Gonadal Histopathological Disorders in Mytilus galloprovincialis Male Exposed to Tars Used in Mussel Farms
- 1ECIMAT-Marine Science Station of Toralla, University of Vigo, Vigo, Spain
- 2Department of Biochemistry, Genetics and Immunology, University of Vigo, Vigo, Spain
Polycyclic aromatic hydrocarbons (PAHs) and trace metals are pollutants widely distributed in the marine environment with toxic effects on live organisms, but few laboratory studies have proved their causal relationship with histopathologic alterations from invertebrates. We analyse the histopathologic effects of a tar mixture used in mussel farms on the gonad of male mussels. Mussels were exposed to water-accommodated fractions from different sub-lethal concentrations of that tar mixture during different times. The accumulation of PAHs and metals in mussels was quantified in each one tar exposure condition, and a comparative histological and morphometric analysis of the male gonad was performed, as well as of the gametogenic disorders and the cellular pathologies induced. Spermatogenesis disruption and alterations of somatic and germinal cells were observed as a direct effect of treatment. The pathologies observed followed an increasing malignity sequence depending on dose and exposure time: spawning induction; arrest of spermatogenesis and spermiogenesis; suppression of immune response; dysplasia of Sertoli and connective cells and finally, severe disorders of germinal cells. We demonstrate for the first time that tar used in waterproofing of floating rafts from the mussel farms causes severe effects on male mussel gonad, constituting a direct risk to the health of these organisms, affecting gametogenesis, quality of gametes and, ultimately, the sustainability of this resource and their quality for human consumption. Moreover, we propose the pathologies described in mussel as biomarkers of marine pollution and the mussel gonad as study model of testicular toxicity mechanisms in male reproduction.
Introduction
Galician Rías (NW Spain) are important aquaculture areas, being mussel farming the main activity (Caballero Miguez et al., 2009). However, the pollution level in these areas rises proportionally to the industrial, tourism and port development. Moreover, the same aquaculture activities interact with the environment and can constitute a source of pollution around farms. Mussel culture is carried out in wood floating rafts, whose cleaning, and waterproofing are usually performed in situ with a mixture of petroleum tars and exhausted oil from the diesel engine. This mixture contains high concentrations of polycyclic aromatic hydrocarbons (PAHs) and transition metals (Ruiz et al., 2014), and filtering organisms, such as mussels, can accumulate them to levels well-above those in the surrounding water (Farrington et al., 2016). So, the spillage of these mixtures around rafts entails a direct pollution risk on the immediate environment of farms, which can affect not only the sustainability of the mussel production but also its quality for human consumption.
Polycyclic aromatic hydrocarbons are considered among the most ubiquitous marine pollutants. Hydrocarbons and their biotransformation metabolites have harmful effects on live organisms, mainly the species with high molecular weight and benzene rings number, which have been identified as highly mutagenic, carcinogenic, and teratogenic (Arcos and Argus, 1975; Lehr and Jerina, 1977; White, 1986; IARC, 1989; Nylund et al., 1992; Boström et al., 2002; Baird et al., 2005; Haines and Hendrickson, 2009; Abbas et al., 2013; Poirier, 2016). Heavy and trace metals are also an extensively studied pollutant group in coastal environments because of their wide distribution, persistence, and toxicity. Some of them, such as Mn, Zn, and Cu, have physiological functions in living organisms but at high concentrations are also toxic (Goyer and Clarkson, 2001). Exposition of live organisms to heavy and trace metals has been related to cellular damages, carcinogenesis, and apoptosis (Gerber et al., 1980; Thompson et al., 1989; Chang et al., 1996; Wang and Shi, 2001; Beyersmann and Hartwig, 2008; Obiakor et al., 2010; Tchounwou et al., 2012; Chandel and Jain, 2014; Fatima et al., 2014). These metals are included in diverse industrial compounds, such as fuels and tars, lubricants, catalysts, paint drying agents, pigments, or pesticides (Laws, 2000; Clark, 2001), so pollution is usually occurring by combination of diverse pollutants whose toxic effects may be additive, suppressive or synergistic (Vakharia et al., 2001; Shen et al., 2006; Wang et al., 2015).
The toxic effects of PAHs and heavy metals and their action mechanisms have been widely studied in vertebrates. Among the main pathological effects usually associated with PAHs and heavy metals, are included toxicity reproductive and metabolic alterations and neoplastic diseases. These pathologies result from direct binding of these chemicals and their metabolites to macromolecules and, secondarily, from toxicity of the reactive oxygen species generated in their biotransformation (Sokol, 1987; Mortelmans and Zeiger, 2000; Adhikari et al., 2001; Ercal et al., 2001; Wang and Shi, 2001; Boström et al., 2002; Pufulete et al., 2004; Flora et al., 2008; Ono et al., 2008; Veeramachaneni, 2008; Sanders et al., 2009; Swedenborg et al., 2009; Balabanič et al., 2011; Tchounwou et al., 2012). Also in marine invertebrates, mainly molluscs, there are studies showing reproductive and neoplastic disorders related to pollution (Viarengo et al., 1990; Bolognesi et al., 1999; Matozzo et al., 2001; Creasy and Foster, 2002; Au, 2004; Pichaud et al., 2008; Jing-Jing et al., 2009; Ji et al., 2011; Ruiz et al., 2011). However, in these, much remains unknown about their biotransformation and toxic mechanisms, and few studies have proved their causal relationship under laboratory conditions (Lavado et al., 2006; Ortiz-Zarragoitia and Cajaraville, 2006; Jing-Jing et al., 2009; Schäfer and Köhler, 2009).
In previous papers, our team has documented in Mytilus galloprovincialis the accumulation of PAHs, trace metals and PCBs, relating it with the mutagenicity of seawater, and gonadal neoplasias development (Ruiz et al., 2011, 2013). Likewise, we have previously evaluated the mutagenic capacity of different chemicals used in mussel farms, including the used in the present work (Ruiz et al., 2014). This is a short-term toxicological study in laboratory in order to observe the histopathological effects caused by the more usually tar mixture used in mussel farms on the male gonad of mussel; prove their causal relationship in order to confirm the observed in natural environment; and propose the histological pathologies induced as possible generalist biomarkers of the aquaculture areas quality.
Materials and Methods
Mussels were exposed to a mixture of petroleum tars and exhausted oil the from diesel engine, generally used in cleaning and maintenance of the mussel rafts, which was kindly provided by mussel farmers from the Ría of Vigo. Our team previously analysed the trace metals and hydrocarbons content of this tar, showing a significantly high concentration of Mn, Pb, and Zn (in a proportion 1:2:7), as well as a 81.5% of PAHs species with 3 and 4 benzene rings and a 18.5% of species with 5 and 6 benzene rings (Ruiz et al., 2014).
Mussels, Laboratory Conditions, and Acclimation
Mussels (M. galloprovincialis), 7–8.5 cm long, were collected from floating rafts in the Ría of Vigo in April, before starting the cleaning and waterproofing tasks of rafts in the culture area. Spring is the reproductive season of mussels in this area (Suárez et al., 2005), but also when we observed a higher PAHs accumulation and prevalence of gonadal histological disorders in these organisms in the wild (up to 739 μg kg−1 dry weight and 62.5%, respectively) (Ruiz et al., 2011, 2013). The reproductive stage seems, therefore, constitute the most sensitive life-stage of mussels and the most suitable to test the toxic effects of pollutants on gametogenesis (Diepens et al., 2017). In the laboratory (Station of Marine Science of Toralla, University of Vigo), mussels were acclimated during 15 days in two polythene tanks of 150 L with 20 μm filtered seawater, in a semi-open system of 2 mL min−1 flow and the same out-flow, under natural photoperiod, and constant aeration, temperature and salinity. Animals were fed every 2 days with a microalgal diet (0.0224 g per mussel) composed by Isochrysis galbana, Tetraselmis suecica, and Chaetoceros gracilis in a proportion of 50:30:20.
Preparation of Water-Accommodated Fractions (WAF)
During waterproofing of rafts, a lot of tar falls to water and farmers pour the tar leftovers in the same environment, so high tar concentrations can be achieved around farms. Nevertheless, the dilutive effect of tides and currents makes their lasting become not too prolonged in time. Only the soluble fraction of these compounds, which varies depending on their concentration and composition, is bioavailable and may exert actual toxicity on aquatic organisms (Singer et al., 2001). The WAF from different concentrations of tar were prepared in 20 μm filtered seawater, following the recommendations by CROSERF (Chemical Response to Oil Spills: Ecological Research Forum) (Singer et al., 2000, 2001; Aurand and Coelho, 2005). Briefly: in a similar way as carried out by the mussel farmers, the tar was heated to make it more fluid. Then, each tar concentration to be tested was prepared in glass Mariotte bottles with 5 L of seawater and maintained at room temperature for 24 h under magnetic stirrer. At the beginning of the experiments, the WAFs from the different tar concentrations were similarly prepared in polythene tanks of 30 L.
Determination of the Median Lethal Concentration (LC50)
Based on preliminary experiments, the WAF from 6 concentrations of tar between 60 and 160 mg L−1 were used in order to determinate its LC50 in an acute toxicity test for 96 h. For mortality test, acclimated mussels were randomly distributed in polythene tanks of 30 L (40 individuals/tank), containing the WAF from 60, 80, 100, 120, 140, and 160 mg L−1 tar prepared as above described. Five litres WAF from each tar concentration was prepared daily and supplied to the corresponding tank, at a 2 mL min−1 flow, replacing the seawater entry. The assay was performed in duplicate (6 × 2 tanks), and the mussels from other two tanks were maintained in seawater and taken as reference. Conditions of water flow, nutrition, temperature, aeration, and photoperiod were the same as during acclimation. Water quality in each tank was daily checked by measuring pH, dissolved oxygen (DO), salinity and ammonium concentration, using portable pH meter, oximeter and salinometer, and ammonium kit LCK 304, respectively.
Mortality data were analysed by the PROBIT regression test with IBM SPSS Statistics 23.0 package (IBM Corporation, Armonk, NY) which allowed calculating the LC50 of the tar mixture for the mussels, and their associated 95% confidence limits. The adequacy of the probit model to the observed data was evaluated by Chi-square test.
Toxicological Assay
Three sub-lethal WAF from tar concentrations were selected below the LC5 value (see result section about LC50 and probit test) to conduct this study: 10, 40, and 60 mg L−1. The WAF of these tar concentrations, the tanks and the mussels were prepared and disposed of as described in the above sections. The toxicological assay was also performed in duplicate. Two tanks with mussels maintained in seawater without tar were taken as reference. The conditions of the water and the mussels, as well as quality system control, were the above described. The toxic treatment lasted 17 days and samples were taken at 0, 10 and 17 days. After this time, mussels exposed to the WAF from 60 mg L−1 tar were transplanted to the other two tanks under non-toxic conditions during 2 weeks before sampling (depuration time). The analysis power of the sample size (320 individuals/4 groups of study) was validated with free software G*power (http://www.psychologie.hhu.de/arbeitsgruppen/allgemeine-psychologie-und-arbeitspsychologie.html), giving a value of 0.96. In each sampling, 20 individuals from each treatment (10 individuals per tank) were collected and, by histological analysis, males were separated for this study.
Biometric Analysis
In each sampling, the size, total weight (Wt), shell weight (Ws), and mantle weight (Wm) of individuals were measured. From these data, the gonadal condition index (GCI) was calculated. This index is an approximation to gametogenic development of an organism. In species of the genus Mytilus, the genital papilla proximity to the mantle tissue is used for the expansion of gonad, and the gametogenic development takes place in this tissue. So, in these species, the GCI is determined as the mantle condition index, which can be calculated as the ratio between the mantle weight and the weight of all soft tissues (the difference between total weight and shell weight) (Suárez et al., 2005).
Histological and Morphometric Analysis
A cross-section (1 cm wide) of the central part of one hemimantle of each mussel was fixed in 10% neutral buffered formalin, dehydrated and embedded in paraffin. Sections 5 μm thick were cut, mounted on microscope slides, and stained with haematoxylin and eosin. Additional slides were stained with modified Gomori methenamine-silver nitrate, aniline blue and periodic acid-Schiff (PAS) in order to detect basement membranes and reticulin fibres, basophilic components, and glycogen, respectively.
The slides were examined under a light microscope Nikon 90i Eclipse. Representative photomicrographs were captured by a high-resolution camera digital (Nikon DS-Fi1c) coupled to a microscope and using the image analysis system Nis-Elements BR 4.0 software (Nikon Instruments Inc., Melville, NY). Morphometric analyses were performed using the same image analysis system above: the number of gonadal follicles, vesicular cells (VC), adipogranular cells (ADG), and granulocytes, as well as the area of gonadal follicles and VCs, were quantified in 20 sections 100 μm2 from each slide at 100 or 400x magnification (for gonadal follicles and cells, respectively). Composition of figures was performed using CorelDraw® Graphics Suite X5 (Corel Corporation, Canada).
PAHs and Metals Extraction and Quantification
After dissection for histological analysis, soft tissues of the individuals sampled from each treatment was pooled, quickly frozen, and pulverised in liquid nitrogen using a laboratory ball mill (Fritsch GmbH, Germany). The analyse of the PAHs and trace metals levels were contracted to the Centre for Scientific and Technological Support to Research (CACTI) of the University of Vigo. PAHs were extracted with methanol in ultrasonication bath for 30 min and separated and quantified by high-performance liquid chromatography (HPLC) within tandem detectors of photodiode array and scanning fluorescence. The 16 most abundant species of PAHs in the tar composition were measured: naphthalene (Nap), acenaphthylene (Acy), acenaphthene (Ace), fluorene (Flu), phenanthrene (Phe), anthracene (An), fluoranthene (Flt), pyrene (Py), benzo(a) anthracene (BaA), chrysene (Chry), benzo(b)fluoranthene (BbF), benzo(k)fluoranthene (BkF), benzo(a)pyrene (BaP), dibenzo(a,h) anthracene (DBA), benzo(g,h,i)perylene (Bper), and indeno—(1, 2, 3,-c,d) pyrene (IP). The most abundant trace metals in tar (Zn, Pb, and Mn) were determined by optical emission spectroscopy with inductively coupled plasma as the excitation source (ICP-OES) after a hydrogen peroxide:nitric acid (2:5) digestion. All analyses were performed in triplicate and PAHs and metals concentrations were expressed as μg kg−1 fresh weight (fw) and mg kg−1 fw, respectively.
Statistical Analysis
Statistical analyses were performed with IBM SPSS Statistics 23.0. All histological preparations were comparatively examined in relation to the reference organisms. The distribution and homoscedasticity of biometric and morphometric data were analysed by Kolmogorov-Smirnov and Levene tests, respectively. Data were assessed to one-way analysis of variance (ANOVA) followed by Tukey's multiple comparison test or Student's t-test to determine the statistical significance between groups. p < 0.05 was considered statistically significant.
Multivariate analysis was carried out using the software packages PRIMER6 & permanova + (Anderson et al., 2008). The distribution of the samples based on quantitative parameters variation as the effect of tar exposure was examined by Hierarchical Cluster analysis using Bray Curtis similarity. Previously, the collinearity between variables was analysed by Pearson correlation matrix, eliminating the variables with r2 > 0.95. The main variables explaining this distribution were identified by parsimonious models (DistLM), and represented by distance-based redundancy analysis (dbRDA) (Anderson et al., 2008).
Results
Water Quality and LC50 Determination
The water quality parameters remained within a narrow range in all tanks throughout the mortality test and the toxicological experiment: 18 ± 1°C; 7.8 ± 0.4 pH; 34.5 ± 0.5%0 salinity; 87.5 ± 5% dissolved oxygen, and 0.03 ± 0.01 mg L−1 ammonium. So, the differences we described in mussels intoxicated relative to reference mussels could be considered as a consequence of tar exposition.
Survival of the reference mussels and those exposed to the WAF from 60 mg L−1 tar was 100%. However, mortality of the mussels exposed to WAF from tar concentrations higher than 80 mg L−1 increased as tar concentration increased. The log-probit regression analyse of the data allowed estimate the LC50 of the tar in 139.84 mg L−1 for M. galloprovincialis (95% confidence interval: 132.57–149.08 mg L−1; regression equation: Y = 0.028X−3.922, Chi-square = 0.182, P = 0.67). In the same analyse, the LC5 (tar concentration whose WAF cause a 5% mortality of mussels) yields a value of 81.20 mg L−1 (95% confidence interval: 64.02 – 92.23 mg L−1). The toxicological assay was then performed with the WAF from tar concentrations below this value.
PAHs and Metals Accumulation
Pollutants accumulation in the mussels exposed to tar increased significantly (p < 0.05) in relation to reference mussels within the following ranges: 6–106 μg kg−1 fw total PAHs (∑16 parent PAHs); 0.08–0.71 mg kg−1 Mn; 0–1.4 mg kg−1 Pb; and 12–75 mg kg−1 Zn (Table 1). The total PAHs accumulation increased proportionally to tar concentration and exposure time in the mussels subjected to 10 and 40 mg L−1 of tar. However, in mussels exposed to 60 mg L−1 tar, the accumulation falls concerning that of the exposed to 40 mg L−1 tar in both two exposure times. Moreover, contrary to expected based on tar composition, the hydrocarbons with 5 and 6 benzene rings were accumulated in greater proportion (up to 30.8%) than species of 3 and 4 benzene rings. The mussels that were transplanted to non-toxic conditions for 2 weeks, after exposure to the WAF from 60 mg L−1 tar during 17 days, showed a small, but significant decrease (p < 0.05) in the accumulation of PAHs of 5 and 6 benzene rings and metals, but not of hydrocarbons of 2, 3, and 4 benzene rings.
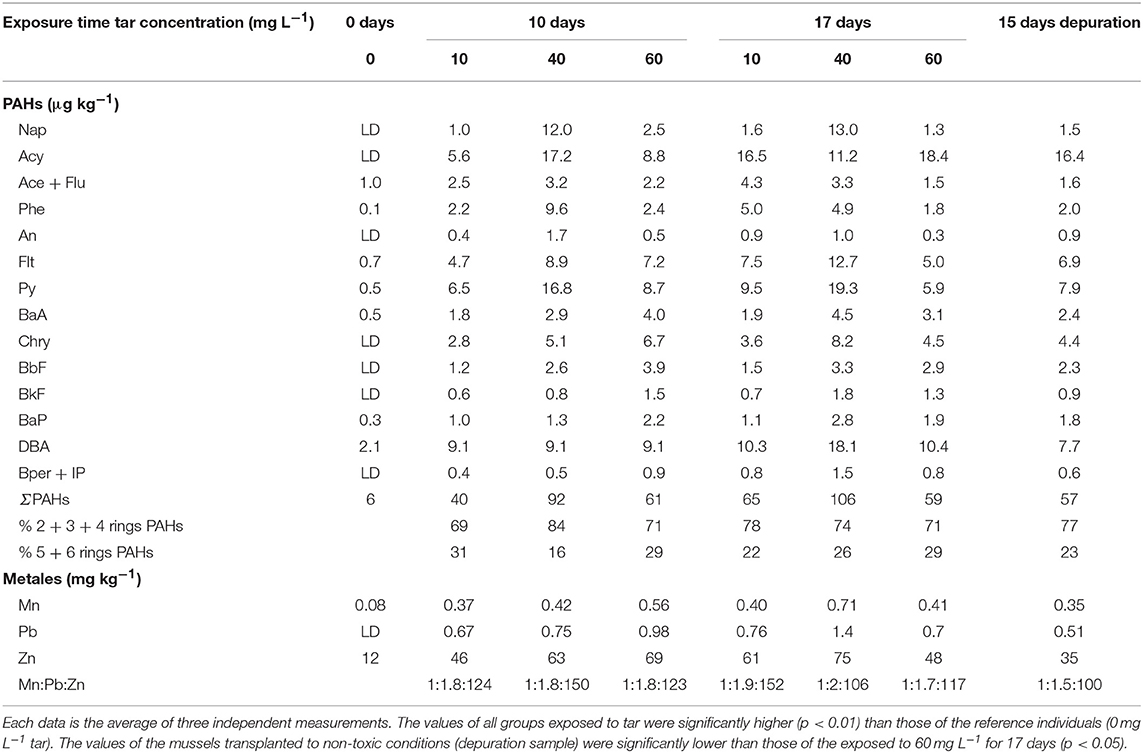
Table 1. Accumulation of hydrocarbons, Mn, Pb, and Zn in Mytilus galloprovincialis exposed to different tar concentrations and exposure times.
The heavy metals followed a similar pattern of accumulation, increasing proportionally with the tar concentrations supplied and exposure times (p < 0.05). Only the mussels exposed to the highest tar concentration during longer time showed a decrease in metals accumulation. Unlike the proportion of the metals in the tar composition, the ratio Mn:Pb:Zn in the mussels exposed to tar was around 1:2:>100. However, the Zn and Pb proportion decreased significantly (p < 0.05) both, in mussels exposed for 17 days to 40–60 mg L−1 and 60 mg L−1 tar, respectively, as in the mussels transplanted to depuration conditions for 15 days.
Biometric Analysis and Gonadal Condition Index
Biometric and histological analysis was performed individually in each one of 80 mussels collected in each sampling. The histological analysis allowed separating the males for this study. The ratio between sexes was almost 1.2 in all sampling, similar to that found for Mytilus edulis and M. galloprovincialis by others authors in the wild (Lubet, 1959; Villalba, 1995; Suárez et al., 2005). All male mussels showed similar size (7.05 ± 0.45 cm) and shell weight (8.45 ± 0.8 g) along the experiment. However, the total weight of soft tissues ranged between 15.98 and 5 g, and the mantle weight between 5.68 and 0.18 g, decreasing significantly as tar concentration and exposure time increased (p < 0.01; Figures 1A,B). The mantle tissue of Mytilus has two interrelated physiologic functions: storage of reserve substances in specialised cells and gametogenesis at the expense of these reserves (see Supplementary Materials, “Mantle tissue of Mytilus galloprovincialis: histological structure”). So, a priori, the significant fall in mantle tissue weight could suggest an alteration of these two functions.
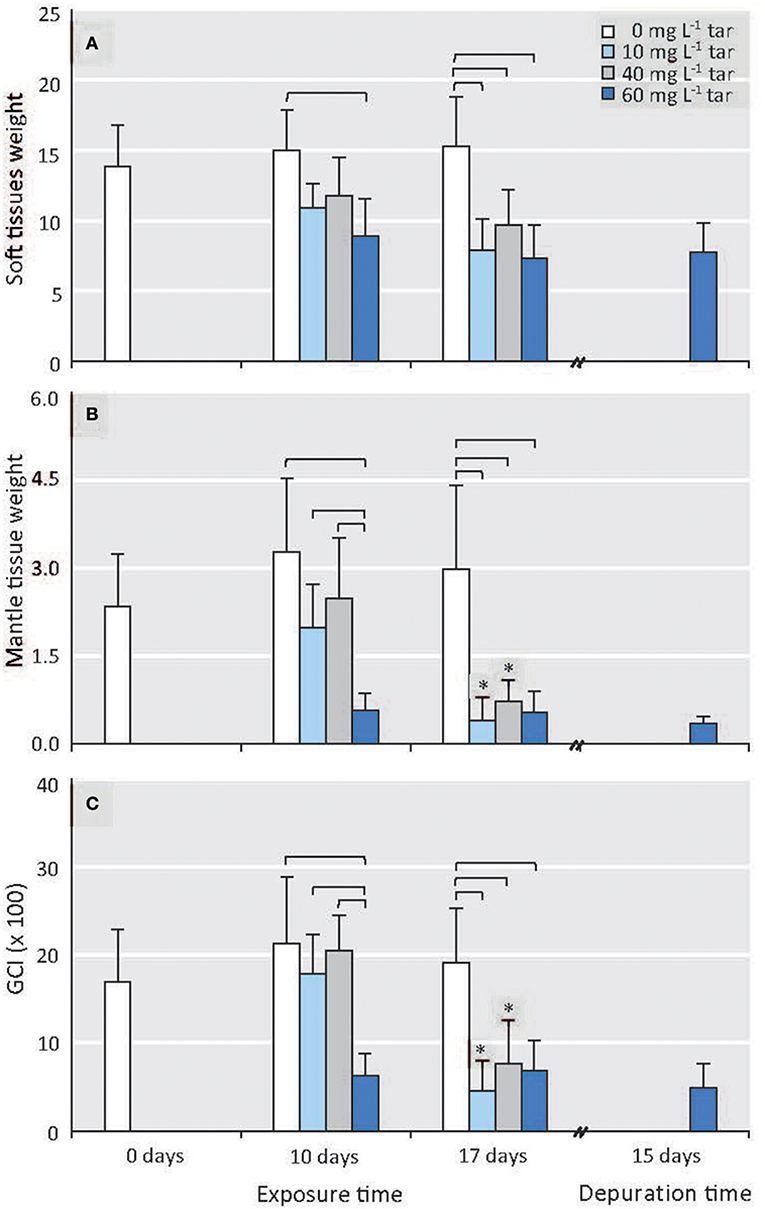
Figure 1. Effect of different tar concentrations and exposure times on biometric parameters. (A) Soft tissues weight. (B) Mantle tissue (gonad) weight. (C) GCI. Data are means ± SD, n ≥ 8. Reference mussels (0 mg L−1 tar). Square brackets represent significance differences between mussels exposed to different tar concentrations during same time (p < 0.001). Significance differences between mussels exposed to each of the tar concentrations at different times: *p < 0.01.
The GCI from the reference individuals was similar throughout the experiment (Figure 1C). The mussels exposed to 10 and 40 mg L−1 of tar mixture for 10 days showed negligible alterations (p > 0.05) of mantle and soft tissues weights, as well as similar GCI values, compared to those of the control mussels. However, the mussels exposed to 60 mg L−1 tar showed a significant decrease in the mantle weight and, consequently, of the GCI (p < 0.01; Figures 1B,C). After 17 days' tar exposure, the mantle tissue weight and the GCI of the mussels exposed to 10 and 40 mg L−1 tar fell (p < 0.01) until similar values as those of the mussels exposed to 60 mg L−1 tar during 10 days; but the mussels exposed to this last concentration showed similar values to those achieved after 17 days of exposition (Figures 1B,C).
Gonadal Stage and Histological Disorders
At the onset of the toxicological assay, the acclimatized mussels were in a pre-spawn stage (see Figure S1). This gametogenic stage is characterised by the presence of large gonadal follicles full of ripe gametes occupying the whole mantle tissue and by a scarce storage tissue, mainly formed by vesicular cells (see Supplementary Materials, “Reproductive cycle of Mytilus galloprovincialis”). Throughout all experiment, the reference individuals showed this same stage, with a slight but significant increase of gonadal follicles number (p < 0.01) parallel to decrease of vesicular cells (VC) area and adipogranular cells (ADG) number (p < 0.0001; Figures 2A1,A2, 3A–E). In the follicular wall, auxiliary cells and some spermatogonias, spermatocytes and spermatids can be observed (see Figures S2A,B). At 17 days, auxiliary cells and eosinophilic granulocytes were observed in the lumen of some follicles from the reference mussels (see Figures S2C,D; Figure 3F). In the seminiferous tubules, these two cellular types have a phagocytic function of cellular debris after the spawn. In bivalves, the increase in temperature is one of the main natural factors inducing spawning (Suárez et al., 2005), but during our experiment, the temperature was kept constant at 18°C. So, the lack of stimuli for spawn could have caused atresia of the over-riped gametes, inducing the phagocytic function of auxiliary cells and the increase in the number of eosinophilic granulocytes in the follicular lumen (Figure 3F) as it is described in the wild under normal conditions (Suárez et al., 2005; Suárez Alonso et al., 2007).
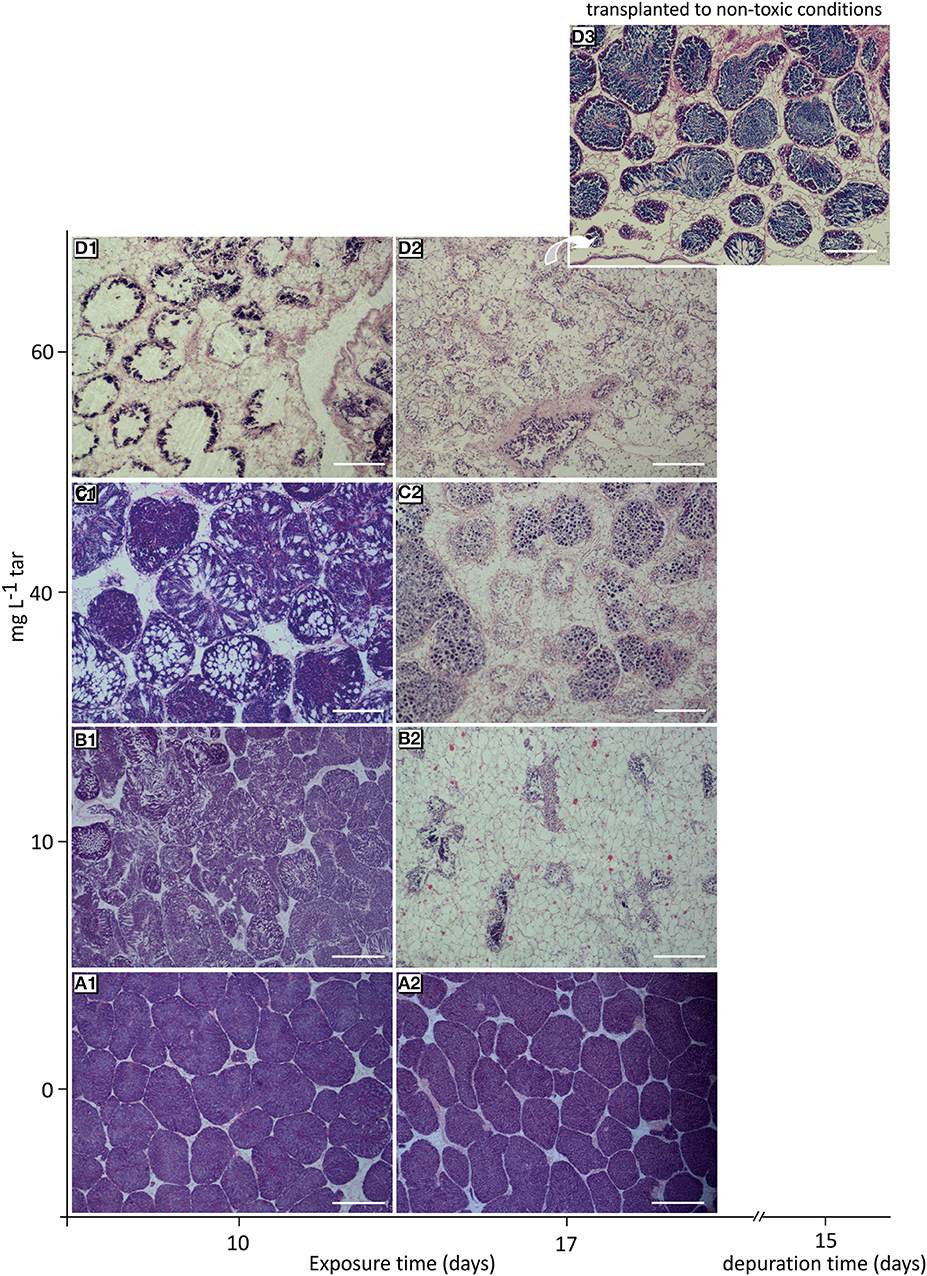
Figure 2. Histological overview of the gonad from mussels exposed to different tar concentrations for 10 and 17 days. (A1,A2) Reference mussels (0 mg L−1 tar) showing a typical pre-spawn stage. (B1) Spawn induction. (B2) Atrophy of gonadal follicles in mussels exposed to 10 mg L−1 tar. (C1) Sloughing of spermatids. (C2) Loss of radial architecture of germinal epithelium and hyalinisation of basement membrane of follicles in mussels exposed to 40 mg L−1 tar. (D1) Disappearance of advanced germ cells. (D2) Virtually empty gonadal follicles with hyperchromatic spermatocytes and proliferation of storage tissue cells in mussels exposed to 60 and 80 mg L−1 tar. (D3) Gametogenesis reactivation in mussels transplanted to non-toxic conditions for 17 days after exposition to 60 mg L−1 tar. Haematoxylin and eosin stain. Scale bar: 500 mm (A1,B1), 200 mm (B2–D3).
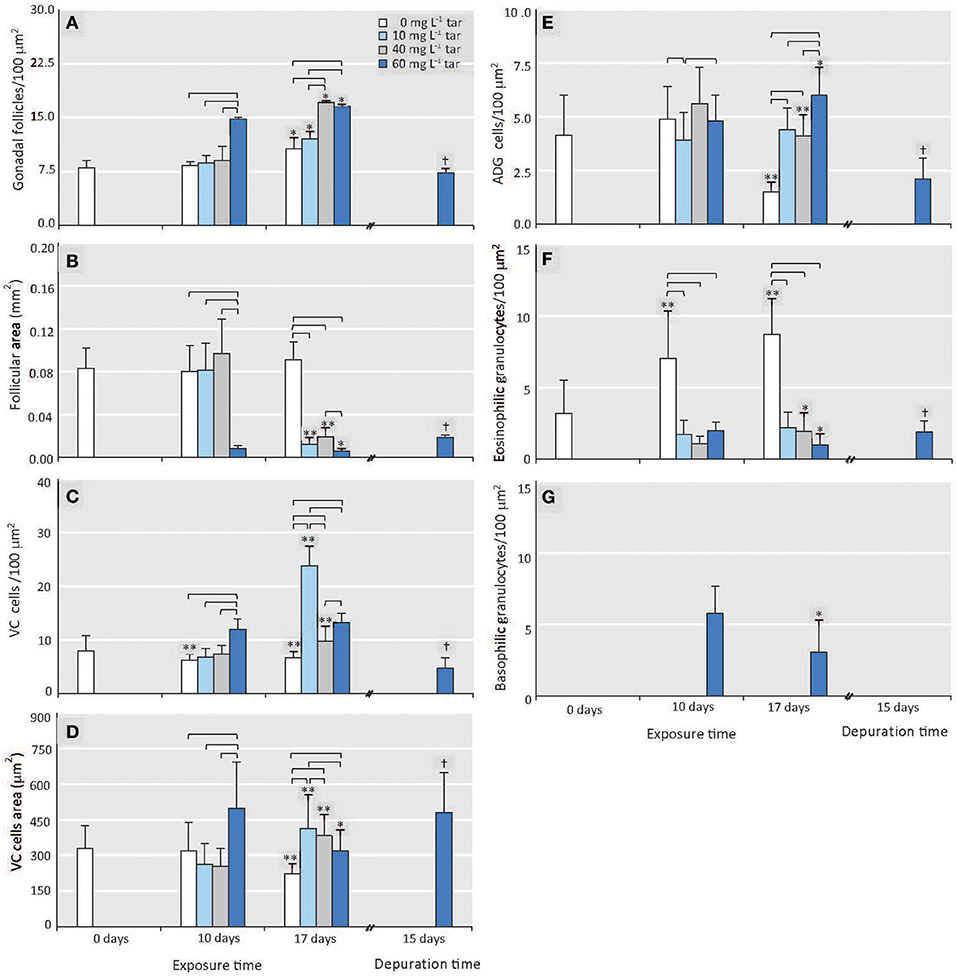
Figure 3. Effect of different tar concentrations and exposure times on histological morphometric parameters. (A) Number of gonadal follicles. (B) Area of gonadal follicles. (C) Number of VC cells. (D) Area of VC cells. (E) Number of ADG cells. (F) Number of eosinophilic granulocytes. (G) Number of basophilic granulocytes. Data are represented as means ± SD, n ≥ 8. Reference mussels (0 mg L−1 tar). Square brackets represent significance differences between mussels exposed to different tar concentrations during same time (p < 0.001). Significance differences between mussels exposed to each of the tar concentrations at different times: *p < 0.01, **p < 0.0001. Significance differences between mussels transplanted to non-toxic conditions for 15 days after exposition to 60 mg L−1 tar for 17 days: †p < 0.001.
The number and area of gonadal follicles from mussels exposed to 10 and 40 mg L−1 tar for 10 days were unchanged in relation to those of the reference individuals (Figures 3A,B). However, lumen was partially empty, and spermatozoids were present in gonoducts, evidencing clear images of spawn (Figures 2B1,C1). Mussels exposed to 40 mg L−1 tar also showed evidence of sloughing of spermatids or emission of immature germinal cells (Figure 2C1). After 17 days, spawn had been massive in most individuals, and atrophy of gonadal follicles was observed, decreasing their size and increasing their number per area (Figures 2B2,C2, 3A,B). The basement membrane of follicles appeared thickened acquiring a hyalinised, appearance and the germinal epithelium lost its characteristic radial architecture (Figures 2B2,C2). Simultaneously, storage tissue was increased, mainly due to the proliferation and hypertrophy of VC cells (Figures 3C–E). The mussels exposed to 60 mg L−1 tar for 10 or more days showed similar histological morphometric parameters to these described in mussels exposed to 10 and 40 mg L−1 tar for 17 days, but in a more obvious way (Figures 3A–E). Besides, these individuals showed virtually empty gonadal follicles, devoid of advanced germ cells (spermatids and spermatozoids), with scarce and hyperchromatic spermatocytes, and staining of the storage tissue seemed cloudy and diffuse (Figures 2D1,D2). Concerning to immune cells, all concentrations of tar assayed caused a significant decrease of eosinophilic granulocytes (p < 0.001; Figure 3F), but aggregates of basophilic granulocytes were observed in the mussels exposed to 60 mg L−1 tar (Figure 3G). More than 80% of the individuals exposed to each one of the tar concentrations assayed showed the histological alterations described.
Cellular Pathological Features
The typical aspect of the different cellular types constituting the mantle tissue of Mytilus galloprovincialis is shown in the Figure S2 (see Supplementary Materials, “Mantle tissue of Mytilus galloprovincialis”), which includes representative photomicrographs of the reference individuals from this work. Exposure of mussels to 10 mg L−1 tar for 17 days and 40 mg L−1 tar during 10 days did not cause observable cellular alterations. However, higher concentrations and longer exposure times induced cellular disorders of increasing intensity and malignity in 75–82% of individuals.
After treatment with 40 mg L−1 of tar for 17 days and with 60 mg L−1 during 10 or more days, substantial changes were observed in somatic cells from the storage tissue. The amorphous extracellular matrix increased and collagen synthesis by fibroblasts and myofibroblasts was stimulated in a possible fibrotic reaction. The fibrosis originated entailed the hyalinisation of tissue, mainly in the basement membrane of the gonadal follicles, which appeared thickened and occasionally broken (Figures 4A,B). The VC cells displayed hypertrophy and hyperplasia (Figures 3C,D, 4). Their size and number increased as the accumulated glycogen decreased, and their nucleus acquired a rounded shape and a more central position (Figure 4). The ADG cells changed their morphology, their size and number also increased abnormally, and their cytoplasm lost its eosinophilic character, acquiring a foamy aspect with refringent microvesicles, probably lipidic, and small PAS-positive granules (Figure 4). As cited above, the number of eosinophilic granulocytes decreased significantly in all mussels exposed to tar. Nevertheless, in individuals exposed to 60 mg L−1, the occurrence of another type of granulocytes with a small and slightly basophilic cytoplasm, clustered around the gonadal follicles was observed (Figure 4C).
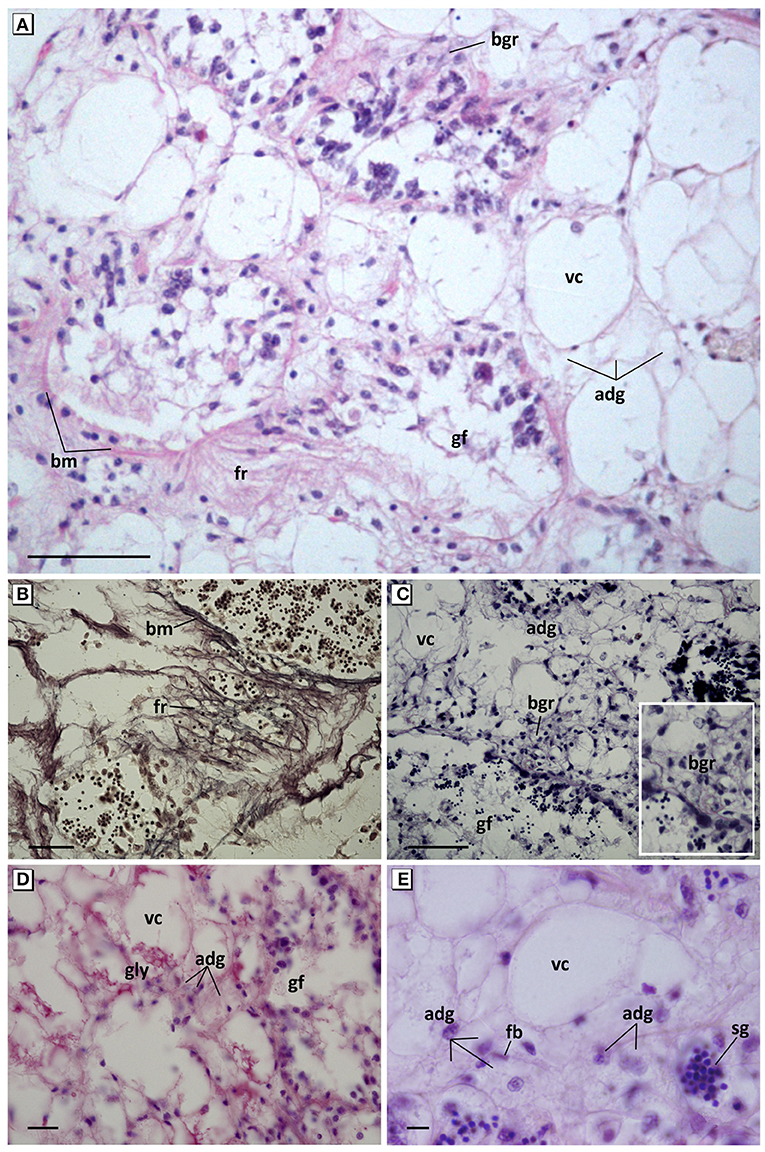
Figure 4. Pathological features of connective tissue cells. (A) Overview of hyperplasia, fibrotic reaction of connective tissue, and basophilic granulocytes. Haematoxylin and eosin stain. (B) Detail of reticulin fibers and the thickening and break of the basement membrane of the gonadal follicles. Gomori methenamine-silver nitrate stain. (C) Hypertrophy and hyperplasia of VC and ADG cells; appearance of cluster of basophilic granulocytes around the gonadal follicles. Aniline blue stain. (D) Decrease of accumulated glycogen in VC cells and increase of small granules in ADG cells. PAS stain. (E) Foamy aspect with refringent microvesicles and loss of eosinophilia of ADG cells. Haematoxylin and eosin stain. fr, Fibrotic reaction; fb, fibroblast; bm, basement membrane; adg, adipogranular cells; vc, vesicular cells; gly, glycogen; bgr, basophilic granulocytes; sg, spermatic granuloma invading connective tissue; gf, gonadal follicle. Scale bar: 50 μm (A,C), 25 μm (B), 10 μm (D,E).
In gonadal follicles, a disorganisation of the germinal epithelium was observed, with loss of germline and total absence of spermatozoa, manifesting a maturation arrest and spermatogenesis failure (Figures 5A,B). In the most of the follicles from mussels exposed to 40 mg L−1 tar for 17 days, the only germinal cells present were abnormal aggregates of spermatids showing a large number of hyperchromatic nuclei immersed in one lightly eosinophilic cytoplasm, likely due to an incomplete cytoplasmic division (Figures 5A,C). In many cases, these aggregates filled the follicular lumen, and occasionally were seen invading the connective storage tissue (Figure 4E), like the spermatic granulomas described in vertebrates (Creasy et al., 2012; Greaves, 2012). Follicles from mussels exposed to 60 mg L−1 tar for 10 days only contained spermatocytes, sometimes also forming aggregates, many of them with condensed chromatin in a peripheral position, evidencing fragmentation of the nucleus (karyorrhexis) and apoptotic processes (Figures 5D,F). In both cases, apoptotic bodies (fragments intensely stained by haematoxylin within a round mass of intensely eosinophilic cytoplasm) of spermatids or spermatocytes were observed into the cytoplasm of auxiliary cells. These cells (Sertoli-like cells) showed hypertrophy and hyperplasia, and their nucleus changed their original basal position elongating toward the follicular lumen perpendicularly to the follicular membrane. Their cytoplasm appeared swollen and separated from the membrane until remain free in the follicular lumen, showing an intense phagocytic activity of apoptotic germ cells (Figures 5E,F). By contrast, the phagocytic response of haemocytes was minimal and very few eosinophilic granulocytes were observed in the follicular lumen. Moreover, Sertoli cells with pleomorphic aspect and swollen and vacuolated cytoplasm filled a large number of gonadal follicles (Figures 5G,H). These morphological and positional changes of Sertoli cells hindered their quantification.
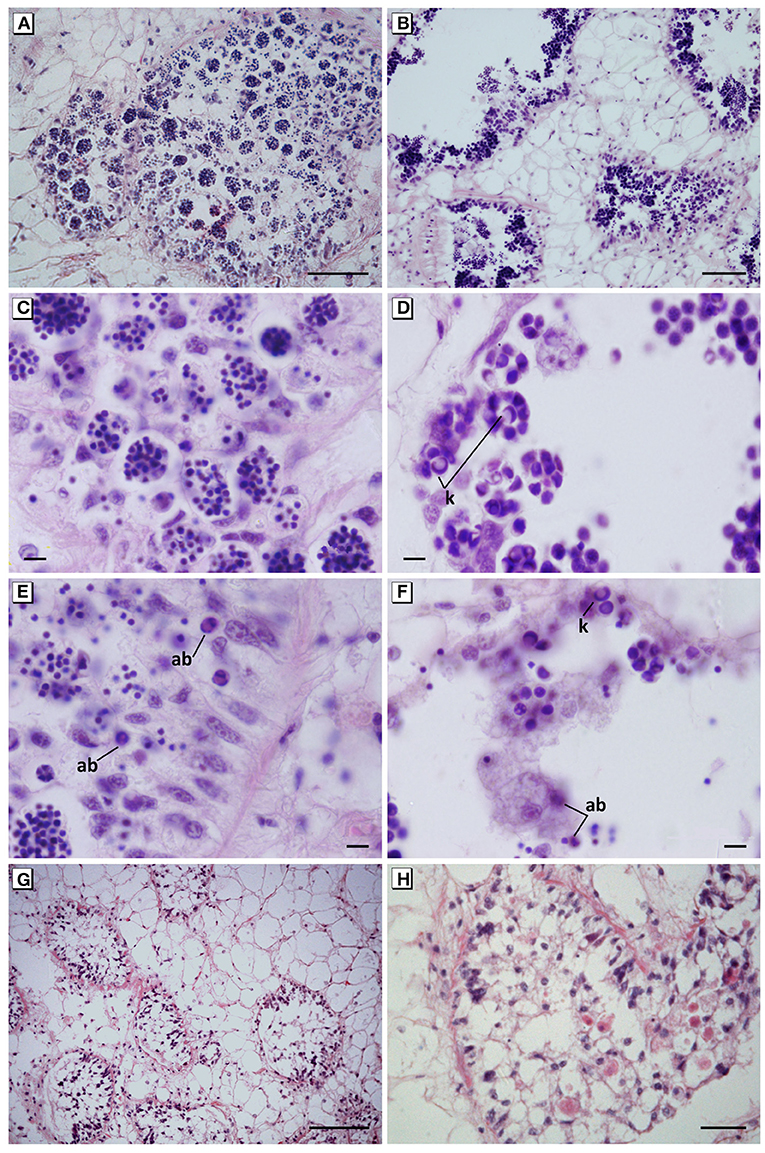
Figure 5. Maturation arrest of the germinal cells and spermatogenesis failure. (A,C) Disorganisation of the germinal epithelium and abnormal aggregates of hyperchromatic spermatids in mussels exposed to 40 mg L−1 for 17 days. (B,D) Disorganisation of the germinal epithelium and abnormal aggregates of apoptotic spermatocytes showing karyorrhexis in mussels exposed to 60 mg L−1 for 10 days. (E) Hypertrophy and hyperplasia of auxiliary cells (Sertoli-like cells) with an intense phagocytic activity of apoptotic spermatids in mussels exposed to 40 mg L−1 tar for 17 days. (F) Auxiliary cells in the lumen follicular engulfing apoptotic spermatocytes in mussels exposed to 60 mg L−1 for 10 days. (G,H) Hyperplasia of pleomorphic and vacuolated auxiliary cells filling the lumen follicular in mussels exposed to 60 mg L−1 for 10 days. In all cases, a total absence of spermatozoa in mussels was evident. Haematoxylin and eosin stain. ab, Apoptotic bodies; k, karyorrhexis. Scale bar: 100 μm (B,G), 50 μm (A), 25 μm (H), 10 μm (C–F).
The most severe disorders caused by the tar mixture on the germinal cells were observed in mussels exposed to 60 mg L−1 for 17 days. In these mussels, auxiliary (Sertoli-like) cells were not observed, or their number decreased drastically. In most individuals, the gonadal follicles were virtually empty, showing large mononucleate and multinucleated germinal cells with prominent and irregular hyperchromatic nuclei (pyknosis), karyorrhexis, and karyolysis (Figure 6A). The gonadal follicles of some others individuals (30% of mussels) showed microcytic germ cells with hyperchromatic irregular nuclei, clear cytoplasm and blurred edge, forming encapsulated nodules which also invaded the connective storage tissue. These encapsulated nodules showed fibrosis and deposits PAS-positive, evidencing glycogen accumulation (Figures 6B–D).
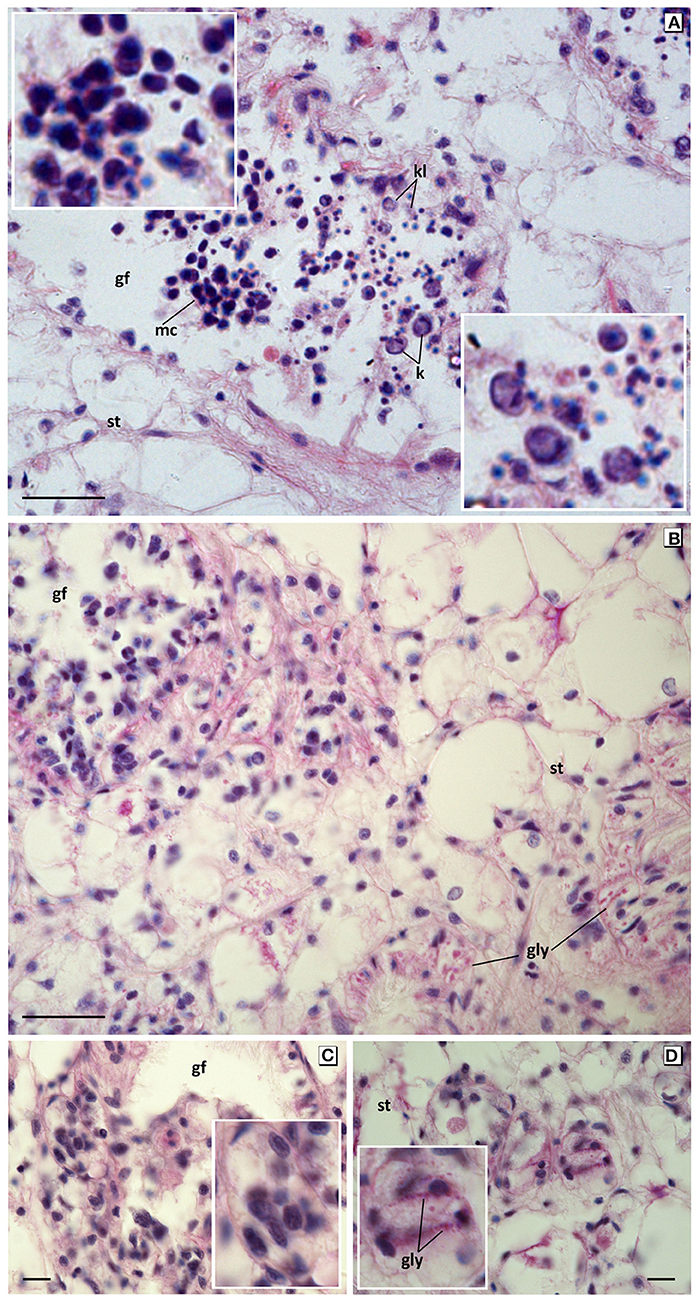
Figure 6. Apoptotic and degenerative disorders in germinal cells from mussels exposed to 60 mg L−1 tar for 17 days. (A) Large mononucleate and multinucleated germinal cells whit big and irregular pyknotic nuclei (top inset), karyorrhesis and karyolisis (bottom inset). Haematoxylin and eosin stain. (B–D) Microcytic germ cells with hyperchromatic irregular nuclei, clear cytoplasm, and blurred edge forming encapsulated nodules, with deposits PAS positive in gonadal follicles and invading the connective tissue. PAS stain. Gf, Gonadal follicle; st, storage tissue; gly, glycogen; k, karyorrhexis; kl, karyolysis. Scale bar: 25 μm (A,B), 10 μm (C,D). Insets: 2x magnification in base image.
Depuration Assay
The mussels transplanted to non-toxic conditions for 2 weeks after exposure to WAF from 60 mg L−1 tar for 17 days, showed signs of an incipient reversion of the above-described disorders (Figure 7). The mantle weight and the GCI were maintained at similarly low values (Figure 1). However, a significant increase of follicular area took place, decreasing the number of the gonadal follicles per area (Figures 2D3, 3A,B). Spermatogenesis was reactivated, while the number of vesicular and adipogranular cells decreased significantly (p <0.001; Figures 2D3, 3C–E). Furthermore, aggregates of basophilic granulocytes disappeared at the same time as eosinophilic granulocytes increased significantly (p <0.001; Figures 4F,G). The appearance of the storage connective tissue cells was similar to those in the reference individuals, and the ADG cells started again to acquire their characteristic eosinophilia; although the VC cells area remained high and their round nucleus indicated a low glycogen accumulation. However, disorganisation and discontinuity of the germinal epithelium and some disorders of germinal cells, as aggregates of multinucleated and pyknotic spermatocytes were still evident (Figures 2D3, 7).
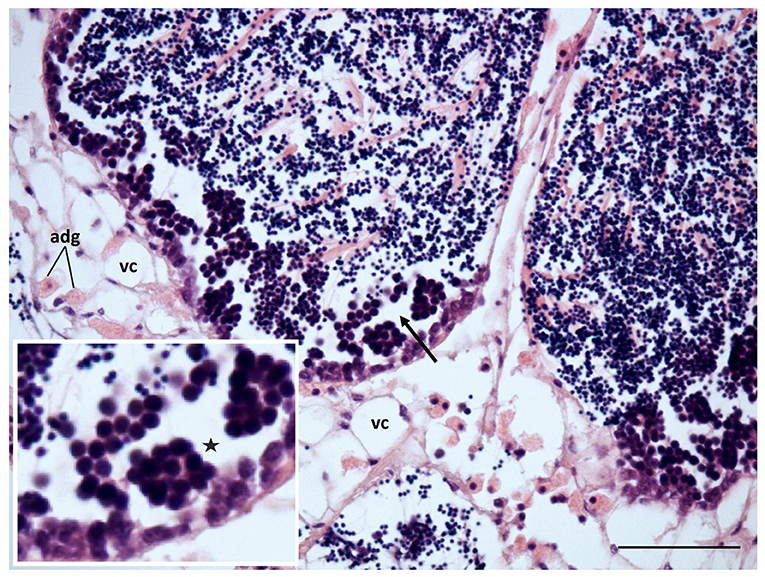
Figure 7. Gametogenesis reactivation and recovery of the connective tissue in mussels transplanted to non-toxic conditions after exposition to 60 mg L−1 tar for 17 days. adg, Adipogranular cells; vc, vesicular cells; discontinuity in the germline (*), and aggregates of large and pyknotic spermatocytes (arrow) can still be observed. Scale bar: 50 μm. Inset: 2x magnification in base image.
Multivariate Analysis of the Quantitative Parameters
The Pearson correlation analysis identified a high collinearity (r2 >0.95) between the PAHs and metals accumulation, and the other hand between the weight of soft tissues and mantle, GCI and follicular area. So, in the Hierarchical Cluster and DistLM analysis, the data of metals accumulation, the weight of soft tissues and mantle and follicular area were not considered.
Cluster analysis showed two significantly different groups. The first group comprised two subgroups: one included reference mussels and the other those exposed to low and medium tar concentrations for 10 days. The second group included the mussels exposed to the highest concentration for 10 and 17 days into a subgroup, and those exposed to low and medium concentrations for 17 days in other. The mussels transplanted to non-toxic conditions for 2 weeks (depuration assay) were included in the second group, although at a considerable distance of mussels exposed to medium and high doses tar. The best variables combination determined by DistLM analyses excluded the GCI, and the first two dbRDA axes explained the 87.7% of the fitted variability (Figure 8).
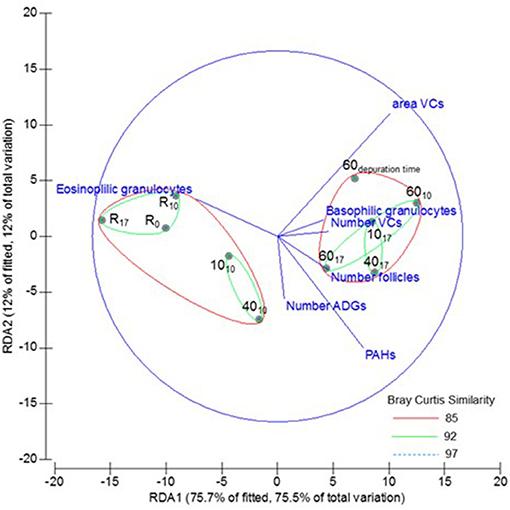
Figure 8. Multivariate analysis: cluster analysis overlayed to dbRDA based to the fitted model selected by Bray-Curtis similarity of chemicals accumulation, and biometric and morphometric variables from Mytilus galloprovincialis, exposed to different tar concentrations during 0, 10, and 17 days (subscripts). R, Reference mussels.
Discussion
Toxicological studies have demonstrated that hydrocarbons disrupt multiple endocrine pathways affecting hormonal regulation, metabolism and reproduction, and that several congeners have mutagenic effects contributing to carcinogenesis (Creasy and Foster, 2002; DeFur, 2004; Oetken et al., 2004; Pufulete et al., 2004; Dietrich and Krieger, 2009; Sanders et al., 2009; Balabanič et al., 2011; Creasy et al., 2012; De Falco et al., 2015).
Heavy metals have also been related to cellular injury, metabolic disorders, and DNA and proteins damage, that may lead to cell and reproductive cycle disturbance, carcinogenesis, or apoptosis (Gerber et al., 1980; Ronis et al., 1996; Wang and Shi, 2001; Tchounwou et al., 2004, 2012; Beyersmann and Hartwig, 2008; El-Sayed and El-Neweshy, 2010; Obiakor et al., 2010; Ebrahimi and Taherianfard, 2011). Although in invertebrates the number of these studies is lower, and these organisms present different susceptibility to pollutants, several authors have suggested similar effects and mechanisms than that of vertebrates and have propose them as models in endocrine disruption research (Viarengo et al., 1990; Bolognesi et al., 1999; Matozzo et al., 2001; DeFur, 2004; Porte et al., 2006; Zhou et al., 2009; Fatima et al., 2014).
PAHs and Metals Accumulation
Applying, where it was necessary, the conversion suggested by OSPAR (2005) to compare data given in dry or fresh weight, the PAHs, Mn, Pb, and Zn accumulated in mussels in this study are within the range found by other authors in Mytilus spp, and are similar to found by our team in mussels from the Ría of Vigo (Broman et al., 1990; Baumard et al., 1998; Piccardo et al., 2001; Besada et al., 2002; O'Connor, 2002; Webster et al., 2006; Monteduro et al., 2007; Bartolomé et al., 2010; Ruiz et al., 2011). Compared to the composition of the tar used, the variation of hydrocarbons and metals in the mussels exposed to WAF of different concentrations of tar seems to indicate that PAHs with a larger number of benzene rings, Zn and Pb are more efficiently accumulated. On the other hand, the decrease of these species of PAHs and metals in the mussels after depuration for 2 weeks suggest their faster elimination, like it is also appointed by other authors (Phillips, 1976; George, 1980; Rainbow, 2002; Glad et al., 2017). These facts seem to reflect the specific metabolism of Mytilus and the complex relationship between bioavailability, accumulation, biotransformation, and excretion of these compounds.
Gonadal and Histological Disorders
In the present study, cumulative gonadal disorders were observed in male mussels concerning both tar exposure and accumulation of PAHs and metals. In molluscs, similar disorders have been related to PAHs and metals pollution in wild environments (Pridmore et al., 1990; Viarengo et al., 1990; Bolognesi et al., 1999; Alonso et al., 2001; Tay et al., 2003; Aarab et al., 2004; Smolders et al., 2004; Jing-Jing et al., 2009; Yurchenko and Vaschenko, 2010; Ortiz-Zarragoitia et al., 2011; Ruiz et al., 2011, 2013; Smolarz et al., 2017). However, this is the first study demonstrating that tar used in the waterproofing of the floating rafts from mussel farms has a direct causal relationship with the histopathological alterations observed in male mussel gonad, which affect the reproductive function, the associate storage tissue metabolism and the immune response.
The germinal epithelium of male gonad and the spermatogenesis process are, in general terms, astonishingly similar in even very different animals, and their control mechanism is also highly conserved (Roosen-Runge, 1977; Working, 1988; Bonilla and Xu, 2008; Cheng and Mruk, 2010; White-Cooper and Bausek, 2010). However, there are scarce studies about cellular disorders and pathologies of this process in invertebrates, contrarily to in vertebrates. In the latter, the testis is a known target of PAHs and metals toxicity, experiencing disruption of spermatogenesis, degeneration, and loss of germ cell, and testicular atrophy (Ronis et al., 1996; Adhikari et al., 2001; Creasy and Foster, 2002; Dietrich and Krieger, 2009; El-Sayed and El-Neweshy, 2010; Mathur et al., 2010; Ebrahimi and Taherianfard, 2011; Creasy et al., 2012; Chandel and Jain, 2014).
In this study, the first histological effect of exposure to tar in mussels was the spawning induction, like described in other invertebrates (Tay et al., 2003; Aarab et al., 2004; Jing-Jing et al., 2009; Ortiz-Zarragoitia et al., 2011). However, as tar concentration and exposure time increases, the effect observed was a gonadal atrophy, manifested as a significant decline of the GCI and a drastic reduction of gonadal follicles size. In vertebrates, diameter reduction of seminiferous tubules is associated with loss of germ cells and constitutes a quantitative index of testicular injury (Creasy and Foster, 2002; Richburg et al., 2002; Dietrich and Krieger, 2009).
Cell Injuries
As dose and exposure time increased, pathological disorders in different cell types were observed. The study of these cellular alterations could subsequently shed light on cellular target of tar in the gonad of molluscs, as well as on the role of these cells in the spermatogenesis regulation of these organisms.
In addition to spawning induction, exposure to 40 mg L−1 tar for 10 days provoked a premature release or sloughing of immature spermatids, which resulted in cellular disorganisation of germinal epithelium and loss of its centripetal radial architecture, as has also been described in others molluscs from polluted environments (Yurchenko and Vaschenko, 2010). However, higher concentrations of tar and longer exposure times originated retention of abnormal aggregates of spermatids or spermatocytes with signs of degeneration and apoptosis, which occasionally were seen invading the connective storage tissue, like some spermatic granulomas described in vertebrates (Creasy et al., 2012; Greaves, 2012). These aggregates, have been termed multinucleated giant cells in several organisms where were related with disturbances of Sertoli cells and with the arrest of spermatogenesis and spermiogenesis (Creasy and Foster, 2002; De Falco et al., 2015). Among functions of Sertoli cells are the paracrine signalling, regulation of the material passing to gametes developing, structural support of spermatogenesis and release of the mature spermatids (Creasy and Foster, 2002; França et al., 2015). These functions are accomplished through junctional complexes maintained with the developing germ cells. Several toxicants, including hydrocarbons, disturb these junctions, leading to spermatogenesis failure (Richburg et al., 2002; Salian et al., 2009; Cheng, 2014).
As described above, in mussels exposed to 40 mg L−1 tar for 17 days and to 60 mg L−1 tar for 10 days, auxiliary or Sertoli-like cells proliferated abnormally, their aspect changed acquiring a swelled and vacuolated cytoplasm, and basal position of their nucleus was modified. Similar injuries have been described in Sertoli cells of vertebrates caused by toxicants and related to rapid and massive degeneration of germ cells, which suggest disruption of their structural and metabolic function (Creasy and Foster, 2002; Dietrich and Krieger, 2009; Cheng, 2014). Another function of Sertoli cells is phagocytosis and removal of residual sperm and degenerating germ cells (Boekelheide et al., 2000; França et al., 2015). This function seemed highly induced in these mussels, in which an inordinate phagocytic activity of apoptotic germ cells was observed, as also has been described in fish (Dietrich and Krieger, 2009). So, the Sertoli cells hyperplasia could be both a response to counteract germinal cells degeneration through their phagocytic capacity, as also the result of a direct endocrine disruptor effect of tar. Moreover, similar as we observed in a large number of follicles from these mussels, seminiferous tubules full of pleomorphic and vacuolated Sertoli cells have been described in neoplastic and degenerative processes of these cells from vertebrates (Henley et al., 2002; Vegter et al., 2010). According to this latter idea, the absence of Sertoli cells in mussels exposed to 60 mg L−1 tar for 17 days seems to support their possible degeneration. The diminution or absence of Sertoli cells cause deregulation of germ cells and their most severe disorders, getting to form encapsulated nodules invading the connective tissue, similar to in situ germinal carcinomas described in vertebrates (Guminska et al., 2010).
The storage cells from interstitial connective tissue (VC and ADG cells) of the mussels exposed to 40 mg L−1 of tar mixture for 17 days and to 60 mg L−1 during 10 or more days showed pathological hyperplasia, hypertrophy and appearance changes compared to those of the reference mussels. These morphologic changes seem to suggest an alteration of their biochemical components and their function. In Mytilus spp, the VC cells function is the glycogen storage. These cells contain only a large glycogen vacuole, some mitochondria and a limited metabolic activity. However, in this work, the hyperplasia and hypertrophy of these cells as a consequence of tar exposition was parallel to depletion of glycogen store. Glycogen is the main energetic reserve in adult bivalves, and several authors have also reported its decrease as a result of oil exposure, limiting the energy available for growth and reproduction (Pridmore et al., 1990; Smolders et al., 2004). On the other hand, the ADG cells contain mainly lipid droplets and protein granules and present a high metabolic activity that varies during the gametogenic cycle under hormonal control (Pipe, 1987; Peek et al., 1989). Several authors identify the ADG cells with Leydig cells, but although there are hints on their possible role in steroidogenesis, their function (trophic and/or regulatory) has not yet been fully clarified (Croll and Wang, 2007; Lafont and Mathieu, 2007). In Leydig cells from vertebrates, similar disorders to those we described in the ADG cells from mussels in this work have been identified as pathologic: abnormal enlargement, changes in shape, and internal structure and foamy appearance of cytoplasm due to proliferation of lipid droplets (lipidosis) (Creasy and Foster, 2002; Dietrich and Krieger, 2009; Cheng, 2014). Lipidosis of Leydig cells has been related to their degeneration and with disturbance of their function (Chung et al., 2011; Oh, 2014). The Leydig cells function is the biosynthesis of testosterone, which indirectly regulates spermatogenesis in a complex cascade of chemical interactions with Sertoli and germ cells, resulting in feedback regulation of testosterone synthesis. So, disorders of Sertoli cells, such as those we described above, not only disrupt the interactions with germ cells causing the spermatogenesis failure, but also the interactions with Leydig cells, inhibiting testosterone production, which in turn induces germ cell degeneration (Creasy and Foster, 2002; Boekelheide, 2005). The sequence of disorders that we described in this work seems to point to these complex interrelations. So, the auxiliary cells of mussels, like the Sertoli cells of vertebrate, could be one of the primary targets of PAHs toxicity, whose injuries could induce sloughing of immature germ cells, as well as disturb the possible steroidogenic function of the ADG cells. Moreover, the ADG and germinal cells could also constitute secondary targets of these compounds, inducing the degeneration and the severe injuries we described in germ cells.
Fibrosis is defined as the abnormal proliferation of fibroblast resulting in an increased synthesis and deposition of collagen, which confers a thickened appearance of the connective tissue. This phenomenon is associated to diverse pathologies as degenerative lesions and tumours coursing with inflammatory processes. In the connective tissue lining seminiferous tubules of the vertebrate testis, fibrosis has been equally described associated with injuries and degeneration of germ cells but also following exposure to endocrine disruptors (Creasy and Foster, 2002; Albrecht et al., 2006; Dietrich and Krieger, 2009). The collagen synthesis stimulation in the fibrotic reaction is mediated by the release of fibroblast growth factors from paracrine and/or tumoral cells, whose induction has been related to estrogens and oxidative stress (Walker, 2001; Albrecht et al., 2006; Dietrich and Krieger, 2009; Gómez-Sámano et al., 2017). This process is considered to be a scarring reaction aimed to maintain the structure of the tissue after degenerative injuries (Albrecht et al., 2006; Dietrich and Krieger, 2009). However, the estrogens-induced fibrosis in reproductive tract seems to suggest a different function related to the testicular development and differentiation (Watanabe and Onitake, 1995; Dietrich and Krieger, 2009; Jiang et al., 2013). Also in the gonad of bivalve molluscs, several authors have reported fibrosis associated to gonadal and haemocytic neoplasms, as well as to hydrocarbons and metals pollution and oxidative stress (Yevich and Barszcz, 1976; Peters et al., 1994; Alonso et al., 2001). In our work, the fibrotic reaction observed around gonadal follicles, related to spermatogenesis arrest and the severe disorders that we described, could be caused jointly by possible xenoestrogenic effects of tar and by the oxidative stress and cell damage generated, as described in other organisms.
The immune response is responsible for recognition, oxidation, and phagocytosis of non-self particles. The bivalve immune system is a non-specific defence mechanism known as the innate immune system, which is yet not fully understood. This is a primitive mechanism, evolutionarily conserved, triggered by intracellular molecular signalling cascades (Danilova, 2006; Song et al., 2010). The haemocytes activity from bivalves is not limited to immune response, but they are involved in different physiological processes including tissue repair, shell production, nutrition, and response to environmental and biological stresses (Song et al., 2010). The lineage of bivalve haemocytes is not clear. Several authors have suggested their origin from two different cells, precursors of granulocytes, and hyalinocytes, respectively. However, other authors indicate a single type of precursor cell of hyalinocytes, which would mature to granulocytes (Cheng, 1984; Hine, 1999). Moreover, the different types of granulocytes identified in bivalves (basophils and eosinophils) could be the result of subsequent differentiation and maturation processes (Donaghy et al., 2009).
The bivalve haemocytes seem particularly susceptible to environmental pollution by chemical or biological toxics. However, the bibliographic data are sometimes contradictory because several authors describe an increase of haemocytes number in bivalves exposed to oil spills, whereas others report their decrease (Pichaud et al., 2008; Hannam et al., 2009). Moreover, it has been described that innate immune response in bivalves is accomplished mainly by granulocytes, while hyalinocytes are the main responsible of tissues repair, requiring aggregation at a point of injury (Ruddell, 1971; Hégaret et al., 2003). According to these assertions and with studies above cited, our results show firstly an evident decrease of the number of eosinophilic granulocytes in mussels undergoing at all tar concentrations and exposition times assayed, evidencing immunosuppression induced by treatment, although no infection was observed. Secondarily, in mussels exposed to the highest tar concentration, which developed the more severe injuries, we observed an increase of basophilic granulocytes forming aggregates around the affected follicles. These data suggest that the immunotoxic effects of PAHs are dependent on concentration and exposure time, resulting in either an increase or decrease of different types of granulocytes, whose specific functions should be addressed in later works. On the other hand, the significant decrease of eosinophilic granulocytes induced by tar intoxication in mussels would explain the absence of these phagocytic granulocytes containing degradation products described by others authors as brown cells (Puy-Azurmendi et al., 2010; Bignell et al., 2011).
Depuration Assay
Several works conclude that hormonal disturbance effects may be reverted as the xenoendocrine disrupters disappear, but it is known that processes of cell death are irreversible (Creasy and Foster, 2002; Creasy et al., 2012). In our study, the mussels transplanted to non-toxic conditions after the toxicity assay showed recovery signs of all cellular injuries.
Hydrocarbons, metals, and other chemicals exert direct effects on the function of Leydig and Sertoli cells in vertebrates, causing, among other, inhibition of testosterone synthesis, cholesterol regulation, lipid homeostasis, and insulin signalling (Jeng, 2014; De Falco et al., 2015). Testosterone inhibition, in turn, induces germ cell degeneration (Creasy and Foster, 2002; Boekelheide, 2005). In our work, the connective tissue cells (VC and ADG cells) from mussels transplanted to depuration conditions, recovered their appearance. So, the alterations described in these cells could be due to a direct xenoestrogenic effect or mediated by endocrine disruption of auxiliary cells. In these mussels, the germinal line was also partially restored, and no signs of apoptosis and degeneration were observed. In vertebrates it has been demonstrated that spermatogonial precursor cells are relatively resistant to injurious stimuli (Creasy and Foster, 2002; Creasy et al., 2012). So, in our study, some spermatogonial stem cells would have remained intact to allow resuming the gametogenesis. Nevertheless, due to the short time of transplant to non-toxic conditions, several disorders, as germinal epithelium discontinuity and aggregations of multinucleated and hyperchromatic spermatocytes were still evident.
As described in other bivalve species (Mayrand et al., 2005), we also observed granulocytes number is recovered, but we have not enough data to know if their phagocytic capacity is recovered too, neither if animals become more sensitive to stress and pathogens. Non-cellular defence responses could also be involved in this granulocytes number recovery as described in some bivalves (Charlet et al., 1996; Roch, 1999; Ortiz-Zarragoitia and Cajaraville, 2006). However, these studies are still scarce to understand the integrated immunomodulation in these organisms.
Conclusions
The chemicals accumulation, and biometric and morphometric analysis of the mussel male gonad presented in this study indicates quantitative specific patterns of change after exposure to tar as corroborated by multivariate analysis (concentration of PAHs accumulated; number of gonadal follicles, haemocytes, and connective interstitial cells; area of VC cells), which together with the qualitative observations of other histopathological changes (fibrosis, appearance change of the Sertoli, ADG, and germinal cells) could offer a mechanistic understanding of the joint effects of hydrocarbons and metals on reproductive function of invertebrates. This histopathological approach could support further molecular studies in order to clarify the role of the different gonadal cells in the spermatogenesis regulation of invertebrates, and be used in aquatic toxicology.
To our knowledge, this is the first study to demonstrate that tars used in the waterproofing of the floating rafts from mussel farms cause severe effects on male mussel gonad, inducing histopathological alterations, which affect the reproductive function, the associate storage tissue and the immune response. The malignancy of injuries is dependent on the concentration and exposure time and affects to different cell types in a manner comparable to that described in other organisms both vertebrates and invertebrates. So, the spillage of these mixtures around rafts entails a direct pollution risk on the immediate environment of farms, which affect the health of mussels, their reproduction and ultimately, the sustainability of this resource and its quality for human consumption.
Our findings suggest that the histopathological changes produced by the combined effects of heavy metals and PAHs tar that we described could be used as biomarkers in the monitoring of marine pollution and quality of aquaculture areas. Also, the mussel gonad could be considered as an excellent model in studies about the testicular toxicity mechanisms, disruption of spermatogenesis and metabolism in male reproduction.
Data Availability
All datasets generated for this study are included in the manuscript/Supplementary Files.
Author Contributions
AA, YR, and FS designed the study. AA, PS, YR, and VD performed the data collection and wrote the draft of the manuscript. AA performed the histological and microphotographic analysis. PS performed the statistical analysis. AA, PS, YR, VD, and FS contributed to the interpretation of the results. PS and FS contributed to the final manuscript.
Funding
This research did not receive any specific grant from funding agencies in the public, commercial, or not-for-profit sectors.
Conflict of Interest Statement
The authors declare that the research was conducted in the absence of any commercial or financial relationships that could be construed as a potential conflict of interest.
Acknowledgments
We thank Antonio Antepazos by providing the mussels and tars used in this work and Drs. Juan Carro and Pilar Solla (Department of Anatomic Pathology, Montecelo Hospital, Pontevedra, Spain) for their comments.
Supplementary Material
The Supplementary Material for this article can be found online at: https://www.frontiersin.org/articles/10.3389/fmars.2019.00577/full#supplementary-material
References
Aarab, N., Minier, C., Lemaire, S., Unruh, E., Hansen, P. D., and Larsen, B. K. (2004). Biochemical and histological responses in mussel (M. edulis) exposed to North Sea oil and to a mixture of North Sea oil and alkylphenols. Mar. Environ. Res. 58, 437–441. doi: 10.1016/j.marenvres.2004.03.121
Abbas, I., Garçon, G., Saint, G. F., Andre, V., Gosset, P., Billet, S., et al. (2013). Polycyclic aromatic hydrocarbons within airborne particulate matter (PM2.5) produced DNA bulky stable adducts in a human lung cell coculture model. J. Appl. Toxicol. 33, 109–119. doi: 10.1002/jat.1722
Adhikari, N., Sinha, N., Narayan, R., and Saxena, D. K. (2001). Lead induced death cell in testis of young rats. J. Appl. Toxicol. 27, 275–277. doi: 10.1002/jat.754
Albrecht, M., Rämsch, R., Köhn, F. M., Schwarzer, J. U., and Mayerhofer, A. (2006). Isolation and cultivation of human peritubular cells: a novel model for investigation of fibrotic processes in the human testis and male infertility. J. Clin. Endocrinol. Metab. 81, 1956–1960. doi: 10.1210/jc.2005-2169
Alonso, A., Suárez, P., Álvarez, C., San Juan, F., and Molist, P. (2001). Structural study of a possible neoplasia detected in Mytilus galloprovincialis collected from the Ría of Vigo (NW Spain). Dis. Aquat. Organ. 47, 73–79. doi: 10.3354/dao047073
Anderson, M. J., Gorley, R. N., and Clarke, K. R. (2008). PERMANOVA+ for PRIMER: Guide to Software and Statistical Methods. Plymouth, UK: PRIMER-E.
Arcos, J. C., and Argus, M. G. (1975). Chemical Induction of Cancer: Structural Basis and Biological Mechanisms. New York, NY: IIA. Academic Press.
Au, D. W. T. (2004). The application of histo-cytopathological biomarkers in marine pollution monitoring: a review. Mar. Pollut. Bull. 48, 817–834. doi: 10.1016/j.marpolbul.2004.02.032
Aurand, D., and Coelho, G. (2005). Cooperative Aquatic Toxicity Testing of Dispersed Oil and the Chemical Response to Oil Spills: Ecological Effects Research Forum (CROSERF). Technical Report 07-03. Lusby: MD Ecosystem Management and Associates, Inc., Available online at: https://www.bsee.gov/sites/bsee.gov/files/osrr-oil-spill-response-research//296ac.pdf
Baird, W. M., Hooven, L. A., and Mahadevan, B. (2005). Carcinogenic polycyclic aromatic hydrocarbon-DNA adducts and mechanism of action. Environ. Mol. Mutagen. 45, 106–114. doi: 10.1002/em.20095
Balabanič, D., Rupnik, M., and Klemenčič, A. K. (2011). Negative impact of endocrine-disrupting compounds on human reproductive health. Reprod. Fertil. Dev. 23, 403–416. doi: 10.1071/RD09300
Bartolomé, L., Navarro, P., Raposo, J. C., Arana, G., Zuloaga, O., Etxebarría, N., et al. (2010). Occurrence and distribution of metals in mussels from Cantabrian Coast. Arch. Environ. Contam. Toxicol. 59, 235–243. doi: 10.1007/s00244-010-9476-7
Baumard, P., Budzinski, H., and Garrigues, P. (1998). Polycyclic aromatic hidrocarbons in sediments and mussels of the western Mediterranean Sea. Environ. Toxicol. Chem. 17, 765–776. doi: 10.1002/etc.5620170501
Besada, V., Fumega, J., and Vaamonde, A. (2002). Temporal trends of Cd, Cu, Hg, Pb and Zn in mussel (Mytilus galloprovincialis) from the Spanish North-Atlantic coast 1991-1999. Sci. Total Environ. 288, 239–253. doi: 10.1016/S0048-9697(01)01010-5
Beyersmann, D., and Hartwig, A. (2008). Carcinogenic metal compounds: recent insight into molecular and cellular mechanisms. Arch. Toxicol. 82, 493–512. doi: 10.1007/s00204-008-0313-y
Bignell, J. P., Stentiford, G. D., Taylor, N. G. H., and Lyons, B. P. (2011). Histopathology of mussels (Mytilus sp.) from the Tamar estuary, UK. Mar. Environ. Res. 72, 25–32. doi: 10.1016/j.marenvres.2011.05.004
Boekelheide, K. (2005). Mechanisms of toxic damage to spermatogenesis. J. Natl. Cancer Inst. Monographs. 34, 1–3. doi: 10.1093/jncimonographs/lgi006
Boekelheide, K., Fleming, S. L., Johnson, K. J., Patel, S. R., and Schoenfeld, H. A. (2000). Role of Sertoli cells in injury-associated testicular germ cell apoptosis. Proc. Soc. Exp. Biol. Med. 225, 105–115. doi: 10.1046/j.1525-1373.2000.22513.x
Bolognesi, C., Landini, E., Roggieri, P., Fabbri, R., and Viarengo, A. (1999). Genotoxicity biomarkers in the assessment of heavy metal effects in mussels: experimental studies. Environ. Mol. Mutagen. 33, 287–292. doi: 10.1002/(SICI)1098-2280(1999)33:4<287::AID-EM5>3.0.CO;2-G
Bonilla, E., and Xu, E. Y. (2008). Identification and characterization of novel mammalian spermatogenic genes conserved from fly to human. Mol. Human. Reprod. 14, 137–142. doi: 10.1093/molehr/gan002
Boström, C. E., Gerde, P., Hanberg, A., Jernström, B., Johansson, C., Kyrklund, T., et al. (2002). Cancer risk assessment, indicators, and guidelines for polycyclic aromatic hydrocarbons in the ambient air. Environ. Health. Persp. 110, 451–488. doi: 10.1289/ehp.110-1241197
Broman, D., Näuf, C., Lundbergh, I., and Zebühr, Y. (1990). An in situ study on the distribution, biotransformation and flux of polycyclic aromatic hydrocarbons (PAHs) in an aquatic food chain (seston-Mytilus edulis L.-Somateria mollissima L.) from the Baltic: an ecotoxicological perspective. Environ. Toxicol. Chem. 9, 429–442. doi: 10.1002/etc.5620090404
Caballero Miguez, G., Garza Gil, M. D., and Varela Lafuente, M. M. (2009). The institutional foundations of economic performance of mussel production: the Spanish case of the Galician floating raft culture. Mar. Policy 33, 288–296. doi: 10.1016/j.marpol.2008.07.008
Chandel, M., and Jain, G. C. (2014). Toxic effects of transition metals on male reproductive system: a review. J. Environ. Occup. Sci. 3, 204–2013. doi: 10.5455/jeos.20140929042630
Chang, L. W., Magos, L., and Suzuki, T. (1996). Toxicology of Metals, Vol. 1. 1st Edn. Boca Raton. FL: CRC Press.
Charlet, M., Chernysh, S., Phillippe, H., Hetru, C., Hoffmann, J. A., and Bulet, P. (1996). Innate immunity: isolation of several cystein-rich antimicrobial peptides from the blood of a mollusc, Mytilus edulis. J. Biol. Chem. 271, 21808–21813. doi: 10.1074/jbc.271.36.21808
Cheng, C. Y. (2014). Toxicants target cell junctions in the testis: insights from the indazole-carboxylic acid model. Spermatogenesis. 4:e981485. doi: 10.4161/21565562.2014.981485
Cheng, C. Y., and Mruk, D. D. (2010). The biology and regulation of spermatogenesis: the past, present and future. Phil. Trans. R. Soc. 365, 1459–1463. doi: 10.1098/rstb.2010.0024
Cheng, T. C. (1984). “A classification of molluscan haemocytes based on functional evidence,” in Comparative Pathobiology, Vol. 6, Invertebrate Blood, ed T. C. Cheng (New York, NY: Springer), 111–146. doi: 10.1007/978-1-4684-4766-8_5
Chung, J. Y., Kim, Y. J., Kim, J. Y., Lee, S. G., Park, J. E., Kim, W. R., et al. (2011). Benzo(a)pyrene reduces testosterone production in rat Leydig cells via a direct disturbance of testicular steroidogenic machinery. Environ. Health. Persp. 119, 1569–1574. doi: 10.1289/ehp.1003391
Creasy, D., Bube, A., De Rijk, E., Kandori, H., Kuwahara, M., Masson, R., et al. (2012). Proliferative and nonproliferative lesions of the rat and mouse male reproductive system. Toxicol. Pathol. 40, 40S–121S. doi: 10.1177/0192623312454337
Creasy, D. M., and Foster, P. M. D. (2002). “Male reproductive system,” in Handbook of Toxicology Pathology, Vol. 2, eds W. M. Haschek, C. A. Rousseaux, M. A. Wallig (New York, NY: Academic Press), 785–846. doi: 10.1016/B978-012330215-1/50043-0
Croll, R. P., and Wang, C. (2007). Possible roles of sex steroids in the control of reproduction in bivalve molluscs. Aquaculture 272, 76–86. doi: 10.1016/j.aquaculture.2007.06.031
Danilova, N. (2006). The evolution of immune mechanisms. J. Exp. Zool. Mol. Dev. Evol. 306, 496–520. doi: 10.1002/jez.b.21102
De Falco, M., Forte, M., and Laforgia, V. (2015). Estrogenic and anti-androgenic endocrine disrupting chemicals and their impact on male reproductive system. Front. Environ. Sci. 3:3. doi: 10.3389/fenvs.2015.00003
DeFur, P. L. (2004). Use and role of invertebrate models in endocrine disruptor research and testing. ILAR J. 45, 484–493. doi: 10.1093/ilar.45.4.484
Diepens, N. J., Koelmens, A. A., Baveco, H., Van der Brink, P. J., Van der Heuvel-Greve, M. J., and Brock, C. M. (2017). “Prospective environmental risk assessment for sediment-bound organic chemicals: a proposal for tiered effect assessment,” in Reviews of Environmental Contamination and Toxicology Series, Vol. 239, ed P. de Vooght (Amsterdam: Springer), 1–77. doi: 10.1007/398_2015_5004
Dietrich, D. R., and Krieger, H. O. (2009). Histological Analysis of Endocrine Disruptive Effects in Small Laboratory Fish. New Jersey, NJ: John Wiley & Sons, Inc.
Donaghy, L., Lambert, C., Choi, K. S., and Soudant, P. (2009). Hemocytes of the carpet shell clam (Ruditapes decussatus) and the Manila clam (Ruditapes philippinarum): current knowledge and future prospects. Aquaculture 297, 10–24. doi: 10.1016/j.aquaculture.2009.09.003
Ebrahimi, M, and Taherianfard, M. (2011). The effects of heavy metals exposure on reproductive systems of cyprinid fish from Kor River. Iran J Fish Sci. 10, 13–24. Available online at: http://jifro.ir/files/site1/user_files_eb12be/admin-A-10-1-56-e9f44ce.pdf
El-Sayed, Y. S., and El-Neweshy, M. S. (2010). Impact of lead toxicity on male rat reproduction at “hormonal and histopathological levels”. Toxicol. Environ. Chem. 92,765–774. doi: 10.1080/02772240902984453
Ercal, N., Gurer-Orhan, H., and Aykin-Burns, N. (2001). Toxic metals and oxidative stress part I: mechanisms involved in metal-induced oxidative damage. Curr. Top. Med. Chem. 1, 529–539. doi: 10.2174/1568026013394831
Farrington, J. W., Tripp, B. W., Tanabe, S., Subramanian, A., Sericano, J. L., Wade, T. L., et al. (2016). Goldberg's proposal of “the Mussel Watch”: reflections after 40 years. Mar. Pollut. Bull. 110, 501–551. doi: 10.1016/j.marpolbul.2016.05.074
Fatima, M., Usmani, N., and Hossain, M. M. (2014). Heavy metal in aquatic ecosystem emphasizing its effect on tissue bioaccumulation and histopathology: a review. J. Environ. Sci. Technol. 7, 1–15. doi: 10.3923/jest.2014.1.15
Flora, S. J. S., Mittal, M., and Mehtam, A. (2008). Heavy metal induced oxidative stress & its possible reversal by chelation therapy. Indian. J. Med. Res. 128, 501–523. Available online at:http://medind.nic.in/iby/t08/i10/ibyt08i10p501.pdf
França, L. R., Nóbrega, R. H., Morais, R. D. V. S., De Castro Assis, L. H., and Schulz, W. (2015). “Sertoli cell structure and function in anamniote vertebrates,” in Sertoli Cell Biology, 2nd Edn., ed M. D. Griswold (Pullman, WA: Elsevier), 385–409. doi: 10.1016/B978-0-12-417047-6.00013-2
George, S. G. (1980). Correlation of Metal Accumulation in Mussels With the Mechanisms of Uptake, Metabolism and Detoxification: Biochemical and Ultra-Structural Studies (Extended Abstract). Vlth Int. Conf. Chem. Medit. Rovinj, Yugoslavia.
Gerber, G. B., Léonard, A., and Jacquet, P. (1980). Mutagenicity, carcinogenicity and teratogenicity of Lead. Mut. Res. Gen. Toxicol. 76, 115–141. doi: 10.1016/0165-1110(80)90006-8
Glad, M., Bihari, N., Jakšić, Ž., and Fafandel, M. (2017). Comparison between resident and caged mussels: polycyclic aromatic hydrocarbon accumulation and biological response. Mar. Environ. Res. 129, 195–206. doi: 10.1016/j.marenvres.2017.06.004
Gómez-Sámano, M. A., Grajales-Gómez, M., Zuarth-Vázquez, J. M., Navarro-Flores, M. F., Martínez-Saavedra, M., Juárez-León, O. A., et al. (2017). Fibroblast growth factor 21 and its novel association with oxidative stress. Redox. Biol. 11, 335–341. doi: 10.1016/j.redox.2016.12.024
Goyer, R. A., and Clarkson, T. W. (2001). “Toxic effect of metals,” in Toxicology: The Basic Science of Poisons, ed C. D. Klaassen (New York, NY: McGraw-Hill), 811–868.
Greaves, P. (2012). “Chapter 11: Histopathology of preclinical toxicity studies. interpretation and relevance in drug safety studies,” in Chapter 11, Male Genital Tract, 4th Edn. (Amsterdam: Elsevier), 615–666. doi: 10.1016/B978-0-444-53856-7.00011-7
Guminska, A., Oszukowska, E., Kuzanski, W., Sosnowski, M., Wolski, J. K., Walczak-Jedrzejowska, R., et al. (2010). Less advanced testicular dysgenesis is associated by a higher prevalence of germ cell neoplasia. Int. J. Androl. 33, e153–162. doi: 10.1111/j.1365-2605.2009.00981.x
Haines, P. A., and Hendrickson, M. D. (2009). Polycyclic Aromatic Hydrocarbons: Pollution, Health Effects and Chemistry. (Polymer Science and Technology Series). New York, NY: NOVA Science Publishers.
Hannam, M. L., Bamber, S. D., Galloway, T. S., Moody, A., and Jones, M. B. (2009). Effects of the model PAH phenanthrene on immune function and oxidative stress in the haemolymph of the temperate scallop Pecten maximus. Chemosphere 78,779–784. doi: 10.1016/j.chemosphere.2009.12.049
Hégaret, H., Wikfors, G. H., and Soudant, P. (2003). Flow-cytometric analysis of haemocytes from eastern oyster, Crassostrea virginica, subjected to a sudden temperature elevation. II. Haemocyte functions aggregation, viability, phagocytosis and respiratory burst. J. Exp. Mar. Biol. Ecol. 293, 249–265. doi: 10.1016/S0022-0981(03)00235-1
Henley, J. D., Young, R. H., and Ulbright, T. M. (2002). Malignant sertoli cell tumors of the testis: a study of 13 examples of a neoplasm frequently misinterpreted as seminoma. Am. J. Surg. Pathol. 26, 541–550. doi: 10.1097/00000478-200205000-00001
Hine, P. M. (1999). The inter-relationships of bivalve haemocytes. Fish. Shellfish. Immun. 9, 367–385. doi: 10.1006/fsim.1998.0205
IARC (International Agency for Research on Cancer) (1989). “Diesel and Gasoline Engine Exhausts and Some Nitroarenes. Vol 46,” in IARC Monographs on the Evaluation of Carcinogenic Risk to Humans (Lyon IARC Press), 41-45. Available online at: http://monographs.iarc.fr/ENG/Monographs/vol46/mono46.pdf
Jeng, H. A. (2014). Exposure to endocrine disrupting chemicals and male reproductive health. Front. Public. Health. 2:55. doi: 10.3389/fpubh.2014.00055
Ji, K., Seo, J., Liu, X., Lee, J., Lee, S., Lee, W., et al. (2011). Genotoxicity and endocrine-disruption potentials of sediment near an Oil Spill Site: two years after the Hebei Spirit Oil Spill. Environ. Sci. Technol. 45, 7481–7488. doi: 10.1021/es200724x
Jiang, X., Skibba, M., Zhang, C., Tan, Y., Xin, Y., and Qu, Y. (2013). The roles of Fibroblast growth factors in the testicular development and tumor. J. Diabetes. Res. 2013:489095. doi: 10.1155/2013/489095
Jing-Jing, M., Lu-qing, P., Jing, L., and Lin, Z. (2009). Effects of benzo(a)pyrene on DNA damage and histological alterations in gonad of scallop Chlamys farreri. Mar. Environ. Res. 67, 47–52. doi: 10.1016/j.marenvres.2008.10.006
Lafont, R., and Mathieu, M. (2007). Steroids in aquatic invertebrates. Ecotoxicology 16, 109–130. doi: 10.1007/s10646-006-0113-1
Lavado, R., Janer, G., and Porte, C. (2006). Steroid levels and steroid metabolism in the mussel Mytilus edulis: the modulating effect of dispersed crude oil and alkyphenols. Aquat. Toxicol. 78 (Suppl.), S63–S72. doi: 10.1016/j.aquatox.2006.02.018
Lehr, R. E., and Jerina, D. M. (1977). Metabolic activation of polycyclic hydrocarbons. Arch. Toxicol. 39, 1–6. doi: 10.1007/BF00343269
Lubet, P. (1959). Recherches sur le cycle sexuel et l'émission des gamètes chez les mytilides et les pectinides (Mollusques bivalves). Rev. Trav. Inst. Pêches Marit. 23, 386–548.
Mathur, N., Pandey, G., and Jain, G. C. (2010). Male reproductive toxicity of some selected metals: a review. J. Biol. Sci. 10, 396–404. doi: 10.3923/jbs.2010.396.404
Matozzo, V., Ballarin, L., Pampanin, D. M., and Marin, M. G. (2001). Effects of copper and cadmium exposure on functional responses of hemocytes in the clam, Tapes philippinarum. Arch. Environ. Contam. Toxicol. 41, 163–170. doi: 10.1007/s002440010234
Mayrand, E., St-Jean, S. D., and Courtenay, S. C. (2005). Haemocyte responses of blue mussels (Mytilus edulis L.) transferred from a contaminated site to a reference site: can the immune system recuperate? Aquac. Res. 36, 962–971. doi: 10.1111/j.1365-2109.2005.01302.x
Monteduro, R. A., Pellizzato, F., Sperni, L., and Pavoni, B. (2007). Contamination in Mytilus galloprovincialis by chlorinated hydrocarbons (PCBs and pesticides), PAHs and heavy metals in the Lagoon of Venice. Polycyclic. Aromatic. Hydrocarbons. 27, 437–459. doi: 10.1080/10406630701624416
Mortelmans, K., and Zeiger, E. (2000). The Ames Salmonella/microsome mutagenicity assay. Mut. Res. 455, 29–60. doi: 10.1016/S0027-5107(00)00064-6
Nylund, L., Heikkila, P., Hameila, M., Pyr, L., Linnainmma, K., and Sorsa, M. (1992). Genotoxic effects and chemical composition of four creosotes. Mutat. Res. 265, 223–236. doi: 10.1016/0027-5107(92)90051-3
Obiakor, M. O., Okonkwo, J. C., Ezeonyejiaku, C. D., and Ezenwelu, C. O. (2010). Genotoxicology: single and joint action of copper and zinc to Synodontis clarias and Tilapia nilotica. J. Appl. Sci. Environ. Manage. 14, 59–64. doi: 10.4314/jasem.v14i3.61468
O'Connor, T. P. (2002). National distribution of chemical concentrations in mussels and oysters in the USA. Mar. Environ. Res. 53, 117–143. doi: 10.1016/S0141-1136(01)00116-7
Oetken, M., Bachmann, J., Schulte-Oehlmann, U., and Oehlmann, J. (2004). Evidence for endocrine disruption in invertebrates. Int. Rev. Cytol. 236, 1–44. doi: 10.1016/S0074-7696(04)36001-8
Oh, S. (2014). Disturbance in testosterone production in Leydig cells by polycyclic aromatic hydrocarbons. Dev. Reprod. 18, 187–195. doi: 10.12717/DR.2014.18.4.187
Ono, N., Oshio, S., Niwata, Y., Yoshid, S., Tsukue, N., Sugawara, I., et al. (2008). Detrimental effects of prenatal exposure to filtered diesel exhaust on mouse spermatogenesis. Arch. Toxicol. 82, 851–859. doi: 10.1007/s00204-008-0302-1
Ortiz-Zarragoitia, M., and Cajaraville, M. P. (2006). Biomarkers of exposure and reproduction-related effects in mussels exposed to endocrine disruptors. Arch. Environ. Contam. Toxicol. 50, 361–369. doi: 10.1007/s00244-005-1082-8
Ortiz-Zarragoitia, M., Garmendia, L., Barbero, M. C., Serrano, T., Mariogomez, I., and Cajaraville, M. P. (2011). Effects of the fuel oil spilled by the Prestige tanker on reproduction parameters of wild mussel populations. J. Environ. Monit. 13, 84–94. doi: 10.1039/C0EM00102C
OSPAR. Convention for the Protection of the Marine Environment of the North-East Atlantic (2005). Assessment of Data Collected Under the Co-Ordinated Environmental Monitoring Programme. Ospar Commision Publication Number: 2005/235.
Peek, K., Gabbott, P. A., and Runham, N. W. (1989). Adipogranular cells from the mantle tissue of Mytilus edulis L. II. Seasonal changes in the distribution of dispersed cells in a performed Percoll density gradient. J. Exp. Mar. Biol. Ecol. 126, 217–230. doi: 10.1016/0022-0981(89)90188-3
Peters, E. C., Yevich, J. C., Harshbarger, J. C., and Zaroogian, G. E. (1994). Comparative histopathology of gonadal neoplasms in marine bivalve molluscs. Dis. Aquat. Org. 20, 59–76. doi: 10.3354/dao020059
Phillips, D. J. H. (1976). The common mussel Mytilus edulis as an indicator of pollution by zinc, cadmium, lead and copper. I. Effects of environmental variables on uptake of metals. Mar. Biol. 38, 59–69. doi: 10.1007/BF00391486
Piccardo, M. T., Coradeghini, R., and Valerio, F. (2001). Polycyclic aromatic hydrocarbon pollution in native and caged mussels. Mar. Pollut. Bull. 42, 951–956. doi: 10.1016/S0025-326X(01)00057-1
Pichaud, N., Pellerin, J., Fournier, M., Gauthier-Clerc, S., Rioux, P., and Pelletier, E. (2008). Oxidative stress and immunologic responses following a dietary exposure to PAHs in Mya arenaria. Chem. Cent. J. 2:23. doi: 10.1186/1752-153X-2-23
Pipe, R. K. (1987). Ultrastructural and cytochemical study on interactions between nutrient storage cells and gametogenesis in the mussel Mytilus edulis. Mar. Biol. 96, 519–528. doi. 10.1007/BF00397969
Poirier, M. C. (2016). Linking DNA adduct formation and human cancer risk in chemical carcinogenesis. Environ. Mol. Mutagen. 57, 499–507. doi: 10.1002/em.22030
Porte, C., Janer, G., Lorusso, L. C., Ortiz-Zarragoitia, M., Cajaraville, M. P., Fossi, M. C., et al. (2006). Endocrine disruptors in marine organisms: approaches and perspectives. Comp. Biochem. Physiol. 143, 303–315. doi: 10.1016/j.cbpc.2006.03.004
Pridmore, R. D., Roper, D. S., and Hewitt, J. E. (1990). Variation in composition and condition of the Pacific oyster, Crassostrea gigas, along a pollution gradient in Manukau Harbour, New Zealand. Mar. Environ. Res. 30, 163–177. doi: 10.1016/0141-1136(90)90017-I
Pufulete, M., Battershill, J., Boobis, A., and Fielder, R. (2004). Approaches to carcinogenic risk assessment for polycyclic aromatic hydrocarbons: A UK perspective. Toxicol. App. Pharmacol. l40, 54–66. doi: 10.1016/j.yrtph.2004.04.007
Puy-Azurmendi, E., Ortiz-Zarragoitia, M., Kuster, M., Martínez, E., GUillamón, M., Domínguez, C., et al. (2010). An integrated study of endocrine disruptors in sediments and reproduction-related parameters in bivalve molluscs from the Biosphere's Reserve of Udaibai (Bay of Biscay). Mar. Environ. Res. 69, S63–S66. doi: 10.1016/j.marenvres.2009.10.003
Rainbow, P. S. (2002). Trace metal concentrations in aquatic invertebrates: why and so what? Environ. Pollut. 120, 497–507. doi: 10.1016/S0269-7491(02)00238-5
Richburg, J. H., Johnson, K. J., Schoenfeld, H. A., Meistrich, M. L., and Dix, D. J. (2002). Defining the cellular and molecular mechanisms of toxicant action in the testis. Toxicol. Lett. 135, 167–183. doi: 10.1016/S0378-4274(02)00254-0
Roch, P. (1999). Defense mechanisms and disease prevention in farmed marine invertebrates. Aquaculture 172, 125–145. doi: 10.1016/S0044-8486(98)00439-6
Ronis, M. J., Badger, T. M., Shema, S. J., Roberson, P. K., and Shaikh, F. (1996). Reproductive toxicity and growth effects in rats exposed to lead at different periods during development. Toxicol. App.l Pharmacol. 136, 361–71. doi: 10.1006/taap.1996.0044
Roosen-Runge, E. C. (1977). The Process of Spermatogenesis in Animals. Cambridge; London; New York, NY: Cambridge University Press.
Ruddell, C. L. (1971). The fine structure of oyster agranular amoebocytes from regenerating mantle wounds in the Pacific oyster, Crassostrea gigas. J. Invertebr. Pathol. 18, 260–268. doi: 10.1016/0022-2011(71)90154-6
Ruiz, Y., Suárez, P., Alonso, A., Longo, E., and San Juan, F. (2013). Mutagenicity test using Vibrio harveyi in the assessment of water quality from mussel farms. Water. Res. 47, 2742–2756. doi: 10.1016/j.watres.2013.02.031
Ruiz, Y., Suárez, P., Alonso, A., Longo, E., and San Juan, F. (2014). Chemicals used for maintenance of wood rafts in mussel farms: evaluation of their potential toxic risk for to mussel culture. Aquacult. Env. Interac. 6, 55–66. doi: 10.3354/aei00113
Ruiz, Y., Suarez, P., Alonso, A., Longo, E., Villaverde, C., and San Juan, F. (2011). Environmental quality of mussel farms in the Vigo estuary: pollution by PAHs, origin and effects on reproduction. Environ. Pollut. 159, 250–265. doi: 10.1016/j.envpol.2010.08.031
Salian, S., Doshi, T., and Vanage, G. (2009). Neonatal exposure of male rats to bisphenol A impairs fertility and expression of Sertoli cell junctional proteins in the testis. Toxicology 265, 56–67. doi: 10.1016/j.tox.2009.09.012
Sanders, J. M., Bucher, J. R., Peckham, J. C., Kissling, G. E., Hejtmancik, R. S., and Chabra, R. S. (2009). Carcinogenesis studies of cresols in rats and mice. Toxicology 257, 33–39. doi: 10.1016/j.tox.2008.12.005
Schäfer, S., and Köhler, A. (2009). Gonadal lesions of female sea urchin (Psammechinus miliaris) after exposure to the polycyclic aromatic hydrocarbon phenanthrene. Mar. Environ. Res. 68, 128–136. doi: 10.1016/j.marenvres.2009.05.001
Shen, G., Lu, Y., and Hong, J. (2006). Combined effect of heavy metals and polycyclic aromatic hydrocarbons on urease activity in soil. Ecotoxicol. Environ. Safety 63, 474–480. doi: 10.1016/j.ecoenv.2005.01.009
Singer, M. M., Aurand, D., Bragin, G. E., Clark, J. R., Coelho, G. M., Sowby, M. L., et al. (2000). Standardization of the preparation and quantification of water-accommodated fractions of petroleum for toxicity testing. Mar. Pollut. Bull. 40, 1007–1016. doi: 10.1016/S0025-326X(00)00045-X
Singer, M. M., Aurand, D., Coelho, G., Bragin, G. E., Clark, J. R., Jacobson, S., et al. (2001). “Making, measuring, and using water-accommodated fractions of petroleum for toxicity testing,” in Proceedings, 2001 International Oil Spill Conference, American Petroleum Institute (Washington, DC), 1269–1274. doi: 10.7901/2169-3358-2001-2-1269
Smolarz, K., Hallmann, A., Zabrzanska, S., and Pietrasik, A. (2017). Elevated gonadal atresia as biomarker of endocrine disruptors: field and experimental studies using Mytilus trossulus (L.) and 17-alpha ethinylestradiol (EE2). Mar. Pollut. Bull. 120, 58–67. doi: 10.1016/j.marpolbul.2017.04.007
Smolders, R., Bervoets, L., De Coen, W., and Blust, R. (2004). Cellular energy allocation in zebra mussels exposed along a pollution gradient: linking cellular effects to higher levels of biological organization. Environ. Pollut. 129, 99–112. doi: 10.1016/j.envpol.2003.09.027
Sokol, P. Z. (1987). Hormonal effects of lead acetate in the male rat: mechanism of action. J. Biol. Reprod. 37,1135–1138. doi: 10.1095/biolreprod37.5.1135
Song, L., Wang, L., Qiu, L., and Zhang, H. (2010). “Bivalve Immunity,” in Invertebrate Immunity Vol. 708 in Book Serie: Advances in Experimental Medicine and Biology, ed K. Söderhäll (New York, NY: Springer), 44–65. doi: 10.1007/978-1-4419-8059-5_3
Suárez Alonso, P., Álvarez González, C., Molist García, P., and San Juan Serrano, F. (2007). Atresia gonadal durante el ciclo gametogénico de Mytilus galloprovincialis Lamarck, 1819 cultivado en la ría de Vigo (noroeste de la península Ibérica). Bol. Inst. Esp. Oceanogr. 23, 1–4.
Suárez, M. P., Álvarez, C., Molist, P., and San Juan, F. (2005). Particular aspects of gonadal cycle and seasonal distribution of gametogenic stages of Mytilus galloprovincialis cultured in the Estuary of Vigo. J. Shellfish. Res. 24, 531–540. doi: 10.2983/0730-8000(2005)24[531:PAOGCA]2.0.CO;2
Swedenborg, E., Rüegg, J., Mäkelä, S., and Pongratz, I. (2009). Endocrine disruptive chemicals: mechanisms of action and involvement in metabolic disorders. J. Mol. Endocrinol. 43, 1–10. doi: 10.1677/JME-08-0132
Tay, K. L., The, S. J., Doe, K., Lee, K., and Jackman, P. (2003). Histopathology and histochemical biomarkers response of Baltic clam Macoma balthica, to contamined Sydney harbors sediment, Nova Scotia, Canada. Environ. Health. Persp. 111, 273–280. doi: 10.1289/ehp.5437
Tchounwou, P. B., Yedjou, C. G., Foxx, D., Ishaque, A., and Shen, E. (2004). Lead-induced cytotoxicity and transcriptional activation of stress genes in human liver carcinoma cells (HepG2). Mol. Cell. Biochem. 255, 161–170. doi: 10.1023/B:MCBI.0000007272.46923.12
Tchounwou, P. B., Yedjou, C. G., Patlolla, A. K., and Sutton, D. J. (2012). Heavy metals toxicity and the environment. EXS 101, 133–164. doi: 10.1007/978-3-7643-8340-4_6
Thompson, E. D., McDermott, J. A., Zerkle, T. B., Skare, J. A., Evans, B. L. B., and Cody, D. B. (1989). Genotoxicity of Zn in 4 short-term mutagenicity assays. Mut. Res. Gen. Toxicol. 223, 267–272. doi: 10.1016/0165-1218(89)90119-5
Vakharia, D. D., Liu, N., Pause, R., Fasco, M., Bessette, E., Zhang, Q. Y., et al. (2001). Effect of metals on polycyclic aromatic hydrocarbon induction of CYP1A1 and CYP1A2 in human hepatocyte cultures. Toxicol. Appl. Pharmacol. 170, 93–103. doi: 10.1006/taap.2000.9087
Veeramachaneni, D. N. (2008). Impact of environmental pollutants on the male: effects on germ cell differentiation. Anim. Reprod. Sci. 105, 144–157. doi: 10.1016/j.anireprosci.2007.11.020
Vegter, A. R., Kooistra, H. S., Van Sluijs, F. J., Van Bruggen, L. W. L., Ijzer, J., Zijlstra, C., et al. (2010). Persistent mullerian duct syndrome in a miniature schnauzer dog with signs of feminization and a Sertoli cell tumour. Reprod. Domest. Anim. 45, 447–452. doi: 10.1111/j.1439-0531.2008.01223.x
Viarengo, A., Pertica, M., Poli, G., Moore, M. N., and Orunesu, M. (1990). Heavy metal effects on lipid peroxidation in the tissues of Mytilus galloprovincialis Lam. Comp. Biochem. Physiol. 97C, 37–42. doi: 10.1016/0742-8413(90)90168-9
Villalba, A. (1995). Gametogenic cycle of cultured mussel, Mytilus galloprovincialis, in the bays of Galicia (N.W. Spain). Aquaculture 130, 269–277. doi: 10.1016/0044-8486(94)00213-8
Walker, R. A. (2001). The complexities of breast cancer desmoplasia. Breast. Cancer. Res. 3, 143–145. doi: 10.1186/bcr287
Wang, S., and Shi, X. (2001). Molecular mechanisms of metal toxicity and carcinogenesis. Mol. Cell. Biochem. 222, 3–9. doi: 10.1007/978-1-4615-0793-2_1
Wang, T., Feng, W., Kuang, D., Deng, O., Zhang, W., Wang, S., et al. (2015). The effects of heavy metals and their interactions with polycyclic aromatic hydrocarbons on the oxidative stress among coke-oven workers. Environ. Res. 140, 405–413. doi: 10.1016/j.envres.2015.04.013
Watanabe, A., and Onitake, K. (1995). Changes in the distribution of fibroblast growth factor in the teleostean testis during spermatogenesis. Genetics 277, 475–483. doi: 10.1002/jez.1402720609
Webster, L., Russell, M., Packer, G., and Moffat, C. F. (2006). Long term monitoring of polycyclic aromatic hydrocarbons (PAHs) in blue mussels (Mytilus edulis) from a remote scottish location. Polycycl. Aromat. Comp. 26, 283–298. doi. 10.1080/10406630600904109
White, K. L. Jr. (1986). An overview of immunotoxicology and carcinogenic polycyclic aromatic hydrocarbons. Environ. Carcino. Revs. C4, 163–202. doi: 10.1080/10590508609373342
White-Cooper, H., and Bausek, N. (2010). Evolution and spermatogenesis. Phil. Trans. R. Soc. 365, 1465–1480. doi: 10.1098/rstb.2009.0323
Working, P. K. (1988). Male reproductive toxicology: comparison of the human to animal models. Environ. Health. Persp. 77, 37–44. doi: 10.1289/ehp.887737
Yevich, P. P., and Barszcz, C. A. (1976). Gonadal and haematopoietic neoplasms in Mya arenaria. Mar. Fish. Rev. 38, 42–43.
Yurchenko, O. V., and Vaschenko, M. A. (2010). Morphology of spermatogenic and accessory cells in the mussel Modiolus kurilensis under environmental pollution. Mar. Environ. Res. 70, 171–180. doi: 10.1016/j.marenvres.2010.04.007
Zhou, J., Zhu, X., and Zhong-Hua, C. (2009). Endocrine disruptors: an overview and discussion on issues surrounding their impact on marine animals. J. Mar. Anim. Ecol. 2, 7–17. https://pdfs.semanticscholar.org/5588/7aabf3a41ba26929d1cd71c38f4c7eeb8c46.pdf
Keywords: Mytilus galloprovincialis, histopathology, tar, mussel farms, gonad
Citation: Alonso A, Suárez P, Ruiz Y, Dobal V and San Juan F (2019) Gonadal Histopathological Disorders in Mytilus galloprovincialis Male Exposed to Tars Used in Mussel Farms. Front. Mar. Sci. 6:577. doi: 10.3389/fmars.2019.00577
Received: 27 June 2019; Accepted: 30 August 2019;
Published: 11 September 2019.
Edited by:
Vincenzo Parrino, University of Messina, ItalyReviewed by:
Maurizio Manera, University of Teramo, ItalyTibor Kiss, Hungarian Academy of Sciences, Hungary
Copyright © 2019 Alonso, Suárez, Ruiz, Dobal and San Juan. This is an open-access article distributed under the terms of the Creative Commons Attribution License (CC BY). The use, distribution or reproduction in other forums is permitted, provided the original author(s) and the copyright owner(s) are credited and that the original publication in this journal is cited, in accordance with accepted academic practice. No use, distribution or reproduction is permitted which does not comply with these terms.
*Correspondence: Fuencisla San Juan, fsanjuan@uvigo.es