Biocontrol action of Trichothecium roseum against the wheat powdery mildew fungus Blumeria graminis f. sp. tritici
- 1College of Life Sciences, Henan Normal University, Xinxiang, China
- 2Henan International Joint Laboratory of Agricultural Microbial Ecology and Technology, Henan Normal University, Xinxiang, China
- 3State Key Laboratory of Wheat and Maize Crop Science, College of Plant Protection, Henan Agricultural University, Zhengzhou, China
- 4College of Horticulture and Plant Protection, Inner Mongolia Agricultural University, Hohhot, China
Trichothecium roseum is known to be a mycoparasite and inhibit phytopathogenic fungi. However, so far, only scarce information is available on the impacts of T. roseum on powdery mildews. Based on the morphological and molecular analysis, we identified T. roseum as a mycoparasite on colonies of the wheat powdery mildew fungus (Blumeria graminis f. sp. tritici, Bgt, recently clarified as B. graminis s. str.) and then showed that T. roseum was capable of efficiently impairing colony formation and conidial distribution of Bgt. After inoculation of T. roseum conidia on Bgt colonies, the biomasses of Bgt significantly decreased 1.46, 1.64, 7.55, and 10.49 times at 2, 4, 6, and 8 dpi, respectively. Thus, T. roseum, acting as a potential biological agent, impeded the developments of Bgt, making it a viable alternative for wheat powdery mildew control. Utilizing the Agrobacterium tumefaciens-mediated transformation (ATMT) system, a T. roseum strain that constitutively expressed green fluorescent protein was produced to improve the visualization of the T. roseum-Bgt interaction and showed direct hyphae interaction of T. roseum with Bgt structures during parasitic processes. These findings indicate that ATMT is a potent and efficient method for transforming T. roseum. Nevertheless, our results suggest that T. roseum is an antagonistic parasite of the wheat powdery mildew fungus, and hence, can be considered for phytopathogen management.
Background
Blumeria graminis f. sp. tritici (Bgt), recently clarified as B. graminis s. str., infests wheat and causes a destructive foliar disease (Liu et al., 2021). It inflicts severe economic losses and is regarded as the world's sixth most crucial fungal phytopathogen (Dean et al., 2012). Currently, chemical fungicides, which potentially harm human health and environment, have been widely used to control the wheat powdery mildew disease. However, chemical fungicide treatments cause powdery mildews to develop resistance (Safaei et al., 2022). Thus, environmentally friendly and human-friendly alternatives for controlling Bgt were in the focus of recent research (Kiss, 2003; Zhu et al., 2022a). Previously, Ampelomyces quisqualis, Pseudozyma flocculosa, Verticillium lecanii and Tilletiopsis spp. were the main biocontrol agents (BCAs) for powdery mildew diseases (Hajlaoui and Bélanger, 1993; Falk et al., 1995; Dik et al., 1998; Köhl et al., 2019). So far, commercial biological control products for efficiently controlling cereal powdery mildew are still in demand and thus the identification of novel BCAs is needed.
The Trichothecium genus consists of at least 77 species, which mostly are plant pathogenic fungi (http://www.mycobank.org/). T. roseum is reported as a primarily fungus causing postharvest disease that infest a variety of vegetables, fruits, and crops (Barnett and Hunter, 1972), including tomato, cucumber, orange, apple, mango, strawberry, Hami melon, peppers and maize (Yang et al., 2003; Kasuyama and Tanina, 2007; Dal Bello, 2008; Kwon et al., 2010; Inácio et al., 2011; Hamid et al., 2014; Lin et al., 2016; Xue et al., 2016; Li et al., 2022). T. roseum promotes fruit degradation after infection and then disrupts the long-term preservation of fruits and vegetables (Wei et al., 2018). While it is a pathogen on plants, T. roseum is also a BCA against multiple plant diseases, including fungi and insects (Zhang et al., 2010). It was reported that T. roseum, acting as a BCA, infected the psyllid insect Pauropsylla buxtoni and suppressed the gemination of Phakopsora pachyrhizi uredospore (Kumar and Jha, 2002; Batta, 2020). Moreover, T. roseum was shown to be a BCA against plant phytopathogens, such as Sclerotinia sclerotiorum, Rhizoctonia solani and Penicillium digitatum (Freeman and Morrison, 1949; Huang and Kokko, 1993; Tesfagiorgis and Laing, 2010). However, little is known about the capability of T. roseum on efficiently parasitizing and/or suppressing powdery mildew fungi, including Bgt.
Identification of parasites that are capable of inhibiting cereal phytopathogens is crucial for developing BCAs against these diseases. In this study, we reported the discovery and identification of a T. roseum strain isolated from Bgt-infested wheat leaves under natural environmental conditions. Since there is little information available on the mycoparasitism and/or biocontrol effects of T. roseum on powdery mildews, we examined the disease-suppressive properties of T. roseum on Bgt developments. To enhance the visualization of the interactions between T. roseum and Bgt, a green fluorescent protein (GFP)-transformed strain of T. roseum was constructed using the Agrobacterium tumefaciens-mediated transformation (ATMT) system.
Materials and methods
Fungal and plant materials
Wheat seeds (Triticum aestivum L. cv. Aikang 58) were sown in plastic pots (Ø 9 cm) with sterilized nutrient soil (including peat soil, vermiculite and perlite) and pots were placed in growth chambers (temperature, 22°C; humidity, 70%; light/dark, 16 h/8 h) (Zhu et al., 2022b). Blumeria graminis f. sp. tritici (Bgt) (isolate Bgtzm2022) was propagated on its host wheat leaves under the same conditions. One day before inoculation, the powdery mildew-infected leaves were shaken to obtain fresh spores.
The mycoparasite was collected from mildew-infected wheat grown at Henan Normal University in 2021. And the single spore of the mycoparasite was isolated. To obtain a pure strain, individual spores of the mycoparasite were isolated and transferred to PDA and incubated at 25°C in the dark (Zhu et al., 2020a).
Microscopic observation
In order to morphologically identify the isolated mycoparasite, the fungus was firstly observed by light microscopy (Sunny Optical, EX30, Zhejiang, China), then the spores and mycelial structure were observed by scanning electron microscopy (SEM) (Hitachi TM3030Plus, Japan) according to a previously described method (Zhu et al., 2020a).
DNA extraction and amplification
The total genomic DNA of the mycoparasite was extracted according to the cetyltrimethylammonium bromide (CTAB) method (Rogers and Bendich, 1994). The mycoparasite ribosomal ITS sequence was amplified by polymerase chain reaction (PCR) with universal primers, ITS1 and ITS4 (White et al., 1990). Reaction mixture composition of PCR include 2X M5 HiPer plus Taq HiFi PCR mix (25 μL), Forward primer: ITS1 (5′-TCCGTAGGTGAACCT0GCGG-3′) (2.5 μL), Reverse primer: ITS4 (5′-TCCTCCGCTTATTGATATGC-3′) (2.5 μL), templete (1 μL), ddH2O (19 μL). PCR was conducted on a C1000 TouchTM Thermal Cycler (Bio-Rad, Hercules, California, United States), applying following program: 94°C for 5 min, followed by 35 cycles of 94°C for 30 s, 54°C for 30 s, 72°C for 1 min, and final elongation at 72°C for 5 min. 1.5% agarose gel was used for electrophoresis detection of PCR products. The amplicon was sequenced (Invitrogen, Shanghai, China) and the obtained sequence was deposited into GenBank with Accession No. MZ292093.
Phylogenetic analysis
In order to further identify T. roseum, a phylogenetic tree of Trichothecium spp. ITS sequences was built. Sequences were retrieved and analyzed in MEGA software. The phylogenetic tree is constructed according to Maximum likelihood method with the options as 1000 bootstrap method, Tamura-Nei model, 50% site coverage cut off. The ITS sequences of Arthrociadiella mougeotii and Blumeria graminis were applied as outgroups (Zhu et al., 2020b, 2021a).
Hyperparasitism assays
To determine whether T. roseum was a mycoparasite, conidia of Bgt were inoculated onto healthy wheat leaves (cv. Aikang 58). Six days post inoculation (dpi), mildew-infected plants were inoculated with spore suspension (concentration 1 × 106 spores mL−1) of the identified T. roseum strain and kept in a growth chamber. Bgt-inoculated plants treated with water were served as controls. At 2, 4, 6, and 8 dpi, the leaves were collected for microscopic analysis. Then the leaves were cut to 2 cm in size for discoloring and the fungal structures were stained with trypan blue according to a previous study and observed under a light microscopy (Sunny Optical, EX30, Zhejiang, China) to morphologically identify the fungal characteristics (Zhu et al., 2017). Meanwhile the leaves were air-dried for observation by the SEM.
Fungal biomass determination
To determine whether Bgt biomasses were affected by T. roseum, mildew-infested leaves were treated with water or T. roseum spore suspension. At 2, 4, and 6 dpi, leaves were collected and total DNA was isolated using a previously described method (Zhu et al., 2019). The elongation factor 1 alpha of wheat, T. roseum and Bgt was amplified with primers TaEF1 (wheat elongation factor 1, TaEF1-F: TGGTGTCATCAAGCCTGGTATGGT and TaEF1-R: ACTCATGGTGCATCTCAACGGACT), TrEF2 (T. roseum elongation factor 2, TrEF2-F: CGTCGCTTCTGACTCCAAGA and TrEF2-R: AGCCTCAACAGCCTTACCAG) and BgtEF1 (Bgt elongation factor 1, BgtEF1-F: AAGCTAAAGGCCGAACGTGA and BgtEF1-R: GCACAGTCAGCTTGAGAGGT), respectively (Coram et al., 2008; Hu et al., 2018). Each of the sequences was separately fused into the pMD™19-T vector (Takara, Japan). Real time PCR mixture [2x AceQ qPCR SYBR Green Master Mix (7.5 μL, Vazyme), forward primer (0.3 μL), reverse primer (0.3 μL), DNA Templete (1.5 μL), ddH2O (5.4 μL)] was used for anlyzing. RT-qPCR experiments were conducted on a LightCycler 96 real-time PCR instrument (Roche, Switzerland) as followings processes: initiated at 95°C for 15 min, followed by 45 cycles at 95°C for 10 s and 60°C for 30 s. The fungal BgtEF1, TrEF1, and TaEF1 plasmids were diluted into a serial concentration for generation of the standard curves. Ct means were determined using qPCR. The Bgt, T. roseum and wheat DNA concentrations were calculated according to the corresponding standard curves. Then T. roseum and Bgt biomass changes were calculated based on the DNA concentration ratios of T. roseum/wheat or Bgt/wheat according to a previously reported method (Zhu et al., 2022a). The data was obtained from three (n = 3) independent biological replicates.
Green fluorescent protein transformation and observation of T. roseum
To transform T. roseum, Agrobacterium tumefaciens strain GV3101 was transferred with the binary vector pPK2Tgfp (Martínez-Cruz et al., 2017) and then grown for 24 h at 28°C in 2 ml of YEP medium (Yeast Extract Peptone Broth Medium) containing 50 μg ml−1 kanamycin and 50 μg ml−1 gentamicin in an orbital shaker at 140 rpm. The suspension of transferred Agrobacterium tumefaciens was directly pipetted onto T. roseum (strain ZM-Tr2021) grown on PDA and incubated for 4 days at room temperature (Zhu et al., 2022c). Then the spores of T. roseum were inoculated onto PDA medium containing 50 μg ml−1 carbendazim and 100 μg ml−1 cefotaxime and incubated for 10 days at room temperature. The newly formed colonies were selected as transformed T. roseum and used for further experiments.
To observe the interaction of T. roseum and Bgt, mildew-infested wheat leaves were treated with GFP-transformed T. roseum and incubated for 6 days under the same condition described above. The structures of T. roseum and Bgt were observed with a fluorescence microscope (BX63, Olympus, Japan).
Results
Identification of T. roseum as a mycoparasite in Bgt colony
Initially a mycoparasite on the wheat powdery mildew fungus Blumeria graminis f. sp. tritici (Bgt) was found. To identify the mycoparasite, the morphological characteristics and genetical analysis were conducted. The colonies of the fungus on PDA were granular pink and white, with dark pink on the reverse side (Figures 1A,B). Conidia were hyaline, two-celled, and single-septate, ellipsoid to pyriform (15–25 × 8 to 12 μm), with truncation in the middle, and produced in clusters. Mycelia displayed hyaline hyphae and slender, 2–4 μm in diameter, and conidiophores were branched (Figure 1C). According to the morphological characteristics, the pathogen was initially identified as Trichothecium roseum.
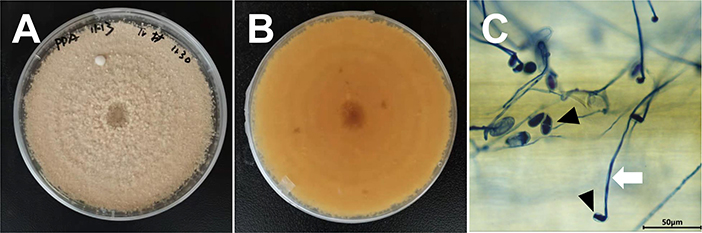
Figure 1. Morphological characteristics of T. roseum. (A,B) T. roseum colonies on PDA 12 dpi. (C) Mycelia and conidia of T. roseum on Bgt colonies. White arrow indicated mycelia of T. roseum. Black arrow indicated conidia of T. roseum.
The ITS region of the mycoparasite rDNA was PCR-amplified. The sequence of the amplicon (accession No. MZ292093) was 613 bp and showed 100% identity to a reported T. roseum (EU552162) strain on Leucadendron xanthoconus. To conduct phylogenetic analyse, the ITS sequences of Trichothecium sp. were retrieved and applied for phylogenetic analysis. The phylogenetic tree clearly showed that T. roseum (EU552162) and this identified fungus were clustered in the same branch (Figure 2). Therefore, the isolated mycoparasite was identified and confirmed as T. roseum based on the morphological characteristics and molecular analysis.
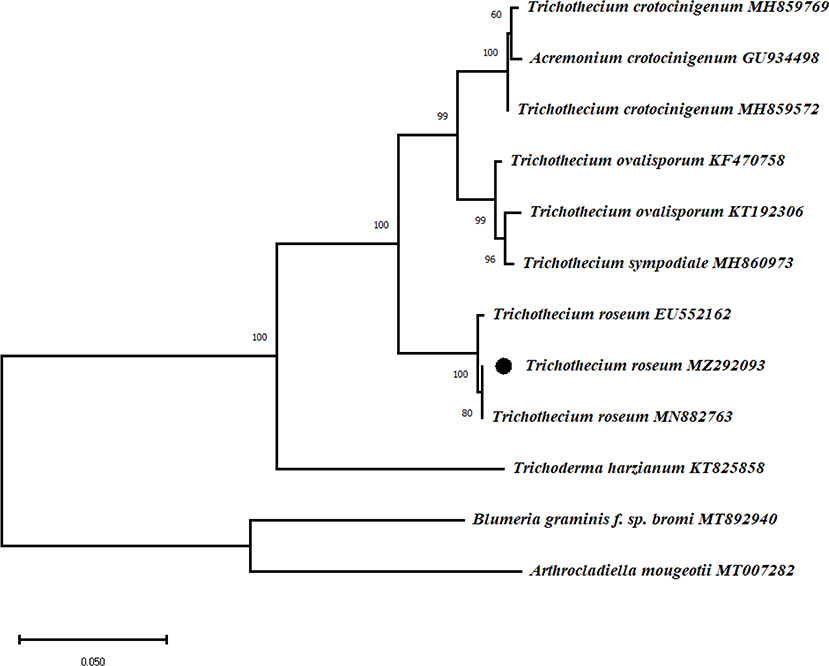
Figure 2. Phylogenetical analysis of T. roseum and Trichothecium sp. The maximum likelihood tree was constructed in MEGA software with 1000 bootstrap replicates and Tamura-Nei model. The bar indicates a distance of 0.050. Bold words highlighted T. roseum. Arthrocladiella mougeotii and Blumeria graminis f. sp. bromi were included as outgroups.
The mycoparasitical action of T. roseum on Bgt colonies
To test whether T. roseum was capable of parasiting on Bgt colonies, spore suspension of T. roseum or water was separately sprayed on Bgt-infected of wheat leaves and incubated for 8 days (Figure 3). Compared to the groups (water treatment), colonies of Bgt turned from white to dark and conidiophores were collapsed. By development on Bgt colonies, structures of T. roseum covered on Bgt colonies and interacted with Bgt conidia (Figures 3A–C,E–G). The SEM analysis showed the same results: conidiophores and conidia of Bgt were twined and destroyed by T. roseum structures (Figures 3D,H). In addition, T. roseum did not infect healthy wheat (Supplementary Figure 1). Therefore, the mycoparasitical action of T. roseum was confirmed.
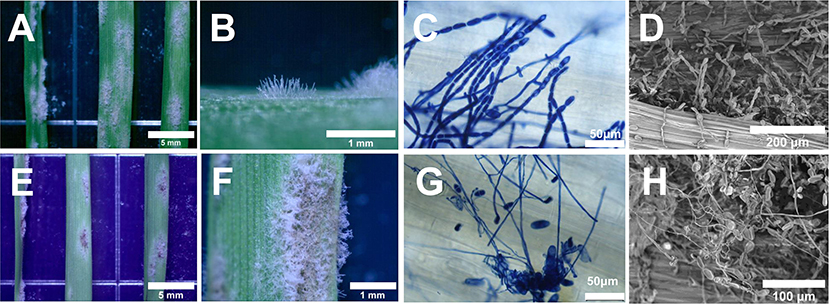
Figure 3. Morphological characteristics of Bgt and T. roseum colonies during interaction. (A–D) Water-treated Bgt colonies. (E–H) Bgt colonies treated with T. roseum. The colonies were monitored under stereo microscopy (A,B,E,F), light microscopy (C,G) and scanning microscopy (D,H). The scale bars were 5 mm in (A,E), 1 mm in (B,F), 50 μm in (C,G), 200 μm in (D) and 100 μm in (H).
The developments of T. roseum on Bgt
To further determine the developmental processes of T. roseum on Bgt, a mycoparasitism experiment was performed. Comparing to the control groups (Bgt treated with water) (Figures 4A–C), T. roseum started to form mycelia at 2 dpi, produced conidia at 4 dpi and extensively formed fungal strutures at 6 dpi. At the meantime, Bgt conidiophores and conidia were collapsed by T. roseum (Figures 4D–F).
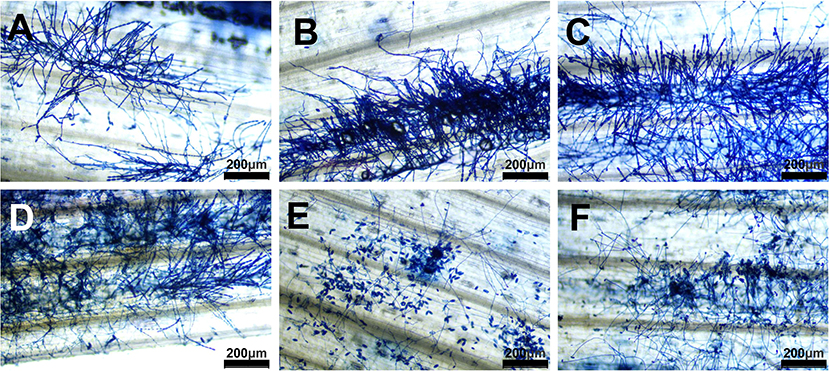
Figure 4. Colonies of Bgt with or without T. roseum interactions. (A–C) Bgt colonies treated with water at 2 dpi, 4 dpi and 6 dpi, respectively. (D–F) Bgt colonies treated with T. roseum suspension at 2, 4, and 6 dpi, respectively. Scale bars in (A–F) were 200 μm.
T. roseum directly decreases biomasses of Bgt on wheat leaves
To quantitatively measure the biomass changes during the interactions, mildew-infested wheat leaves were inoculated with T. roseum and the biomasses of both fungi were quantified using RT-qPCR (Figures 5A–C). During the growth of T. roseum, the biomasses of T. roseum notably increased 1.74 times at 2 dpi, 366.36 times at 4 dpi, 1,458 at 6 dpi and 6,964 at 8 dpi when compared to that at 0 dpi (Figure 5D). In contrast, the biomasses of Bgt significantly decreased 1.46 times at 2 dpi, 1.64 time at 4 dpi, 7.55 times at 6 dpi and 10.49 times at 8 dpi when compared to that at 0 dpi (Figure 5E).
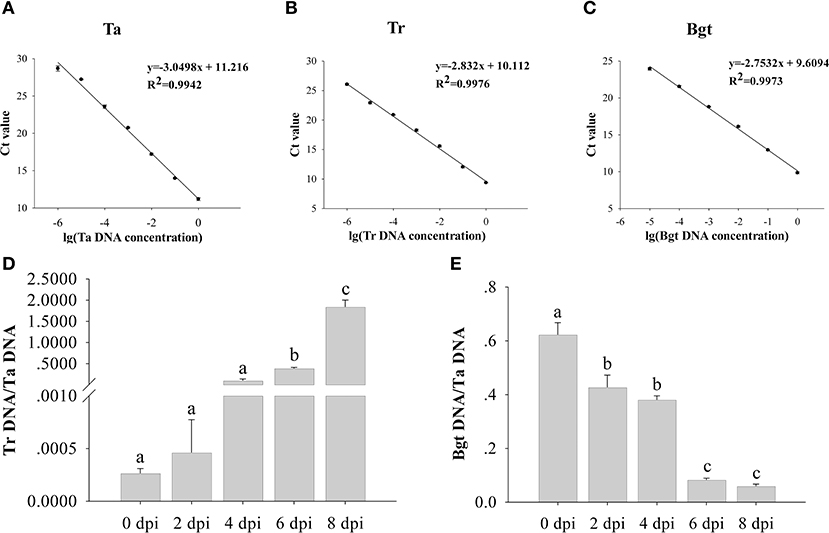
Figure 5. Biomass changes of Blumeria graminis f. sp. tritici (Bgt) and T. roseum during interactions. The DNA concentration standard curves of wheat (Ta) (A), T. roseum (Tr) (B) and B. graminis f. sp. tritici (Bgt) (C) were used to calculate the biomasses of T. roseum (D) and Bgt (E). The relative quantities of PCR product of TaEF1, TrEF1, and BgtEF1 in infected samples were calculated using the gene-specific standard curves to quantify wheat, T. roseum and Bgt gDNA. The standard curves derived from the serial dilutions were built utilizing the primers of elongation factors of Ta, Tr, and Bgt, respectively. Each value is given as mean ± SD of three independent biological experiments. Significant differences were determined in a One-way ANOVA test, with post-hoc Tukey test: different letters indicate significant differences (P < 0.05).
Green fluorescent protein transformation of T. roseum
To enhance the visualization of the mycoparasitism interaction between T. roseum and Bgt, spores of GFP transformed and wild type (untransformed) T. roseum were separately inoculated onto mildew-infested wheat leaves and examined under a fluorescence microscope. The GFP-transformed T. roseum strain showed direct interaction with Bgt structures (Figures 6A–C). In some cases, Bgt conidiophores and conidia were observed with fluorescent signals (Figures 6D–F). In addition, the mycoparasitical activities of the GFP-transformed T. roseum strain was not affected when compared to the wild-type strain.
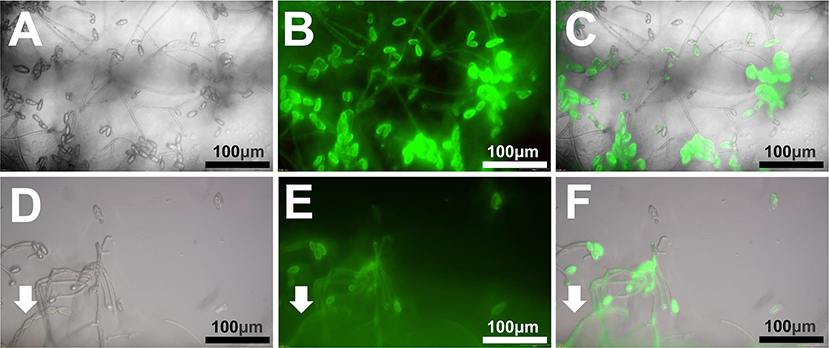
Figure 6. Visualization of interaction between GFP transformed T. roseum and Bgt. (A,D) Bright field. (B,E) Fluorescence field. (C,F) Merged images. The arrow heads indicate a conidiophore of Bgt. Scale bars = 50 μm.
Discussion
Identification of a hyperparasite on Blumeria graminis f. sp. tritici colony
T. roseum has been repeatedly reported as a fungus causing postharvest disease on a variety of fruits and vegetables (Yang et al., 2003; Kasuyama and Tanina, 2007; Dal Bello, 2008; Kwon et al., 2010; Inácio et al., 2011; Hamid et al., 2014; Lin et al., 2016; Tang et al., 2016; Xue et al., 2016; Li et al., 2022). In addition, T. roseum was shown to be a potential agent for biological control of plant fungal diseases (including soybean rust) and Pauropsylla buxtoni, a disease-causing insect (Freeman and Morrison, 1949; Huang and Kokko, 1993; Kumar and Jha, 2002; Tesfagiorgis and Laing, 2010; Batta, 2020). However, to date, there is no information available concerning the antagonistic effects of T. roseum on the developments of the economically and agriculturally important powdery mildew pathogens, including Bgt.
Initially, we isolated a fungus with suspected mycoparasitic potential from mildew-infected wheat leaves. Due to the fact that the morphological and molecular characterization of this mycoparasite was consist with previously reported T. roseum (Marincowitz et al., 2008; Tang et al., 2015), we identified and confirmed that the mycoparasite was T. roseum (Figures 1, 2).
To test the mycoparasitic actions of T. roseum on Bgt, T. roseum spore suspension was then sprayed on the mildew-infested wheat leaves. Our results showed distinct interactions between T. roseum and Bgt (Figure 3), suggesting T. roseum was capable of parasitizing on powdery mildews. Therefore, we, for the first time, illustrated that T. roseum was a mycoparasite of Bgt and that, in agreements with previous studies, T. roseum was a natural biocontrol agent against phytopathogens under field and laboratory conditions.
T. roseum mediated suppression of Bgt conidial formation and distribution
Bumeria graminis is an obligate biotrophic ascomycete that causes powdery mildews on various plant species (Liu et al., 2021; Zhu et al., 2021a,b). To determine the inhibitory effect of T. roseum on Bgt, we observed the growth of Bgt at 2, 4, and 6 d after spraying T. roseum spore suspension, and found that Bgt spores collapsed significantly and the mycelium of T. roseum entangled on Bgt (Figure 4). In previous studies on Bgt biocontrol, Sporothrix flocculosa was shown to be able to grow rapidly and produce large numbers of spores on wheat powdery mildew spore clusters within 12 h, and to cause collapse of the powdery mildew conidial chain and inhibit conidial production within 24 h (Hajlaoui and Bélanger, 1993). Our experimental results also showed that the parasitization of T. roseum caused the collapse of the conidia chains of Bgt. T. roseum directly wrapped around mildew conidiophores at 2 dpi, erupted hyphae from mildew colonies at 4 dpi and disrupted conidia distribution of Bgt from 4 dpi (Figure 4). In addition, the biomasses of T. roseum notably increased during mycoparasitic processes (Figure 5D). However, the biomasses of Bgt significantly reduced by inoculation of T. roseum (Figure 5E). These results suggested that T. roseum was able to efficiently control Bgt. Previously, T. roseum was reported to inhibit uredospore germination of the soybean rust fungus Phakopsora pachyrhizi and to entomopathogenically infect Pauropsylla buxtoni on fig (Ficus carica) leaves (Kumar and Jha, 2002; Batta, 2020). Secondary metabolites of T. roseum were found to be active in suppressing the disease-causing fungus (Jayaprakashvel et al., 2010). However, whether the secondary metabolites of T. roseum have the same inhibitory effects on Bgt is unclear. Further studies on secondary metabolites of T. roseum will provide more insights into the suppression effects of this mycoparasite on phytopathogens.
Green fluorescent protein transformation of T. roseum by the Agrobacterium tumefaciens-mediated transformation
Previously, the transformation of T. roseum with fluorescent proteins was based on the PEG-mediated protoplast transformation method (Dai et al., 2019, 2020; Wang et al., 2022). Agrobacterium tumefaciens-mediated transformation (ATMT) methods were repeatedly utilized for transfer GFP into biocontrol agents to reveal the interactions between parasites and powdery mildews (Németh et al., 2019). However, so far, to the best of our knowledge, the method of transferring GFP fluorescent protein in T. roseum and making it stably expressed using the ATMT method has not been reported. In our study, to enhance the visualization of the interaction between T. roseum and Bgt, we, for the first time, used the agrobacterium to transfer GFP into T. roseum and obtained a strain with stably expressed GFP fluorescent signal (Figure 6). This indicates that T. roseum can be transformed by the ATMT method. Our previous study has demonstrated the chromosome-scale genome sequence of T. roseum (Zhu et al., 2022c). Genetic overexpression and/or knockout is potent for analyzing the roles of specific genes. Therefore, functions of genes and proteins involved in pathogenicity, mycoparasitism and metabolism can be deeply mined combining the transformation method and genome sequence.
Conclusions
Screening and identification of mycoparasites on crop pathogenic fungi is crucial for exploring new biological control agents of crop diseases and for understanding the biodiversity of hyperparasites. Our study provides new insights into the fungal host range and developmental processes of T. roseum and helps to fill knowledge gaps in the understanding of the interaction between T. roseum and Bgt. The major findings of this study are as follows: (1) T. roseum directly parasitizes on the wheat powdery mildew and suppresses conidiophore formation and conidial distribution of Bgt; (2) T. roseum decreases the biomasses of powdery mildew during mycoparasitic processes, suggesting its potential utilization in the control of wheat powdery mildew; (3) by using the ATMT method, the visualization of T. roseum structures is improved with GFP transformation.
Data availability statement
The datasets presented in this study can be found in online repositories. The names of the repository/repositories and accession number(s) can be found below: https://www.ncbi.nlm.nih.gov/nuccore/MZ292093, MZ292093.
Author contributions
MZ designed and conceived of the study and wrote the initial manuscript. XD, PC, WZ, and YL participated in the experiments, performed the statistical analysis, and prepared the figures and tables. JC, ZL, and ZQ conceived of the study and critically edited the whole manuscript. All authors read and approved the final manuscript.
Funding
This work was financially supported by Joint Fund of Science and Technology Development Program of Henan Province (222301420112), Foundation for the Key Research Program of Higher Education of Henan (23A210004), the Scientific and Technological Project of Henan Province (222102110230), Open Project of Key Laboratory of Integrated Pest Management on Crops in Central China, Ministry of Agriculture and Rural Affairs, P. R. China and Hubei Key Laboratory of Crop Disease, Insect Pests and Weeds Control (2022ZTSJJ2) and Open Project of research on typical aquatic plant remediation techniques for rural water pollution (FIRI20210301), the HAU grant for collaborative crop science research (CCSR2022-1), and Science and Technology Project of Inner Mongolia (2022YFDZ0029).
Acknowledgments
We would like to thank Prof. Alejandro Pérez-García from University of Malaga for sharing the vector pPK2Tgfp and Prof. Zhengang Ru from Henan Institute of Science and Technology for sharing the wheat (cv. Aikang 58) seeds.
Conflict of interest
The authors declare that the research was conducted in the absence of any commercial or financial relationships that could be construed as a potential conflict of interest.
Publisher's note
All claims expressed in this article are solely those of the authors and do not necessarily represent those of their affiliated organizations, or those of the publisher, the editors and the reviewers. Any product that may be evaluated in this article, or claim that may be made by its manufacturer, is not guaranteed or endorsed by the publisher.
Supplementary material
The Supplementary Material for this article can be found online at: https://www.frontiersin.org/articles/10.3389/fsufs.2022.998830/full#supplementary-material
References
Barnett, H. L., and Hunter, B. B. (1972). Illustrated Genera of Imperfect Fungi. 3rd Edn. Minneapolis: Burgess Publishing Co.
Batta, Y. (2020). Entomopathogenic effect of Trichothecium roseum (Pers.) Link (Hypocreales: Ascomycota) against Pauropsylla buxtoni (Psylloidea: Hemiptera) infesting Ficus carica leaves and its potential use as biocontrol agent of the insect. J. Appl. Microbiol. 129, 400–410. doi: 10.1111/jam.14613
Coram, T. E., Wang, M., and Chen, X. (2008). Transcriptome analysis of the wheat–Puccinia striiformis f. sp. tritici interaction. Mol. Plant Pathol. 9, 157–169. doi: 10.1111/j.1364-3703.2007.00453.x
Dai, P., Jiang, Y., Liang, X., Gleason, M. L., Zhang, R., and Sun, G. (2020). Trichothecium roseum enters ‘Fuji' apple cores through stylar fissures. Plant Dis. 104, 1060–1068. doi: 10.1094/PDIS-07-19-1559-RE
Dai, P., Liang, X., Zhang, R., and Sun, G. (2019). Construction of Trichothecium roseum GFP tagged strains based on PEG-mediated protoplast transformation. Mycosystema. 38, 90–97. doi: 10.13346/j.mycosystema.180259
Dal Bello, G. (2008). First report of Trichothecium roseum causing postharvest fruit rot of tomato in Argentina. Aust. Plant Dis. Notes 3, 103–104. doi: 10.1007/BF03211257
Dean, R., Kan, J., Pretorius, Z. A., Hammond-Kosack, K. E., Pietro, A. D., Spanu, P. D., et al. (2012). The top 10 fungal pathogens in molecular plant pathology. Mol. Plant Pathol. 13, 414–430. doi: 10.1111/j.1364-3703.2011.00783.x
Dik, A. J., Verhaar, M. A., and Bélanger, R. R. (1998). Comparison of three biological control agents against cucumber powdery mildew (Sphaerotheca fuliginea) in semi-commercial-scale glasshouse trials. Eur. J. Plant Pathol. 104, 413–423. doi: 10.1023/A:1008025416672
Falk, S. P., Gadoury, D. M., Pearson, R. C., and Seem, R. C. (1995). Partial control of grape powdery mildew by the mycoparasite Ampelomyces quisqualis. Plant Dis. 79, 483–490. doi: 10.1094/PD-79-0483
Freeman, G., and Morrison, R. (1949). The isolation and chemical properties of trichothecin, an antifungal substance from Trichothecium roseum link. Biochem. J. 44, 1. doi: 10.1042/bj0440001
Hajlaoui, M., and Bélanger, R. (1993). Antagonism of the yeast-like phylloplane fungus Sporothrix flocculosa against Erysiphe graminis var tritici. Biocontrol Sci. Technol. 3, 427–434. doi: 10.1080/09583159309355297
Hamid, M. I., Hussain, M., Ghazanfar, M. U., Raza, M., and Liu, X. Z. (2014). Trichothecium roseum causes fruit rot of tomato, orange, and apple in Pakistan. Plant Dis. 98, 1271–1271. doi: 10.1094/PDIS-01-14-0051-PDN
Hu, Y., Liang, Y., Zhang, M., Tan, F., Zhong, S., Li, X., et al. (2018). Comparative transcriptome profiling of Blumeria graminis f. sp. tritici during compatible and incompatible interactions with sister wheat lines carrying and lacking Pm40. PLoS ONE 13, e0198891. doi: 10.1371/journal.pone.0198891
Huang, H., and Kokko, E. (1993). Trichothecium roseum, a mycoparasite of Sclerotinia sclerotiorum. Can. J. Bot. 71, 1631–1638. doi: 10.1139/b93-198
Inácio, C. A., Pereira-Carvalho, R. C., Morgado, F. G. A., Fonseca, M. E. N., and Boiteux, L. S. (2011). A tomato fruit rot caused by Trichothecium roseum in Brazil. Plant Dis. 95, 1318–1318. doi: 10.1094/PDIS-06-11-0464
Jayaprakashvel, M., Selvakumar, M., Srinivasan, K., Ramesh, S., and Mathivanan, N. (2010). Control of sheath blight disease in rice by thermostable secondary metabolites of Trichothecium roseum MML003. Eur. J. Plant Pathol. 126, 229–239. doi: 10.1007/s10658-009-9535-y
Kasuyama, S., and Tanina, K. (2007). Pink-Mold Rot of Cucumber (Cucumis sativus L.) caused by Trichothecium roseum. Bulletin of the Agricultural Experiment Station, Okayama Prefectural General Agriculture Center (Japan).
Kiss, L. (2003). A review of fungal antagonists of powdery mildews and their potential as biocontrol agents. Pest Manage. Sci. 59, 475–483. doi: 10.1002/ps.689
Köhl, J., Goossen-van de Geijn, H., Groenenboom-de Haas, L., Henken, B., Hauschild, R., Hilscher, U., et al. (2019). Stepwise screening of candidate antagonists for biological control of Blumeria graminis f. sp. tritici. Biol. Control 136, 104008. doi: 10.1016/j.biocontrol.2019.104008
Kumar, S., and Jha, D. K. (2002). Trichothecium roseum: a potential agent for the biological control of soybean rust. Indian Phytopathol. 55, 232–234.
Kwon, J.-H., Shen, S.-S., and Kim, J.-W. (2010). Occurrence of pink mold rot of strawberry caused by Trichothecium roseum in Korea. Plant Pathol. J. 26, 296–296. doi: 10.5423/PPJ.2010.26.3.296
Li, Y., Liu, Y., Zhang, Z., Shen, K., Hao, J., Luo, L., et al. (2022). Characterization of the host range and sensitivity to fungicides of Trichothecium spp. associated with fruit rot in the field and in storage. Plant Pathol. 71, 1142–1151. doi: 10.1111/ppa.13550
Lin, S. H., Wang, J. H., and Wu, A. B. (2016). A post-harvest pink rot of pepper caused by Trichothecium roseum in China. Plant Dis. 100, 2164–2164. doi: 10.1094/PDIS-02-16-0246-PDN
Liu, M., Braun, U., Takamatsu, S., Hambleton, S., Shoukouhi, P., Bisson, K. R., et al. (2021). Taxonomic revision of Blumeria based on multi-gene DNA sequences, host preferences and morphology. Mycoscience 62, 143–165. doi: 10.47371/mycosci.2020.12.003
Marincowitz, S., Crous, P., Groenewald, J. Z., and Wingfield, M. (2008). Microfungi occurring on Proteaceae in the fynbos. CBS Biodiversity Series 7, 1–166.
Martínez-Cruz, J., Romero, D., de Vicente, A., and Pérez-García, A. (2017). Transformation of the cucurbit powdery mildew pathogen Podosphaera xanthii by Agrobacterium tumefaciens. New Phytologist 213, 1961–1973. doi: 10.1111/nph.14297
Németh, M. Z., Pintye, A., Horváth, Á. N., Vági, P., Kovács, G. M., Gorfer, M., et al. (2019). Green fluorescent protein transformation sheds more light on a widespread mycoparasitic interaction. Phytopathology 109, 1404–1416. doi: 10.1094/PHYTO-01-19-0013-R
Rogers, S. O., and Bendich, A. J. (1994). “Extraction of total cellular DNA from plants, algae and fungi,” in Plant Molecular Biology Manual, eds S. B. Gelvin and R. A. Schilperoort (Netherlands: Springer), 183–190.
Safaei, M., Jorkesh, A., and Olfati, J. (2022). Chemical and biological products for control of powdery mildew on cucumber. Int. J. Vegetable Sci. 28, 233–238. doi: 10.1080/19315260.2021.1935388
Tang, Q., Fang, L., and Liu, Y. J. (2015). First report of Trichothecium roseum causing trunk disease of Acer buergerianum in China. Plant Dis. 99, 1864–1864. doi: 10.1094/PDIS-05-15-0595-PDN
Tang, Q., Liu, Y. J., and Fang, L. (2016). Trichothecium roseum causes trunk disease of Sapindus mukorossi in China. Plant Dis. 100, 1009. doi: 10.1094/PDIS-10-15-1175-PDN
Tesfagiorgis, H. B., and Laing, M. D. (2010). Isolation and in vitro screening of potential biocontrol agents against powdery mildew of zucchini caused by Podosphaera xanthii. South Afr. J. Plant Soil 27, 179–186.
Wang, B., Han, Z., Gong, D., Xu, X., Li, Y., Sionov, E., et al. (2022). The pH signalling transcription factor PacC modulate growth, development, stress response and pathogenicity of Trichothecium roseum. Environ. Microbiol. 24, 1608–1621. doi: 10.1111/1462-2920.15943
Wei, M., Ge, Y., Li, C., Chen, Y., Wang, W., Duan, B., et al. (2018). Antifungal activity of ε-poly-L-lysine on Trichothecium roseum in vitro and its mechanisms. Physiol. Mol. Plant Pathol. 103, 23–27. doi: 10.1016/j.pmpp.2018.04.005
White, T. J., Bruns, T., Lee, S., and Taylor, J. (1990). Amplification and direct sequencing of fungal ribosomal RNA genes for phylogenetics. PCR Protocols Guide Methods Appl. 18, 315–322. doi: 10.1016/B978-0-12-372180-8.50042-1
Xue, C. S., Xu, J. N., Sun, J. Y., Lu, Y. Y., Li, G. F., and Xiao, S. Q. (2016). First report of Trichothecium roseum causing maize (Zea mays) ear rot in Northern China. Plant Dis. 100, 2324–2324. doi: 10.1094/PDIS-03-16-0358-PDN
Yang, B., Shiping, T., Hongxia, L., Jie, Z., Jiankang, C., Yongcai, L., et al. (2003). Effect of temperature on chilling injury, decay and quality of Hami melon during storage. Postharvest Biol. Technol. 29, 229–232. doi: 10.1016/S0925-5214(03)00104-2
Zhang, X., Li, G., Ma, J., Zeng, Y., Ma, W., and Zhao, P. (2010). Endophytic fungus Trichothecium roseum LZ93 antagonizing pathogenic fungi in vitro and its secondary metabolites. J. Microbiol. 48, 784–790. doi: 10.1007/s12275-010-0173-z
Zhu, M., Duan, X., Cai, P., Li, Y.-,f., and Qiu, Z. (2022a). Deciphering the genome of Simplicillium aogashimaense to understand its mechanisms against the wheat powdery mildew fungus Blumeria graminis f. sp. tritici. Phytopathol. Res. 4, 16. doi: 10.1186/s42483-022-00121-5
Zhu, M., Duan, X., Cai, P., Qiu, Z., and Li, Z-N. (2022c). Genome sequence resource of Trichothecium roseum (ZM-Tr2021), the causal agent of Postharvest Pink Rot. Plant Dis (Accepted). doi: 10.1094/PDIS-03-22-0655-A
Zhu, M., Duan, X., Zeng, Q., Cai, P., Shi, W., and Qiu, Z. (2022b). Podosphaera xanthii causing powdery mildew on Impatiens balsamina in China. Can. J. Plant Pathol. 44, 354–360. doi: 10.1080/07060661.2021.2011421
Zhu, M., Ji, J., Duan, X., Shi, W., and Li, Y.-F. (2021a). First report of powdery mildew caused by Blumeria graminis f. sp. bromi on Bromus catharticus in China. Plant Dis. 105, 1211. doi: 10.1094/PDIS-09-20-1983-PDN
Zhu, M., Ji, J., Shi, W., and Li, Y.-F. (2021b). Occurrence of powdery mildew caused by Blumeria graminis f. sp. poae on Poa pratensis in China. Plant Dis. 105, 1212–1212. doi: 10.1094/PDIS-09-20-2051-PDN
Zhu, M., Ji, J., Wang, M., Zhao, M., Yin, Y., Kong, J., et al. (2020a). Cuticular wax of mandarin fruit promotes conidial germination and germ tube elongation, and impairs colony expansion of the green mold pathogen, Penicillium digitatum. Postharvest Biol. Technol. 169, 111296. doi: 10.1016/j.postharvbio.2020.111296
Zhu, M., Riederer, M., and Hildebrandt, U. (2017). Very-long-chain aldehydes induce appressorium formation in ascospores of the wheat powdery mildew fungus Blumeria graminis. Fungal Biol. 121, 716–728. doi: 10.1016/j.funbio.2017.05.003
Zhu, M., Riederer, M., and Hildebrandt, U. (2019). UV-C irradiation compromises conidial germination, formation of appressoria, and induces transcription of three putative photolyase genes in the barley powdery mildew fungus, Blumeria graminis f. sp. hordei. Fungal Biol. 123, 218–230. doi: 10.1016/j.funbio.2018.12.002
Keywords: biological control, Trichothecium roseum, fungal identification, wheat, powdery mildew fungus, Blumeria graminis, Agrobacterium tumefaciens-mediated transformation, fluorescent protein
Citation: Zhu M, Duan X, Cai P, Zhang W, Liu Y, Cui J, Li Z and Qiu Z (2022) Biocontrol action of Trichothecium roseum against the wheat powdery mildew fungus Blumeria graminis f. sp. tritici. Front. Sustain. Food Syst. 6:998830. doi: 10.3389/fsufs.2022.998830
Received: 20 July 2022; Accepted: 24 October 2022;
Published: 15 November 2022.
Edited by:
Pedro Talhinhas, University of Lisbon, PortugalReviewed by:
Ou Sheng, Guangdong Academy of Agricultural Sciences (GDAAS), ChinaDiána Seress, ELKH, Hungary
Copyright © 2022 Zhu, Duan, Cai, Zhang, Liu, Cui, Li and Qiu. This is an open-access article distributed under the terms of the Creative Commons Attribution License (CC BY). The use, distribution or reproduction in other forums is permitted, provided the original author(s) and the copyright owner(s) are credited and that the original publication in this journal is cited, in accordance with accepted academic practice. No use, distribution or reproduction is permitted which does not comply with these terms.
*Correspondence: Mo Zhu, zhumo@htu.edu.cn; Zongbo Qiu, qiuzongbo@126.com
†ORCID: Mo Zhu orcid.org/0000-0003-0884-1368