) the typical habit of a flaxleaf fleabane (Conyza bonariensis) plant, with a shallow root system with a short, stocky tap root; and a short primary stem that branches into multiple lateral stems that bear one or more inflorescences (denoted by yellow crosses); small grey circles clustered on each inflorescence represent capitulae (i.e., flower heads bearing multiple florets). For each focal plant positioned at the centre of each 1 m2 plot (denoted by the yellow circle in image C), we measured (B) height (cm) from the soil surface to the tip of the tallest stem and (C) maximum canopy diameters (cm) in two directions (D1 and D2), parallel to the soil surface. Percentage planar foliage cover of the target plant (yellow dotted outline in image C) was visually estimated from the two-dimensional space bound by D1 and D2 (the blue square in image C).
Pre-release monitoring of flaxleaf fleabane populations
In November 2020, we identified six mixed-cropping sites suitable for the establishment of fleabane population monitoring and future releases of candidate biocontrol agent(s), located across the Riverina Irrigation Region of NSW. The aim of this field trip was to identify the different microhabitat characteristics of irrigated mixed cropping systems in which crop weeds, especially fleabane, can persist during fallow periods and pose significant challenges to effective weed control across land tenures. The identified microhabitats that will be targeted during future monitoring include roadsides, fence lines, field margins, draining ditches and irrigation embankments.
Flaxleaf fleabane population sampling
Between February and May 2021, we established 45 transects (20 m length) at 9 sites located throughout the Murrumbidgee Irrigation Area, between the townships of Griffith and Leeton, NSW. These transects were established in each of four different habitats comprising the highest densities of fleabane infestations: along roadsides, drainage ditches, field margins and irrigation embankments.
We randomly sampled 10 fleabane plants along each transect, thus resulting in data being collected for a total of 450 plants. For each plant we measured height, foliage cover and number of inflorescences, which were previously shown to be robust surrogates for productivity (i.e., biomass) and reproductive output. A 1 m × 1 m quadrat was also placed around each focal plant, in which we counted the number of fleabane plants (as a measure of population density) and visually estimated overall fleabane % foliage cover. We also estimated the % cover of several abiotic (e.g., bare soil cover) and biotic (e.g., herb and grass cover) variables.
We found that fleabane plants were significantly larger along drainage ditches and irrigation embankments compared with roadsides and field margins. Reproductive output per plant was more than two-times higher along drainage ditches and field margins than either roadsides or irrigation embankments, and declined with increasing cover of other plant species, especially grasses. Population density (i.e., number of individual plants per 1m2) tended to be higher along roadsides and irrigation embankments than either drainage ditches or field margins. The overall % cover of fleabane populations did not vary amongst the four habitat categories but was positively associated with bare soil cover and negatively associated with the cover of herbs and wireweed. This suggests that fleabane cover may be suppressed under high competition with other herbaceous species, or that fleabane could benefit from disturbances (e.g., roadside slashing or spraying) that create bare soil patches not suitable for the establishment of other herbaceous species.
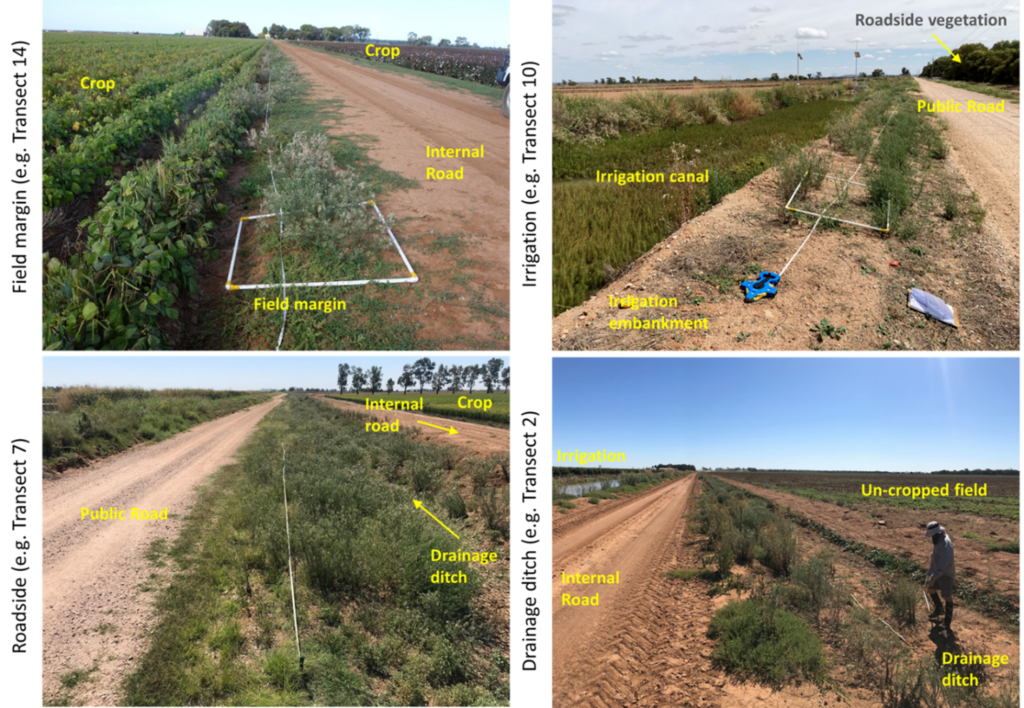
Photographic examples of each of the four main habitats in which flaxleaf fleabane populations were sampled in 2021.
Approved release of the pathogen from biosecurity containment and establishment of a laboratory culture
Flaxleaf fleabane was endorsed as a candidate for biological control in Australia in November 2017 by the Invasive Plants and Animals Committee (now the Environment and Invasives Committee). Comprehensive host-specificity testing for the rust fungus, Puccinia cnici-oleracei (ex. Conyza) obtained from Colombia, South America, began in quarantine in February 2019, during the previous RRnDFP2 project, although testing remained incomplete and was finalised during this current RRnDFP4 project. The application to release the fleabane rust fungus was submitted to the federal regulators on 30th June 2020.
The Department of Agriculture, Water and the Environment (DAWE) approved the release of the rust fungus Puccinia cnici-oleracei (ex. Conyza) for the biological control of flaxleaf fleabane on 17 June 2021. Leaves infected with the fungus were removed from the quarantine facility on 21 June, in the presence of DAWE officers. Since then, a culture on live plants has been established in a non-quarantine controlled-environment room at the CSIRO Black Mountain site in Canberra.
A viable, healthy culture of the rust fungus P. cnici-oleracei (ex. Conyza) was maintained on Conyza bonariensis (flaxleaf fleabane) seedlings within controlled temperature (CT) rooms at CSIRO’s Black Mountain Laboratories in Canberra (culture maintenance ongoing). Infected leaves with mature pustules were then removed from the recipient plants, dried flat to preserve the spores, and stored in the dark within airtight plastic containers with silica desiccant at 4 ˚C. In this way, we accumulated a stockpile of over 1,000 severely infected fleabane leaves for provision to registered community participants in the pilot mass-release program which ran from September 2022 to March 2023.
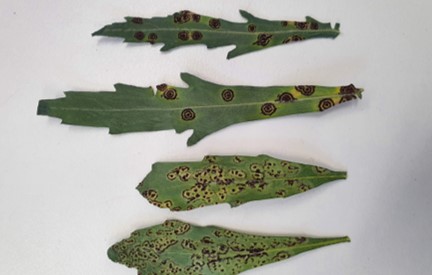
Examples of dried flaxleaf fleabane leaves infected with the biocontrol agent.
Experimental optimisation of pathogen release methods in the field
Several experiments were undertaken with the aim of optimising release methods for the rust fungus in a field context and initiating infection by the fungus on naturalised flaxleaf fleabane populations under prevailing climate conditions in the Australian environment. It had been determined that the best way to inoculate plants was to attach a dried infected leaf to moistened paper towel, which was then suspended above the target plants and covered with a plastic bag.
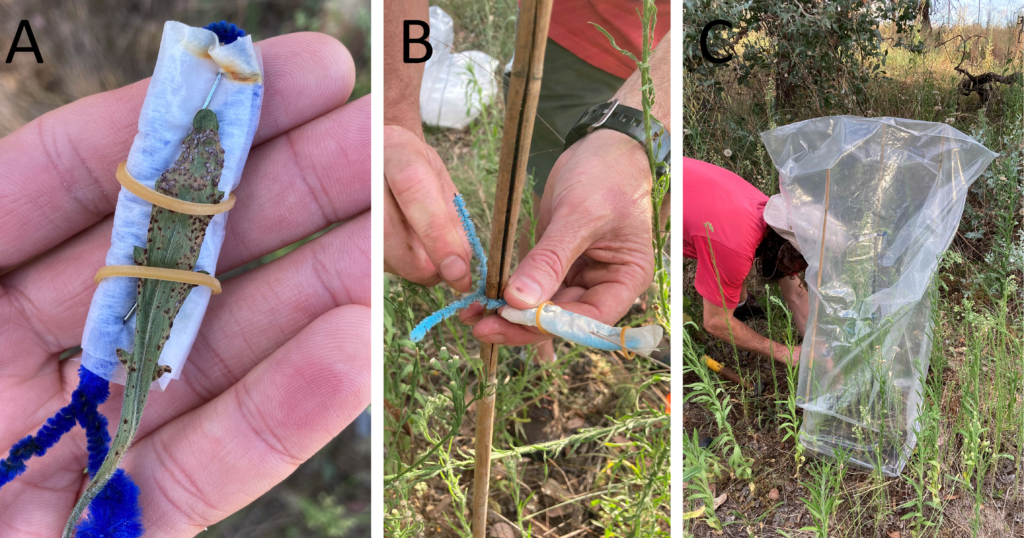
Release of the rust fungus at the Ginninderra Lake site in the ACT.
In December 2021, the fungus was experimentally released on potted flaxleaf fleabane seedlings and maintained for several months under field conditions to monitor for patterns of disease incidence and severity, and effects of fungal infection on plant performance. After a month in January 2022, infection monitored across the treatments provided evidence that maintaining a still, humid microclimate at the time of inoculation is critical for successful infection by the fungus from the lab-reared inoculum to seedlings.
Multiple infection events occurred between January and May 2022, whereby the first set of lesions that developed in January produced spores that spread to nearby healthy leaves that subsequently became infected, and so on. Infection progressed over the entire body of each plant, with lesions eventually being detected on stems and flower heads. These observations confirmed that the fungus was able to readily infect fleabane plants growing outdoors over multiple months, under variable climate conditions.
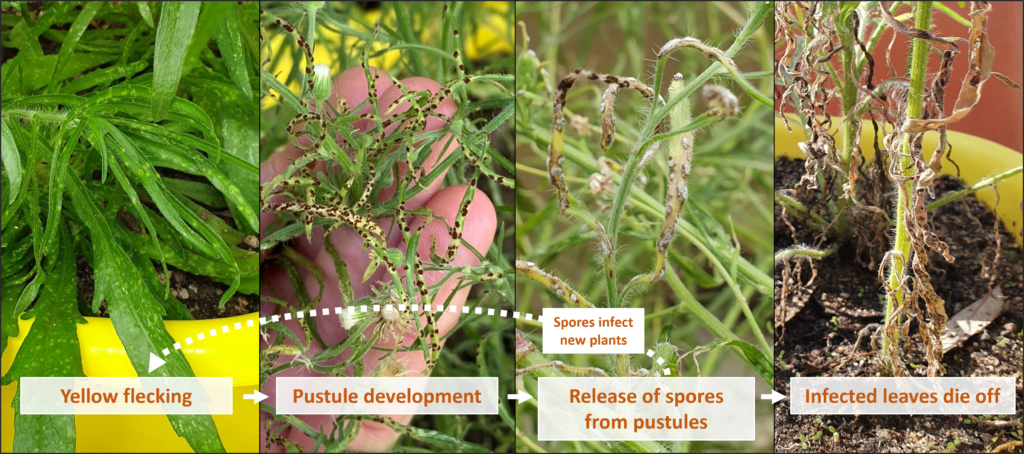
Depiction of disease progression for the fungus on Conyza bonariensis
At the conclusion of the experiment in May 2022, seedlings were harvested, dried, and measured. The reproductive output (measured as the number of flower heads per inflorescence) declined significantly with increasing percentage of leaves on each inflorescence infected by the fungus. On average, severely infected inflorescences produced 50-60% fewer flower heads than non-infected inflorescences. These results indicate that, under high infection severity of developing inflorescences, the fungus can reduce the overall reproductive output of host flaxleaf fleabane plants.
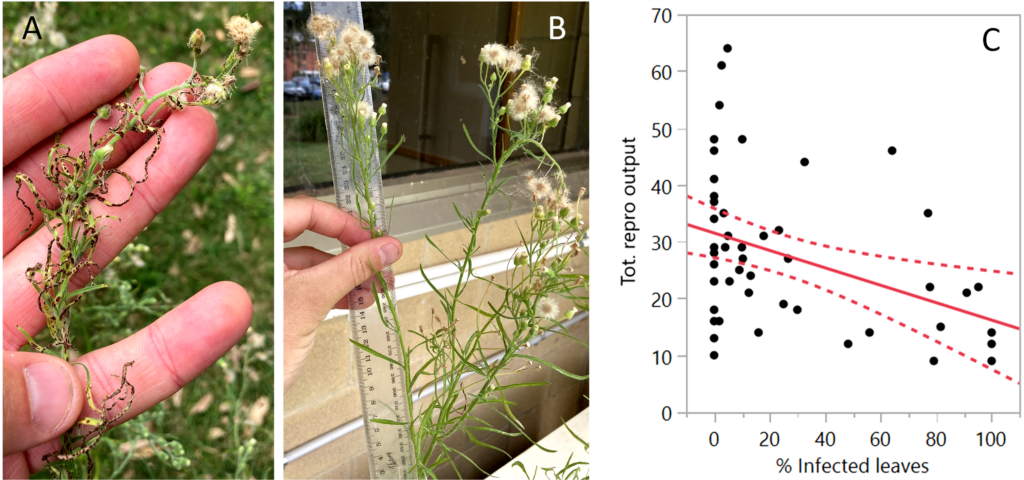
Contrast of the inflorescences where (A) has severe infection by the fungus and stunted flower heads while (B) no infection by the fungus. The graph depicts the relationship between number of flower heads (capitulae) (y axis) and % leaves per inflorescence infected with the fungus (x axis).
In February 2022 and again in November 2022, a total of 24 release plots were set up in the Irrigation Research and Extension Committee (IREC) research station, near Whitton, to test release methods further across different habitat conditions. When returning to the sites to monitor the release plots, infection by the rust fungus was not detected on any focal plant within each of the release plots. Often by the time monitoring occurred, most plants had begun to senesce, with a high cover of dead leaves, making it difficult to determine if any prior infection had occurred between inoculation date and monitoring date.
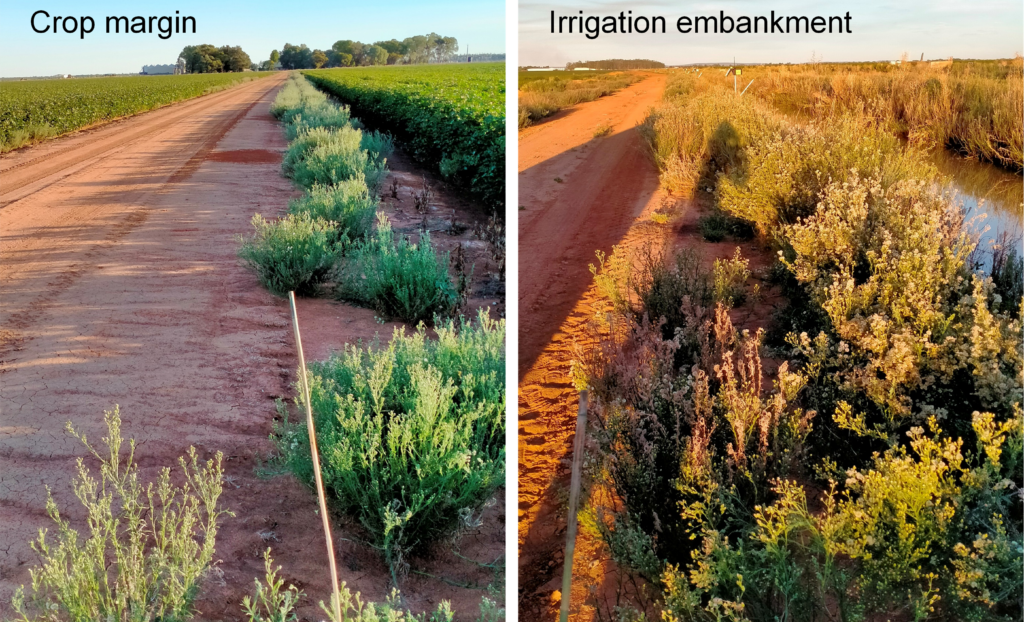
Example of two transects in which the rust was released on flaxleaf fleabane plants in February 2022.
Further experimental releases of the fungus commenced in December 2022 on a naturalised flaxleaf fleabane population located at the CSIRO Black Mountain site in the ACT. Two release methods were trialled; for the first method, flaxleaf fleabane plants were inoculated by suspending an infected leaf above each of the target plants and covered with a large, clear plastic bag or opaque plastic box. For the second method, 12 severely infected flaxleaf fleabane plants growing in 15 cm pots were installed at 12 plots with clusters of field grown plants. Neither bags nor moisture were applied to these plants, as the aim was to allow gradual spore release over a longer period under field conditions.
At one month post inoculation, all plants were inspected for signs of fungal infection. For the first method, 100 % of plants (presence/absence) displayed at least some level of infection. The severity of infection was generally low, ranging from 1-10 % of leaves infected per plant. For the second method, ~58 % of plots showed low levels of infection on resident plants.
By March 2023, approximately 3 months post release, many of the infected leaves that were detected in January-February had died off. We did, however, detect infection on other parts of the plants – namely stem lesions – including of nearby plants, which indicated some spread of the fungus throughout the local population.
The results from these experiments have provided insights into optimal methods for releasing the rust fungus on flaxleaf fleabane:
- Infection by the rust fungus cannot readily occur without creating a humid microclimate around a recipient plant during sporulation, using a plastic bag or other enclosure for at least 12 hours.
- It is likely that younger seedlings are more susceptible to infection by the rust fungus than mature plants, which are likely to be relatively more resistant to infection.
- There is a need to monitor infection incidence and severity at high frequency over short time periods within the first three to four weeks following release of the fungus. Where infection does occur, telia may only be visible for a few days, after which they release spores and cause the host leaf to die off and shed from the infected plant.
Community engagement a in pilot mass-release program of the pathogen across Australia
From September 2022 until February 2023, CSIRO researchers launched a pilot biocontrol agent release program in partnership with landholders/growers and other weed management stakeholders from the grains sector, with the aim of trialling the optimised release protocols for the rust fungus in a variety of landscape and climate contexts. Interest from potential participants in the program was elicited through a joint GRDC-AgriFutures-CSIRO media campaign and face to face workshops.
- https://grdc.com.au/news-and-media/audio/podcast/flaxleaf-fleabane-rust-fungus
- https://groundcover.grdc.com.au/innovation/plant-breeding/new-fungus-to-help-landholders-fight-fast-spreading-weed
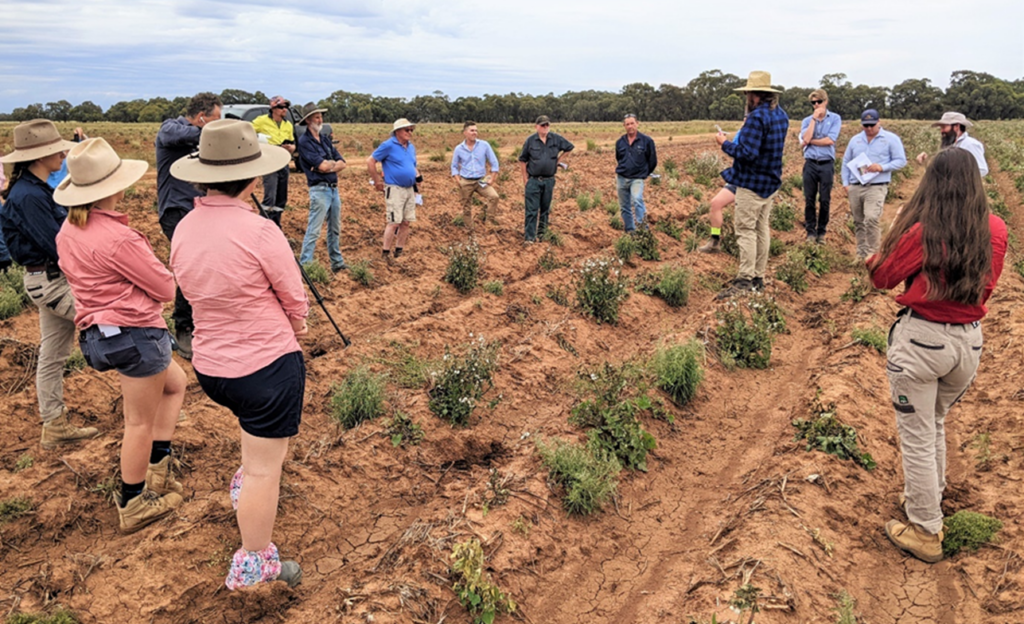
Ben Gooden running a face-to-face workshop on the flaxleaf fleabane biocontrol project for IREC, Murrumbidgee Irrigation and local growers in the Riverina, 10th November 2022.
Fifty-five stakeholders were selected for participation in the program, comprising of private landholders/growers, professional agronomists, research institutes, local councils, and Landcare networks. Participants were sent their own biocontrol agent release kits which included a dried flaxleaf fleabane leaf (or multiple leaves) that were infected with the rust fungus, other tools to prepare and apply the agent, and release and monitoring instructions. Altogether, 366 biocontrol agent release kits were distributed nationwide, focussing on the south-eastern parts of Australia where flaxleaf fleabane infestations cause the greatest impacts on crop yield. From participants who returned to their sites to monitor for the fungus, only ~10 % were able to observe infection.
Several challenges were identified with regards to eliciting verified infection presence/absence data from participating stakeholders. In at least 10 % of cases, inspection of photographs at the time of release indicated the participants had released the fungus on a different plant species, most often tall fleabane (Conyza sumatrensis), which is resistant to infection by the rust. In ~6 % of cases, the host flaxleaf fleabane plants had become senescent by the time the participants had returned to inspect the plants for signs of infection. During the CSIRO-run experiments, we observed that pustules can appear on the leaf surface after about 2-4 weeks, after which time the host leaves die off very quickly (within a couple of days) after the fungus has sporulated. As such, the incidence of infection was likely much higher at the community release sites, but the low frequency of return visits meant that many visible symptoms were likely missed by the participants.
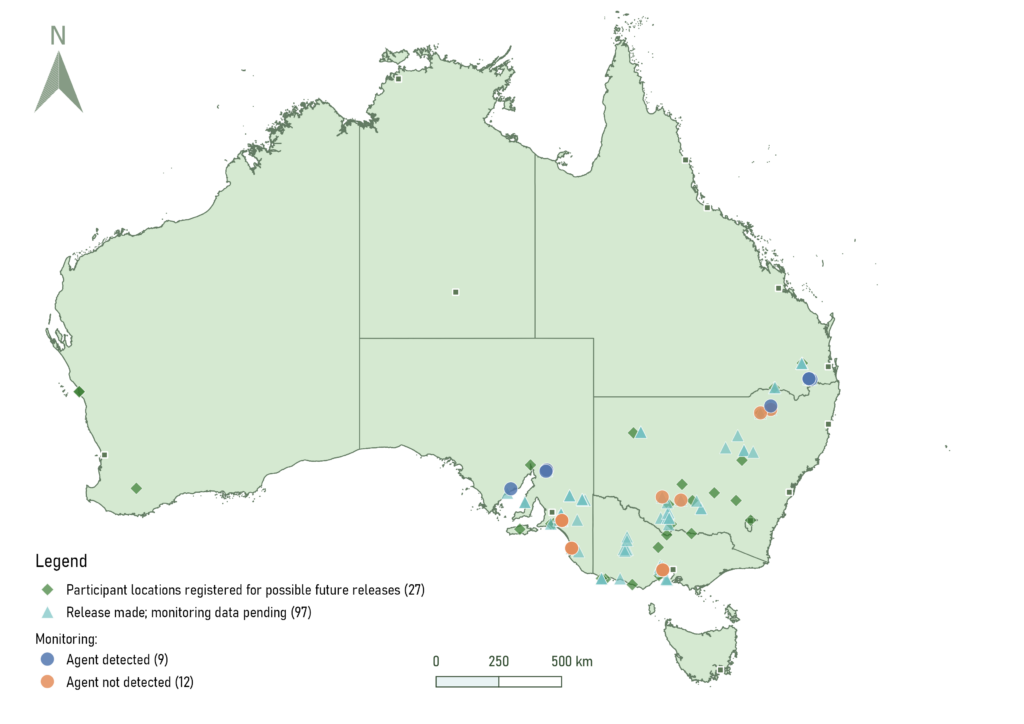
Map of release locations of the flaxleaf fleabane rust fungus in partnership with community stakeholders.
Future research aspirations for biocontrol of flaxleaf fleabane with the rust fungus
Based on the results obtained so far, it is predicted that, even where the rust fungus can establish successfully in the field, infection is unlikely to result in significant reductions in the population size of flaxleaf fleabane for several years. As such, biocontrol represents a longer term and self-sustaining means of gradually reducing weed invasion pressure across productive landscapes. Furthermore, the combination of several biocontrol agents may enable more robust control of focal weeds. In this way, further research into the biocontrol of flaxleaf fleabane with insects may provide enhanced biocontrol outcomes.
Initiate native range research to identify additional candidate agents for Fleabane
Native range surveys were conducted in Brazil (Santa Catarina; Parana; Sergipe; Alagoas; Pernambuco states), Colombia (Antioquia department) and USA. In addition to the insect species reported and prioritized (e.g., Trupanea bonariensis, Astteromyia modesta and Lixus caudiger) as part of the RRnD4P Round 2 project (2016-2020), the following insect species were recorded feeding on Conyza spp:
- Brazil: a new unidentified weevil species, a leaf miner, a stem feeding thrips, Clinodiplosis sp, (Cecidomyiidae) and a micro lepidoptera (Torticidae)
- Colombia: Eutreta rhinophora (Tephritidae), Caloreas cydrota (Choreutidae) (reported as Argyrotaenia in previous report), Lioptilodes sp. (Pterophoridae), Puto barberi (Putoidae) and Proba vitiscuttis (Miridae)
- USA: Neolasioptera erigerontis. Colonies of most of these species were maintained in the native ranges for various biology and host specificity testing (detailed below). Based on the details in the existing literature, barberi and P. vitiscuttis recorded in Colombia were considered generalists and thus considered to have no potential as biocontrol agent.
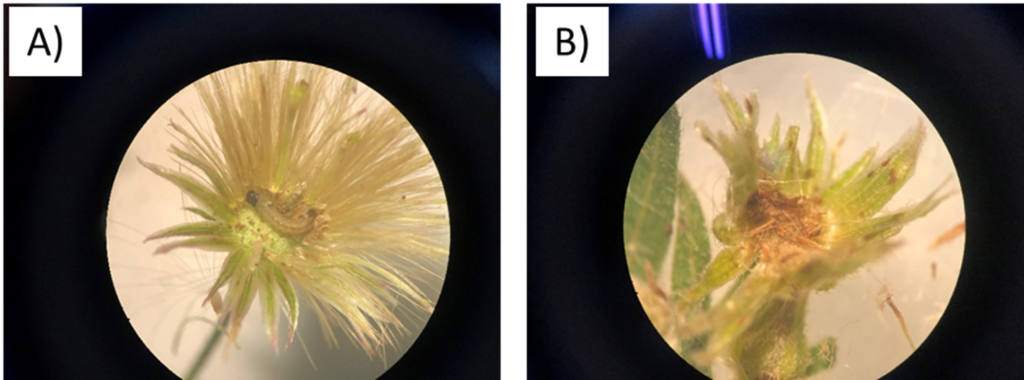
(A) larva of the micro lepidoptera recorded in Brazil, (B) Damage caused by the larval feeding – floral bud with no seeds
Undertake prelimary host-specificy testing to priortise candidates for importation
Stem-boring fly Trupanea bonariensis on flaxleaf fleabane
Host-specificity testing were carried out using the stem-galling fly (T. bonariensis) in Brazil by exposing representative plant species in the genera Conyza, Eupatorium, Porophyllum, Emilia, Symphyotrichum, Chromolaena, Bidens and Baccharis to T. bonariensis.
Only species in the genus Conyza developed galls induced by T. bonariensis. The species Conyza glandulitecta developed the highest number of galls, followed by C. sumatrensis var. sumatrensis, and with only one gall on the species C. bonariensis.
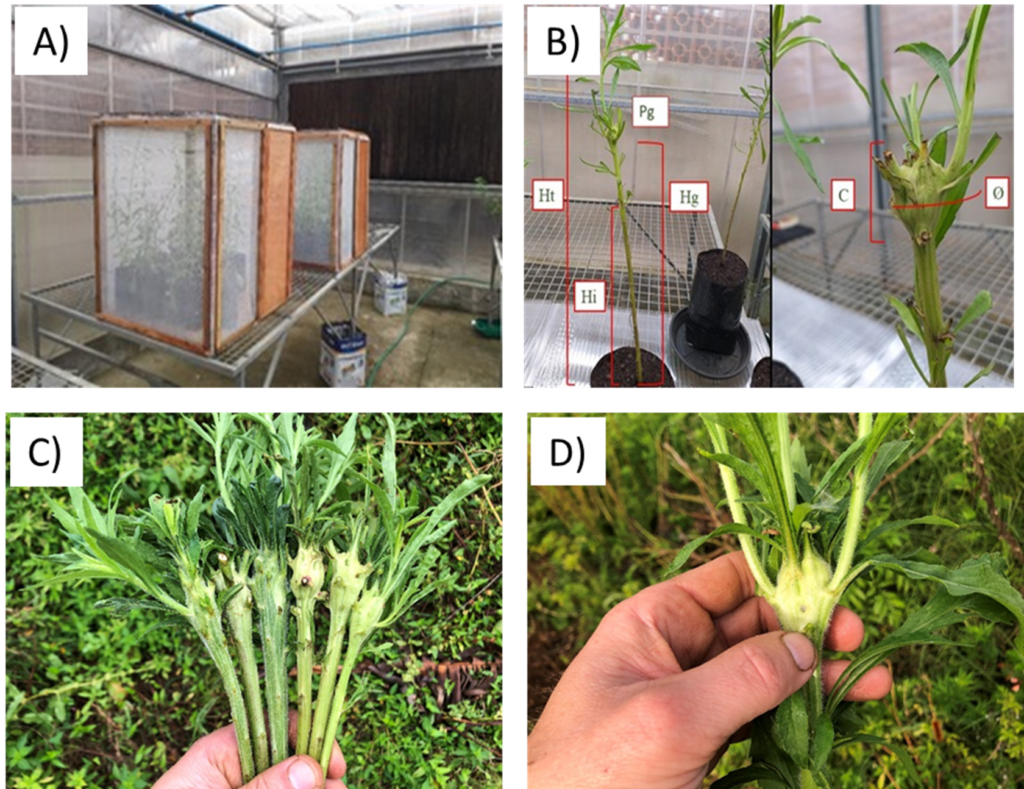
(A) Entomological cages containing Asteraceae species for no-choice tests, (B) making measurements of galls formed in Conyza glandulitecta, (C & D) galls formed by Trupanea bonariensis on Conyza species
Micro Lepidoptera (Torticidae) on flaxleaf fleabane
Host-specificity testing of the micro lepidopteran species (Torticidae) was conducted in Brazil using 43 Asteraceae species. This species was recorded in all field expeditions causing defoliating damage to the five species of Conyza, with a greater extent in C. bonariensis and C. sumatrensis var. sumatrensis. A test was carried out in the field, selecting and measuring the morphological characteristics of two adult Asteraceae plants Bidens pilosa and Praxelis clematidea. In addition, specificity tests were carried out using cut leaves. For studies with cut leaves, larvae were introduced into each petri dich containing leaves of test plants. The experiment was carried out in 43 species of Asteraceae.
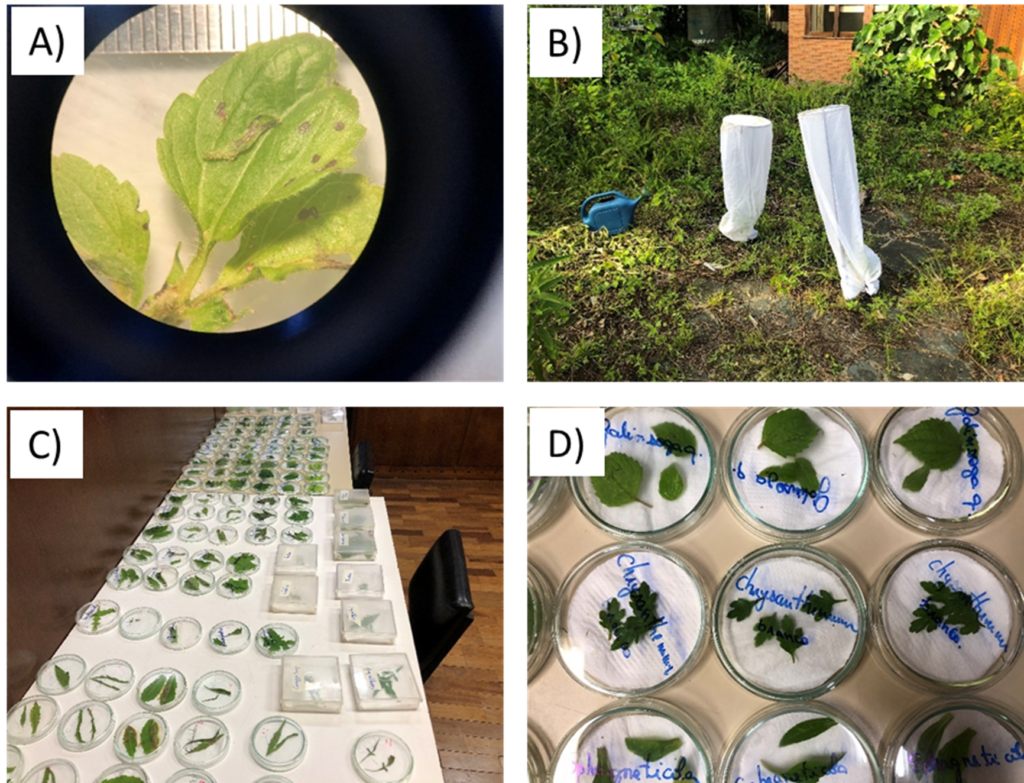
A) Defoliating damage caused by the Torticidae larvae on leaves of Praxelis clematidea, (B) cages setup with Tortricidae caterpillars, (C & D) Petri dish test with Tortricidae caterpillars on leaves of 43 species of Asteraceae
At the end of the trial, observations on the damage caused by the larvae and emergence of adults were made. Larvae were found to reach the adult stage from the following species: B. pilosa, E. fosbergii, Erechtites valerianifolius, Sonchus asper, Elephantopus mollis, Erechtites hieracifolius, C. bonariensis, C. canadensis, C. glandulitecta, C. sumatrensis var. sumatrensis, C. sumatrensis var. leiotheca and C. primulifolia. This candidate agent does not have a sufficiently narrow host range relative to other candidate agents (T. bonariensis, A. modesta and L. caudiger) thus it was not prioritized for importation into Australia.
Leaf-galling fly Asteromyia modesta on flaxleaf fleabane
No-choice specificity tests were carried out in Brazil. The specificity tests were conducted in 73 plant species from 66 genera spanning 20 families. Conyza sumatrensis var. sumatrensis was considered as a control in these trials because of lower number of galls induced by A. modesta on C. bonariensis. All experiments were carried out in “pop up” entomological cages, and at the end of the trial, observations on total number of leaves per plant, number of leaves with galls and total number of galls per plant were recorded.
None of the 73 plant species tested developed galls except C. sumatrensis var. sumatrensis.
In addition to no-choice tests, choice tests were performed to determine host preference of A. modesta for the species within the genus Conyza. After 20 days exposure to the insect, the total number of galls and number of leaves with galls were greater on Conyza sumatrensis var sumatrensis than on the other species (C. bonariensis, C. canadensis, C. glandulitecta, C. primulifolia, C. sumatrensis var. leiotheca).
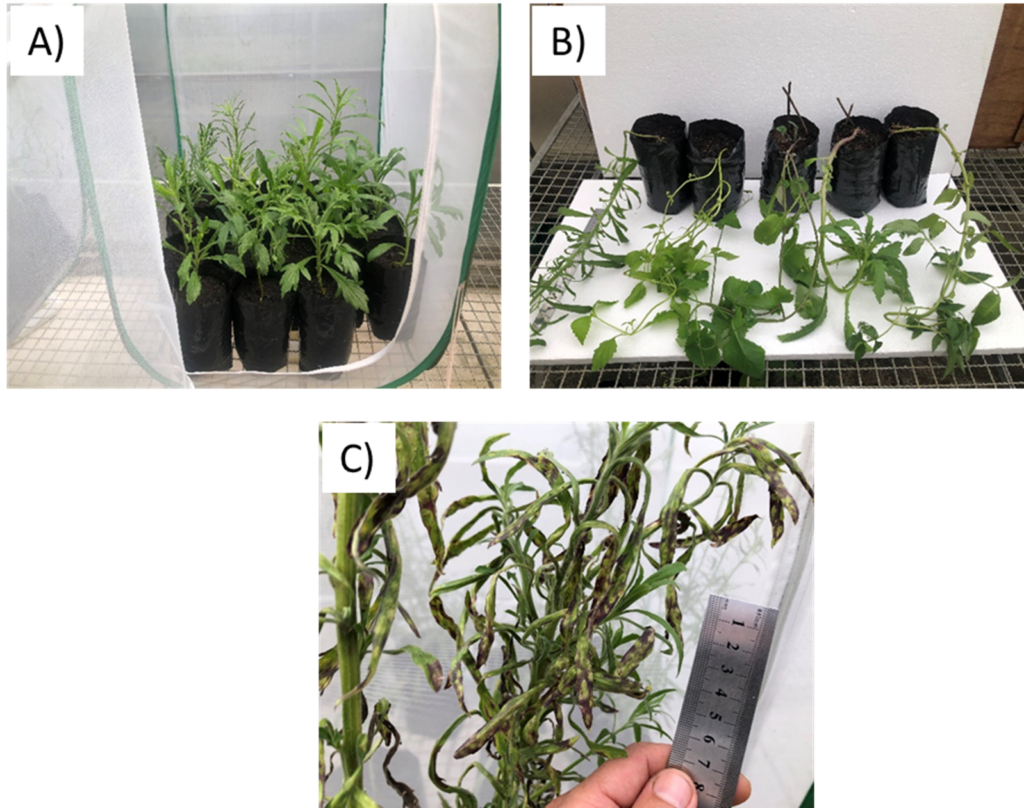
Seedlings of Conyza spp. caged for choice tests, (B) observations made after 20 days of exposure to Asteromyia modesta and (C) dgalls formed by Asteromyia modesta on Conyza sumatrensis var. sumatrensis
Establish and maintain colonies of candidate agents in the native range colony
Colonies of five insect species, Lixus caudiger, an unidentified new weevil (Curculionidae), Asteromyia modesta, Clinodiplosis sp. and Trupanea bonariensis were maintained during the project period (2019-2023). Insects from these colonies were used for various host specificity and biology studies. These colonies were used to ship agents to France (L. caudiger and A. modesta) and Australia (T. bonariensis and A. modesta). Colonies of these five species are still being maintained to import to Australia for host testing should the resources become available for ongoing research on these prioritized insect agents.
Importation of new candidate agent(s) into quarantine to and commence testing
Trupanea bonariensis:
A total of three shipments were made during November 2019, November 2020 and January 2021 to establish a colony under Australian quarantine condition. Hand carrying of this agent was not possible due to COVID-19 impact on travel. Adults emerging from these shipments were introduced into cages containing 3 or 4 potted plants provided as either C. bonariensis only, C. sumatrensis only or a combination of both plant species for oviposition. Number of cages set up were 9, 7 and 13, respectively during the first, second and third shipment.
Plants were exposed to adult T. bonariensis and the adults were collected and reintroduced into another cage after the exposure period of 3 to 15 days. This process of reintroducing adults to fresh plants continued until all imported adults were dead. A total of nine and seven cages of plants exposed to T. borarienesis were maintained during the first and second shipment. These cages with exposed plants were maintained and observations on possible gall formation were made.
No galls were noticed on plants post-exposure and dissections of plants failed to reveal any evidence of oviposition or larval development. It was unclear why the adults did not oviposit on Conyza plants exposed to them. This could be because pupae perhaps have been damaged during the transit or bulk processing of the packages with X-ray screening might have induced sterility.
Trupanea bonarensis is a promising biocontrol agent for C. bonariensis because of its high specificity and the damage it causes to plants. The difficulty to establish a colony is because of the inability to hand carry insects to Australian quarantine and the project team was unable to travel to Brazil to learn key techniques and knowledge about the agent to rear them under quarantine conditions.
Asteromyia modesta:
Asteromyia modesta midge was sourced from a colony established at the FURB laboratories (Blumenau, Brazil) and shipped to Australia and France. These colonies were established using field collected insects from C. sumatrensis. Because hand carrying of agents was impossible because of COVID-19 restrictions on international larval, shipments through international courier were attempted. Larvae/pupae derived from the clean culture, as leaf galls on clean C. sumatrensis leaf material, were shipped to Australia during May and October 2021, and to France during August 2021, September 2021 and February 2022.
A colony of A. modesta could not be established either in Australia or France. The shipments were considerably delayed during transit and the insect did not survive on arrival in Australia during the first shipment. During the second shipment, a total of 160 adults emerged from the imported materials, and these adults were setup in 10 cages each with 12 to 20 adults. However, there was no evidence of oviposition or other development across all the cages and a colony could not be established. It was presumed that possible exposure of the package containing insects to X-ray screening during the transit could have induced sterility in emerged adults. The shipments sent to France were also delayed and all larvae were dead on arrival.
Lixus caudiger:
French import permits for the stem-boring weevil Lixus caudiger were obtained and the agent was sent via courier by collaborators in Brazil in mid-May 2021. A colony of the stem-boring weevil were initiated using both C. bonariensis and C. sumatrensis plants. The adults L. caudiger fed on the leaves of both Conyza species from the margins to the leaf axis, and oviposition marks were observed two weeks after the arrival of the weevils. The eggs are yellowish, oval, about 0.8 x 1.6 mm. Newly hatched larvae seemed to bore directly into the stem and feed on pith and cambium tissue. Using these weevils, a colony of L. caudiger was successfully established in the laboratory, and the colony produced more than 300 individuals and these adults were used in the host specificity testing.
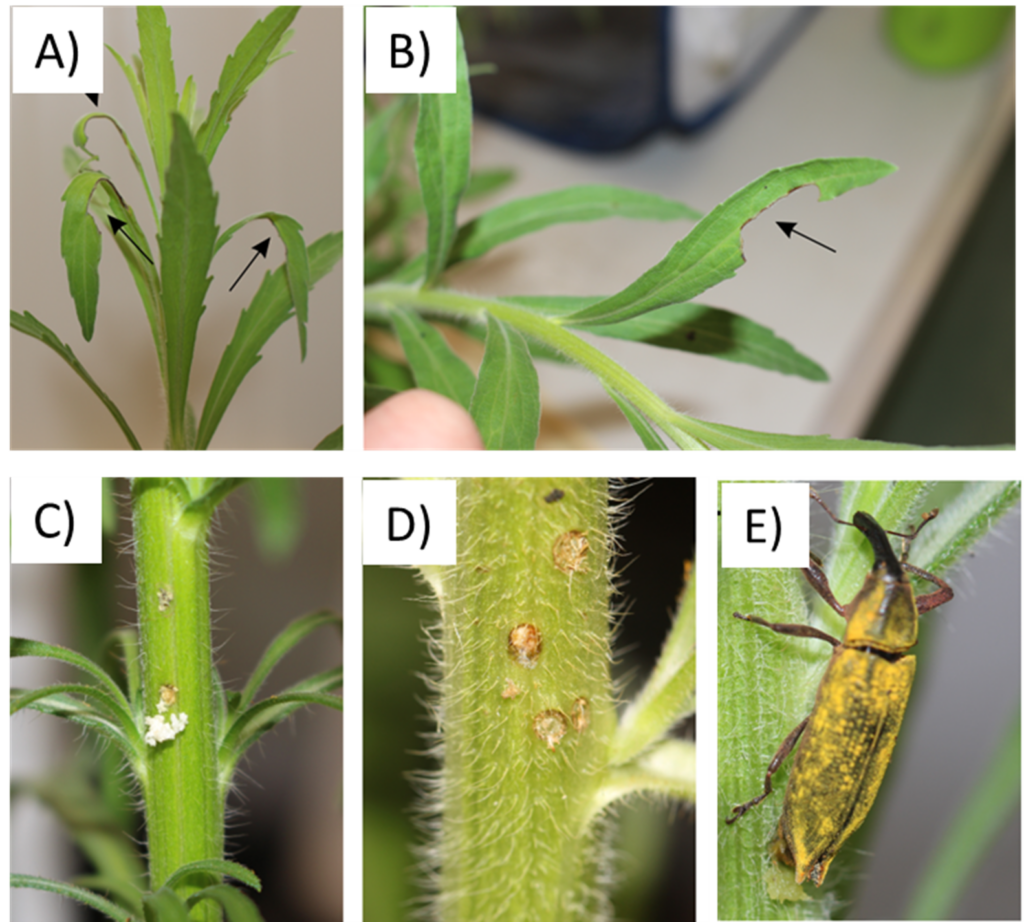
Observations performed on Conyza bonariensis (A&B) Adult feeding damage, (C&D) oviposition marks in the stem, and (E) female ovipositing in a stem.
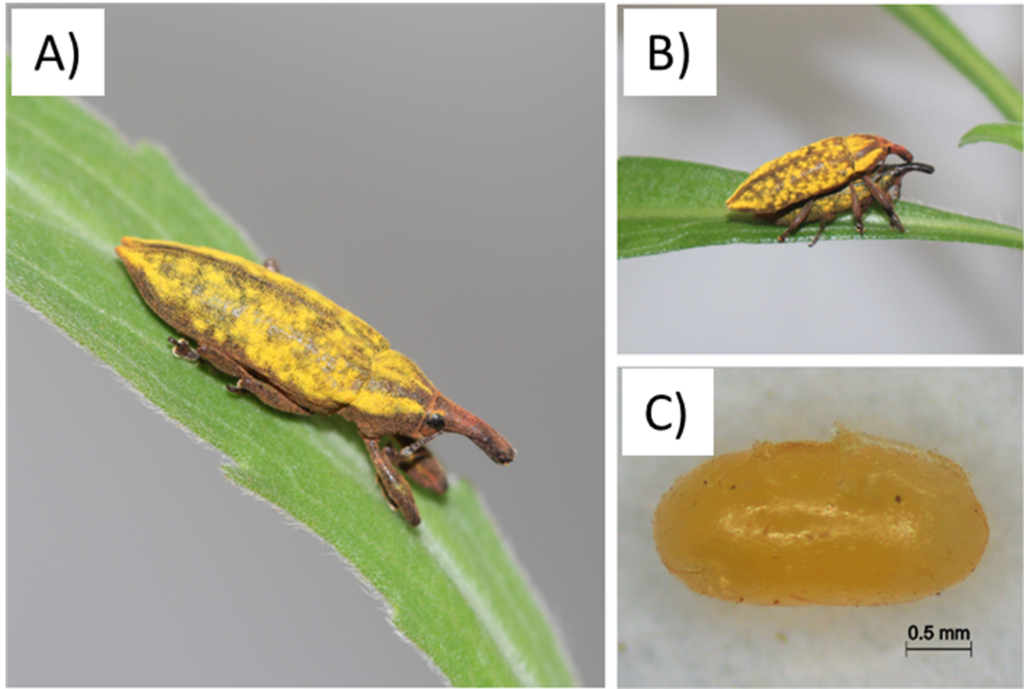
The stem-weevil, Lixus caudiger (A) Adult resting on a Conyza leaf; (B) Pairs in copulation, and (C) Egg laid by L. caudiger.
No-choice tests: 14 species in the subfamily Asteroideae belonging to four different tribes and ten subtribes were tested in adult no-choice trials. Pairs of sexually mature adults were placed on a plant of each species and placed in screened cages. Survival of adults, herbivory and the presence and number of oviposition marks were recorded.
Adult feeding was observed on several but not all tested species. The feeding intensity was far smaller compared to C. bonariensis and other Conyza species. For instance, our results on crops and native Australian species showed no feeding or less feeding relative to the focal weed. Likewise, mortality of adults on those species was also always higher than on Conyza species. While herbivory was quite high on Erigeron karvinskianus, a closely related species to Conyza that is used as ornamental, no oviposition mark was observed; this was confirmed by dissecting the stems. It is unlikely that this species supports the L. caudiger larval development.
Egg laying was observed only on Conyza species, with no differences between the three different Conyza species (C. bonariensis, C. sumatrensis and C. canadensis) in terms of L. caudiger mortality, number of oviposition marks, and herbivory. These species seem to be equivalent hosts for L. caudiger in a no-choice setting. The high mortality observed at the end of the test on most species except Conyza and Erigeron, combined with low herbivory suggest that non-target effects should be minimal on other tested species.
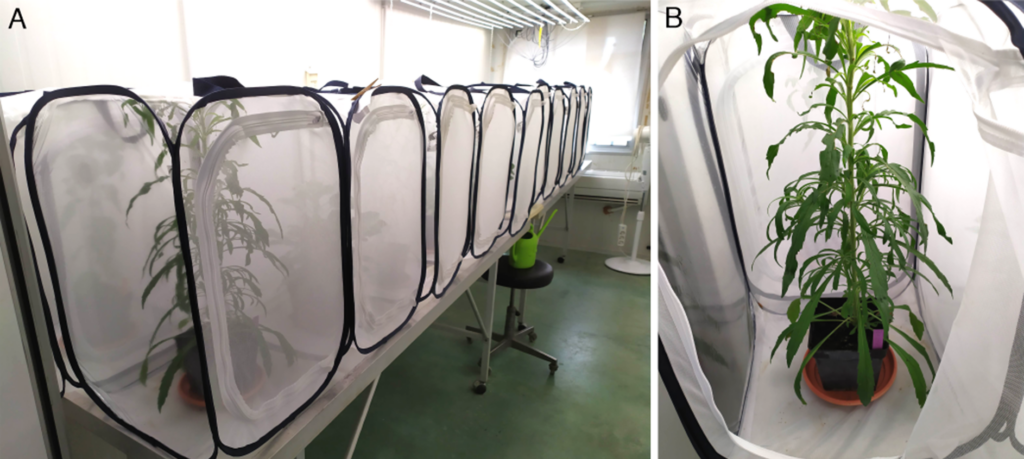
No-choice test set-up for Lixus caudiger. (A) Series of test performed in CT room; (B) Example of size of the plant used in host testing
Choice tests: Choice trails were conducted to investigate if L. caudiger has any preference for E. karvinskianus and C. bonariensis. Experimental setup used were similar to no-choice tests, except that both plant species were maintained in a same cage.
Adult feeding was observed on both plant species; however, the feeding intensity was far greater on C. bonariensis than on E. karvinskianus. Moreover, the different plant parameters measured at the end of the test showed that the feeding of the weevil did not impact E. karvinskianus growth, and there was no difference in growth of this species between the plants that were damaged by adult feeding and control plants not damaged by adult feeding. In contrast, adult feeding and/or larval development seemed to impact the development of C. bonariensis. The number of flower heads per plant was significantly lower (by ~30%) in plants damaged by L. caudiger compared to the control plant.
The results from the choice and no-choice host-specificity testing are very promising. The host range of L. caudiger appears to be restricted to Conyza. All Conyza species included in the tests are introduced weeds in Australia. Further host specificity testing under quarantine conditions in Australia is required to screen other non-target plants in the host test list.
References
Farr DF, Rossman AY (2020) Fungal databases. U.S. National Fungus Collections, ARS, USDA, Available at: https://nt.ars-grin.gov/fungaldatabases/ Accessed 15 March 2020
GBIF.org (24th July 2018) GBIF Occurrence Download https://doi.org/10.15468/dl.pbd67p.
*Hunter GC, Rafter MA, Raghu S and Morin L (2018) Proposed plant host test list for assessing risk of candidate biological control agents for Conyza bonariensis. Prepared by CSIRO.
Llewellyn R, Ronning D, Clarke M, Mayfield A, Walker S, Ouzman J (2016) Impact of weeds on Australian grain production – The cost of weeds to Australian grain is in the adoption of weed management and tillage practices. CSIRO, Australia.
Mangolin CA, Silvério de Oliveira Junior R, de Fátima P.S. Machado M (2012) Genetic Diversity in Weeds. In: Alvarez-Fernandez R (ed) Herbicides – Environmental Impact Studies and Management Approaches. IntechOpen, Available from: https://www.intechopen.com/books/herbicides-environmental-impact-studies-and-management-approaches/genetic-diversity-and-structure-of-weed-plant-populations. doi:DOI: 10.5772/32733.
McClintock D, Marshall JB (1988) On Conyza sumatrensis (Retz) E. Walker and certain hybrids in the genus. Watsonia 17: 172–173.
Michael PW (1977) Some weedy species of Amaranthus (amaranths) and Conyza/Erigeron (fleabanes) naturalized in the Asian-Pacific region Proceedings of the 6th Asian-Pacific Weed Science Society Conference, Indonesia, 1977:87–95
PlantNET 2020, NSW Flora Online (http://plantnet.rbgsyd.nsw.gov.au/floraonline.htm)
*Rafter M, Morin L (2017) Nomination of a target weed for biological control: Conyza bonariensis L. (Asteraceae). Prepared by CSIRO.
Scott JK, Yeoh PB, Michael PJ (2016) Methods to select areas to survey for biological control agents: An example based on growth in relation to temperature and distribution of the weed Conyza bonariensis Biological Control 97: 21–30 doi:10.1016/j.biocontrol.2016.02.014
Thébaud C, Abbott RJ (1995) Characterization of invasive Conyza species (Asteraceae)in Europe: Quantitative trait and isozyme analysis. American Journal of Botany 82: 360–368.
Thébaud C, Finzi AC, Affre L, Debussche M, Escarre J (1996) Assessing why two introduced Conyza differ in their ability to invade Mediterranean old fields. Ecology 77: 791–804.
Werth J, Walker S (2007) Tillage effects on fleabane emergence Proceedings of a fleabane workshop held at Queensland Department of Primary Industries and Fisheries in Toowoomba on 7th February 2007, pp. 22–23.
Widderick M and van der Meulen A. (2016) Ecology and management of common sowthistle. Available at: https://www.youtube.com/watch?v=I_5gm-ZhGE0 Accessed 17 March 2017: Grains Research and Development Corporation.
Widderick M (2014) Weed 15: Common sowthistle (Sonchus oleraceus), in Storrie, A. M. (ed), Integrated weed management in Australian cropping systems. Grains Research and Development Corporation.
Widderick M, Walker S and Sindel B (2004) Better management of Sonchus oleraceus L. (common sowthistle) based on the weed’s ecology. In Proceedings of the 14th Australian Weeds Conference. 6–9 September 2004, Wagga Wagga, New South Wales, Australia. Sindel, B.M. and Johnson, S.B. (eds), pp. 535-537, Weed Society of New South Wales, Sydney, New South Wales.
Wu H (2007) The biology of Australian weeds 49. Conyza bonariensis (L) Cronquist. Plant Protection Quarterly, 22: 122–131.
Wu H, Walker S (2004) Flaxleaf fleabane a difficult-to-control weed in dryland cropping systems associated with zero-tillage. Australian Grain, northern focus iii-v, November-December 2004.
Zelaya IA, Owen MDK, VanGessel MJ (2007) Transfer of glyphosate resistance: evidence of hybridization in Conyza (Asteraceae). American Journal of Botany 94: 660–673.