Endophytic fungi: versatile partners for pest biocontrol, growth promotion, and climate change resilience in plants
- 1School of Plant Sciences, College of Agriculture and Environmental Sciences, Haramaya University, Dire Dawa, Ethiopia
- 2International Centre of Insect Physiology and Ecology (ICIPE), Addis Ababa, Ethiopia
Plant-associated endophytic fungi (EFs) are emerging as a promising solution to advancing modern agriculture and fostering environmental sustainability, especially in the face of climate change scenarios. These fungi, either naturally residing in plants or introduced through artificial inoculation techniques, improve agricultural production due to their various roles in protecting and supporting host plants. The majority of EFs serve as natural biocontrol agents for a variety of agricultural pests, such as insects, phytopathogens, nematodes, and weeds. Notably, EFs produce secondary metabolites, trigger immune responses, modify plant defense gene expression, confer host plant resistance and/or tolerance, and regulate pest growth, populations, and survival to combat agricultural pests. Beyond controlling pests, EFs promote optimal plant growth, development, and resilience by aiding in the synthesis of vital compounds such as phytohormones and bioactive metabolites, nutrient acquisition, and fortifying plants against environmental stresses and climatic changes. Moreover, the mostly nonpathogenic nature of EFs, coupled with their high yield potential, environmental safety, and cost effectiveness, positions them as eco-friendly and economically viable alternatives to synthetic agrochemicals amidst rapid climate change scenarios. As a result, the promising horizon of EFs in agricultural production necessitates interdisciplinary study and microbial modulation approaches to optimize symbiotic plant-EF relationships and their potential for improved productivity. This review provides current and comprehensive insights into the practical applications and multifaceted benefits of EFs in pest management, plant growth promotion, and climate change resilience for future agricultural production improvements. The analysis reveals the potential of developing EFs into innovative bioformulations such as biofertilizers, biostimulants, and biopesticides, thereby paving the way for their integration into a sustainable and more resilient future agricultural system.
1 Introduction
Global food and nutrition security, in the face of rapid population growth and climate change, requires a sustainable food production approach addressing availability, access, utilization, and stability, ensuring livelihoods while preserving natural ecosystems and services (Giller et al., 2021). Agriculture, which is responsible for 90% of global food calories (Cassidy et al., 2013), will be at the forefront of transforming and/or reforming conventional practices by employing agroecological principles to address sustainability and environmental concerns within food systems. These concerns have led plant-associated microorganisms, particularly endophytic fungi (EFs), to emerge as a new frontier in agricultural production, achieving global demands for nutritious foods and eventually becoming fundamental to sustainable agriculture (Chitnis et al., 2020; Singh et al., 2021; Verma et al., 2022). In particular, EFs can reduce reliance on synthetic agrochemicals, which have a detrimental impact on agricultural productivity, ecosystems, and human health, thereby promoting environmentally friendly approaches for addressing food production challenges (Fadiji and Babalola, 2020; Rigobelo and Baron, 2021). Therefore, EFs are crucial for guaranteeing agricultural production and environmental sustainability.
The widespread application of EFs is revolutionizing the field of agriculture, primarily due to their remarkable capacity to support and protect host plants by producing beneficial bioactive molecules and acting as biocontrol agents to naturally regulate plant pest populations (Yan et al., 2019; Verma et al., 2022). These fungi colonize plants through root, stem, or leaf tissues and can occur naturally or be introduced into plants through seed coating, root dipping, and foliar spraying (Vega et al., 2008; Tefera and Vidal, 2009; Jain and Pundir, 2017). Most EFs are classified in the phyla Oomucota, Ascomycota, Chytridiomycota, and Zygomocata (Gul et al., 2014). Most of these important EFs are found in the orders Hypocreales (Ascomycetes) and Entomophthorales (Sharma et al., 2019). For instance, Beauveria, Metarhizium, Paecilomyces, Aschersonia, Hirsutella, and Lecanicillium are the most well-known fungal genera of these orders (Goettel, 2008). Mostly, they are formulated as Beauveria bassiana, Metarhizium anisopliae, and Trichoderma spp. propagules (Gul et al., 2014).
Endophytic functional groupings are diverse in ecological functions, host range, taxonomy, transmission, tissue specificity, and colonization patterns (Aamir et al., 2020). Based on ecological category/diversity/functional roles, EFs were grouped into two main categories: clavicipitaceous fungal endophytes (C-endophytes) and nonclavicipitaceous (NC-endophytes) fungal endophytes (Aremu et al., 2017). C-endophytes, also known as true endophytes, are host-specific and most common in the grass family Poaceae, which includes Balansia sp. and Epichloë sp., and often transmitted vertically through seeds, potentially resulting in an obligate association and higher rates of infection (Khiralla et al., 2016). These EFs are beneficial to the plant by improving plant growth and producing certain toxic chemicals against herbivory; however, they rely on the host plant species, environmental conditions, and genotype (Aremu et al., 2017). In contrast, NC endophytes are predominant and associated with both nonvascular and vascular plant species and are transmitted horizontally. These endophytes, including EFs such as Lecanicillium lecanii, Beauveria bassiana, Metarhizium anisopliae, and Isaria spp., can have impacts on several agricultural pests by antagonizing plant diseases and promoting plant growth (Jain and Pundir, 2017). However, until now, only a few species of EFs have been isolated, identified, characterized, and implemented in pest management.
Mutualistic endophytes, which are mostly fungal microbes that survive within plant tissues without adversely affecting the host, can promote plant growth and development while efficiently reducing agriculturally important pests, especially insects (Vega et al., 2008; Agbessenou et al., 2020), plant pathogens (Yan et al., 2019), and nematodes (Tolba et al., 2021). The mechanisms of EF colonization to suppress potential pests differ depending upon the species of EFs and the host plants to be colonized, and they can be employed in either direct or indirect ways (Quesada-Moraga et al., 2014; Zhang et al., 2019; Rajani et al., 2021). Furthermore, EFs are ubiquitous and have been demonstrated to improve host plant growth and tolerance against various abiotic stresses (Lata et al., 2018; Morsy et al., 2020). As a result, there is significant and developing interest in implementing them in agriculture as biocontrol agents of pests and plant growth and adaptation promoters (Molina-Montenegro et al., 2016; Mantzoukas and Eliopoulos, 2020). The use of EFs is also considered an alternative to synthetic insecticides (Sinno et al., 2020), eco-safety and lack of pathogenicity to plants (Mantzoukas and Eliopoulos, 2020), to reduce the impact of pesticides on living organisms and resistance development (Hernández-Rosas et al., 2020), less harmfulness to nontarget organisms, cost-effectiveness, high yield, and absence of harm to the environment (Mantzoukas and Eliopoulos, 2020). According to Yung et al. (2021), EFs are mostly nonpathogenic, with potential applications in organic matter mineralization, plant nutrition, biocontrol agents, crop productivity improvement, and sustainability while reducing chemical inputs, making them environmentally friendly tools that require integration and/or rotation with other alternatives. In addition, EFs also protect plants from different environmental stresses, such as drought, salinity and temperature (Lata et al., 2018; Morsy et al., 2020). However, their success depends on many factors, including their interactions, target host, and environment (Morales-Sánchez et al., 2020). The specific relationships between host plants and EFs, as well as the environment, could change throughout the plant’s lifecycle based on environmental and intrinsic factors such as leaf traits, host chemistry, and leaf chemical profiles (Sinno et al., 2020). In general, despite their numerous benefits, the utilization of EFs in developing countries by farmers is limited. Nevertheless, these EFs are not yet sufficiently produced and/or available to fulfill farmers’ demands even in developed countries. Hence, this review provides recent and comprehensive information on plant-fungal endophyte associations, the efficiency of EFs as pest biocontrol agents, growth promoters, and climate change resilience partners in plants.
2 Plant-fungal endophyte associations and related regulating factors
All plants appear to be symbiotic with fungal endophytes in natural ecosystems, which survive within plant tissues either throughout their lives or during a particular period of their lifespans without producing obvious damage and/or changing the morphology of their hosts. This makes the plant-fungal endophyte interaction a balanced antagonism where fungi survive by consuming nutrients from the plants, providing various benefits, while the host plants activate virulence mechanisms for colonization and host defenses (Baron et al., 2020). However, EFs inhabiting healthy plant tissues may convert from nonpathogenic to pathogenic modes when the plant is stressed (Galindo-Solís and Fernández, 2022). This diverse group of fungi can have a significant impact on plant families by conferring abiotic and biotic stress tolerance, boosting biomass while reducing water utilization, or decreasing fitness through resource allocation modifications (Khiralla et al., 2016). Through these positive relationships, plant growth, nutrition, and productivity can be improved (Behie and Bidochka, 2014) in various ways, such as providing nitrogen (N) to plants (Behie et al., 2015), acting as biocontrol agents (Akello and Sikora, 2012; Suryanarayanan, 2019; Huang et al., 2020), and improving tolerance to abiotic stress (Morsy et al., 2020). After successful colonization, EFs assist plants in producing plant hormones (phytohormones) and other bioactive compounds by promoting the production of secondary metabolites within plant tissues (Khan et al., 2019), enhancing tolerances to drought, salinity, and extreme temperature (Morsy et al., 2020), interfering with weed growth and germination and hindering the activities of insects, pathogens, and plant parasitic nematodes (Schouten, 2016; Sallam et al., 2021). What is interesting in a plant-fungal endophyte symbiotic relationship is that the plant itself helps fungal endophytes by offering shelter, nutrients, and seed spreading. In general, EFs are known to affect the biosynthesis of phytohormones, enzymes, and other bioactive compounds in plants (Satheesan and Sabu, 2020).
However, for EFs to be effective symbionts of different plants (pests), they need to be capable of infecting, occupying, and establishing themselves in both organisms (Branine et al., 2019). Colonization by EFs may be systemic (Quesada-Moraga et al., 2014), restricted to some plant tissues (Wearn et al., 2012), or distributed throughout the plant parts (Behie et al., 2015). Both gene duplication (Wang and St Leger, 2007) and horizontal gene transfer (Zhang et al., 2019) are involved in the penetration and establishment of EFs inside both host plants (pests). However, environmental conditions, inoculation methods, season of sample collection, species of fungal endophytes, geographical location, and genotype of the host plant itself can influence EF colonization of host plants (; Tefera and Vidal, 2009; Morales-Sánchez et al., 2020; Wu et al., 2020). Furthermore, depending on the method of inoculation (seed coating, foliar spray of conidia, conidial suspension injection, radicle dressing, soil drenching, and root and rhizome immersion), EFs can vary in their ability to colonize different plant parts (Tefera and Vidal, 2009). Wei Q. Y. et al. (2020) found that leaf spraying of conidial suspension on tomato plants resulted in greater colonization by B. bassiana than seed dressing. Leaf inoculation using B. bassiana conidial suspensions has also been described as the most efficient method of colonizing sorghum plants, successfully delivering the fungal endophyte into leaves (Tefera and Vidal, 2009).
Several factors related to geographic factors and the ecological function of the host plant also exert an influence on the diversity, specificity, and specialization of EF communities (Aamir et al., 2020). A report on native Hawaiian plants coexisting along an altitudinal gradient, for instance, revealed distinct patterns of EF diversity, host specificity, and interaction specialization across various elevations, with less specialization and greater diversity occurring at extreme altitudes (Cobian et al., 2019). This suggests that associations between host plants and EFs are more specialized under intermediate conditions and less specific under ecological extremes. A study by Wu et al. (2020) found that certain key chemical components and growth patterns in Dendrobium catenatum were influenced by the integration of cultivar genetics and species of fungal endophyte. He et al. (2019) studied the genetic variation in the forb host Oxytropis ochrocephala, its interaction with EF Alternaria oxytropis, and swainsonine mycotoxin production, revealing that host genotype and precipitation significantly influenced population-scale swainsonine production. Similarly, Hughes et al. (2020) observed significant impacts from the root EF Lulwoana sp. on phenotypic traits across genotypes of Spartina alterniflora, suggesting that plant-EF associations can exert community- and ecosystem-level impacts on plant species. A recent study also revealed that host plant genotype and tissue type have a significant influence on fungal endophytic communities in wheat, with the effects of host genetics mostly limited to leaves and roots (Latz et al., 2021). Hence, studying the factors driving plant-fungal endophyte associations is crucial for optimizing their development as agricultural solutions, with a focus on colonization and related mechanisms, as well as ecological effects on fungal endophyte community assembly and host interactions.
3 Efficiency of endophytic fungi in agricultural pest biocontrol
Agricultural pests, such as pathogens (e.g., fungi, bacteria, viruses, and nematodes), insect pests, and weeds, impede crop growth and yields, necessitating a comprehensive approach to addressing food production, as well as environmental conservation challenges. Management techniques that involve overreliance on synthetic agrochemicals have resulted in the resistance and resurgence of pests and negative environmental outcomes such as pollution (Waqas et al., 2015a; Verma et al., 2022). Alternative strategies such as biocontrol agents utilizing EFs are more effective, sustainable, and environmentally friendly approaches to plant protection (Ambele et al., 2020; Ahmad Y. et al., 2020; Mota et al., 2021). This section presents comprehensive analyses of the efficacy of endophytic fungi in the biocontrol of agricultural pests, including insect pests, plant diseases, parasitic nematodes, and weeds.
3.1 Endophytic fungi against insect pests
Biological control strategies utilizing beneficial organisms have increasingly attracted interest in recent decades as a means to reduce dependence on synthetic pesticides. Endophytic fungi in particular show promise due to their ability to form mutualistic relationships with host plants, alter metabolism, strengthen stress tolerance, and protect against insect pest damage (Aamir et al., 2020). The mechanisms through which EFs colonize and reduce insect herbivore damage vary based on the crops, fungal endophytes, application methods, and target insects. In insect pests, colonization (as a mode of action) of EFs begins with the bonding of fungi to the host plant surface, followed by penetration through the host cuticle and proliferation within the body cavity, which is the mode of entry for most EFs (Vega et al., 2008). During insect invasion, after efficient adherence, conidia develop to form hyphae, and conidial surface proteins, such as Metarhizium adhesin genes, MAD1 and MAD2 of M. anisopliae, and hydrophobin proteins (hyd1 and hyd2) of B. bassiana, are needed for plant and insect attachment and to recognize insect-specific compounds that then degrade the insect cuticles. For instance, Wang and St Leger (2007) reported that in Metarhizium robestsii, the adhesive MAD1 is essential for insect cuticles and MAD2 in plant conidial spore adhesions. Then, degradation of the insect cuticle/plant cell wall allows EF access to plant/insect tissues, which is accomplished by enzymatic activity such as that of various proteases and the mechanical pressure exerted by specific infections of hyphal structures (such as appressoria; Barelli et al., 2016). The EF needs to escape the insect immune system to efficiently parasitize insects and kill them. Once the EF pierces the insect cuticle, it distributes the insect’s hemolymph, where it divides into blastospores (yeast-like asexual spores), which collect nutrients in the hemocoel and generate particular insecticidal metabolites, leading to insect death (Fan et al., 2017). The mechanisms/modes of action by which EFs reduce insect herbivore injury in their host plants comprise antibiosis or pest avoidance/feeding deterrence (Vega et al., 2008), reduction of insect fitness, maximizing risks of predation and parasitism (Shymanovich and Faeth, 2018), reduction of insect survival (Lopez and Sword, 2015; Rasool et al., 2021), retardation of insect growth (Rondot and Reineke, 2019; Ahmad I. et al., 2020) and modification of plant defense gene expression (Ahmad I. et al., 2020). A possible mode of action could be direct defense or indirect plant defense by enhancing plant odors and attracting additional olfactory foraging natural enemies (Fuchs and Krauss, 2019).
Reductions in insect pest-induced plant damage were documented in a number of agricultural crop plants that were treated with EFs following effective colonization (Table 1). For instance, Tuta absoluta (Meyrick; Lepidoptera: Gelechiidae), which causes serious damage to tomato and nightshade (Solanum scabrum), was reduced when these plants were treated with Trichoderma asperellum, Beauveria bassiana (Balsamo) vullemin (Ascomycota: Hypocreales), and Hypocrea lixii (Agbessenou et al., 2020). In tomatoes, the use of B. bassiana was also effective in reducing the damage caused by Helicoverpa armigera (Toffa et al., 2021). A similar reduction in damage caused by bean stem maggot (Ophiomyia phaseoli) was reported in common bean (Phaseolus vulgaris) when M. anisopliae ICIPE 78 was used as a potential EF (Mutune et al., 2016). Similarly, B. bassiana colonizing tomato leaves also resulted in a reduction in the longevity of T. absoluta larvae and caused 50% mortality of all larval instars (Klieber and Reineke, 2016). Furthermore, EFs can be employed along with other insect pest management techniques, such as biological control agents acting as natural enemies (Akutse et al., 2013). Jaber and Araj (2018) reported that EFs such as B. bassiana and Metarhizium brunneum could be combined with the aphid endoparasitoid Aphidius colemani Viereck (Hymenoptera: Braconidae) for the control of the green peach aphid Myzus persicae Sulzer (Homoptera: Aphididae) in sweet pepper (Capsicum annum).
Several reports on insect pest damage reduction after the application of EFs to crops are also available (Quesada-Moraga et al., 2014; Muvea et al., 2015; Rondot and Reineke, 2019; Table 1). For example, the production of alkaloids by Epichloë species in diverse grass species accumulating in plants is toxic to numerous insect pests (Lugtenberg et al., 2016). Nodulisporic acid, produced by Nodulisporium sp., is another alkaloid molecule that is vital for preventing insect herbivory by activating glutamate, which results in the flow of chlorine ions through the chlorine channels of insect muscle and nerve cells, resulting in flaccid paralysis (Rigobelo and Baron, 2021). New insecticidal anthraquinone and chloramphenicol (Yuan et al., 2020) derivatives characterized from Acremonium vitellinum were reported to be effective against the cotton bollworm Helicoverpa armigera (Hübner; Lepidoptera: Noctuidae). In general, a wide range of secondary metabolites, such as polyketides exhibiting antibiotic activity, have been synthesized by fungal endophytes (Lugtenberg et al., 2016; Aamir et al., 2020). Therefore, insect pest management approaches could benefit from harnessing EFs given their low costs, high efficacy, safety for nontarget species and the environment, and potential to enrich agroecosystem biodiversity (Barelli et al., 2016).
3.2 Endophytic fungi against plant diseases and parasitic nematodes
Fungal endophytes can act as biocontrol agents by stimulating the plant’s inherent defense molecules and defending themselves or by producing bioactive compounds with the ability to kill or prevent pathogen attacks on their own (Jaber and Araj, 2018; Huang et al., 2020). These bioactive compounds have antifungal and antibacterial properties and include terpenoids, flavonoids, alkaloids, quinols, chlorinated compounds, peptides, steroids, polyketides, phenols, and other VOCs (Moraes et al., 2020; Lu et al., 2021). As a result, several crop plants treated with EFs showed reductions in plant damage from a variety of plant diseases and plant-parasitic nematodes following effective colonization (Tables 2, 3). In a recent study, Fusarium oxysporum f. sp. cucumerinum, which causes wilt in cucumber, was treated with 30 species of EFs, of which Penicillium sp. and Hypocrea sp. were reported to be effective at hindering the mycelial colony growth of diseases and successfully suppressing wilt severity in cucumber (Abro et al., 2019). Recent studies have indicated that endophytic Trichoderma has the capacity to reduce the incidence of red root rot disease caused by Ganoderma philippii in Acacia mangium seedlings (Gafur, 2023; Gafur et al., 2023). Sallam et al. (2021) also reported the antagonistic activity of T. longibrachiatum, T. asperellum, and T. atroviride against R. solania, and the three EF isolates produced pectinase and chitinase and solubilized phosphorus (P) in soybean plants. Similarly, M. brunneum and B. bassiana colonize wheat, leading to a reduction in disease incidence, severity, and development against F. culmorum, the causal agent of crown and root rot, following plant colonization (Jaber, 2018). Furthermore, Billar de Almeida et al. (2010) also reported the possibility of using EFs for the management of grapevine trunk diseases, which are very important and widespread fungal diseases impacting grapevines. Several other reports on plant–pathogen suppression following the treatment of crops with EFs are well documented (Hassanein et al., 2020; Zanudin et al., 2020; Aldinary et al., 2021; Mota et al., 2021; Table 2).
Much is unclear regarding the particular mode of action by which these fungi combat nematodes in most scenarios; however, they are most likely highly diverse. EFs can kill cells directly and impede cell development, obscure them when finding their host, immobilize and attack resources, repel nematodes, or use a combination of these modes of action (Schouten, 2016). For example, Swarnakumari and Kalaiarasan (2017) found that Purpureocillium lilacinum and Pochonia chlamydosporia can attach, penetrate, colonize, and finally condense egg contents in root-knot nematodes, Meloidogyne spp., and stop development at the gastrula stage. An additional example is the EF Acremonium implicatum from tomato root galls, which suppresses egg hatching and inhibits the development of root galls by the nematode M. incognita (Tian et al., 2014). Furthermore, an EF isolated from Cucumis melo root, which was characterized as P. brefeldianum, was also reported to be effective in reducing the severity and gall numbers caused by M. incognita (Miao et al., 2019). Apart from this, EFs can also produce different nematocidal secondary metabolites against different nematode species. For instance, chaetoglobosin A synthesized by Chaetomium globosum controls M. incognita (Khan et al., 2019), while the production of VOCs by Daldinia cf. concentrica affects the viability of M. javanica (Liarzi et al., 2016). As indicated in Table 3, several reports have revealed the positive impact of EFs on plant growth and their nematicidal effects on diverse species of nematodes (Yan et al., 2011; Liarzi et al., 2016; Bajaj et al., 2017; Zhou et al., 2018; Farhat et al., 2022).
The primary possible mechanisms of plant disease and plant-parasitic nematode suppression differ depending on the EF species. Several mechanisms through which these plant-EF associations benefit the host to shape resistance against various pathogens and nematodes have been suggested (Schouten, 2016; Swarnakumari and Kalaiarasan, 2017; Sallam et al., 2021). Mechanisms could occur through the direct inhibition of pathogens, such as competition for resources (space and nutrients), mycoparasitism and antibiosis (Rajani et al., 2021), and indirect inhibition by induced systemic resistance (ISR) and the induction of secondary metabolites (Rajani et al., 2021; Urooj et al., 2021). For instance, B. bassiana can endophytically inhabit a wide range of plant species and has been reported to diminish and limit the growth and development of various soilborne plant diseases in vitro, such as Pythium, Rhizoctonia, and Fusarium. Beauveria spp. are known to produce an array of bioactive metabolites, which in turn induce systemic resistance and limit the growth of fungal pathogens. Moreover, the defense responses of the EF Piriformospora indica, which causes sheath blight disease, against Rhizoctonia solani were able to disease the severity of the disease by limiting levels of hydrogen peroxide (H2O2) and increasing the activity of antioxidants such as superoxide dismutase (SOD) in rice crops (Nassimi and Taheri, 2017). Induced resistance by EF elicitors against early blight diseases caused by Alternaria solani in tomatoes was recently demonstrated by increasing certain enzymatic activities, such as lipoxygenase, peroxidase, polyphenol oxidase, and phenyl ammonia-lyase, when tomato seeds were treated with fungal colony-forming units (CFU) and different concentrations of crude oligosaccharide (CO) of EF; as a result, the vegetative and reproductive parameters of tomato were enhanced (Sujatha et al., 2021). Several research findings have reported that EFs can induce resistance in various crops against insects (Kiarie et al., 2020), nematodes (Swarnakumari and Kalaiarasan, 2017), phytopathogens (Muvea et al., 2015; Galletti et al., 2020; Urooj et al., 2021), and abiotic stresses (Lata et al., 2018; Morsy et al., 2020; Singh et al., 2021).
Some filamentous fungi, such as Trichoderma, act on plant-parasitic nematodes directly and indirectly through antibiosis, production of lytic enzymes, parasitism, paralysis, competition, improving nutrient and water absorption, modifying plant root morphology and/or rhizosphere interactions, and inducing resistance by initiating hormone-mediated responses, such as salicylic acid, jasmonic acid, and strigolactone, for plant defense (Poveda et al., 2020). As a direct mechanism, for instance, Acremonium implicatum antagonized the colonization of Meloidogyne incognita Chitwood nematodes in tomato (Solanum lycopersicum; Tian et al., 2014), and Penicillium brefeldianum antagonized the colonization of M. incognita nematodes in Cucumis melo (Miao et al., 2019). EFs can also produce different nematocidal secondary metabolites that can act indirectly against different nematode species. For instance, it was revealed that chaetoglobosin A formed by Chaetomium globosum acted against the root-knot nematode M. incognita (Khan et al., 2019), and volatile organic compounds (VOCs) released by Daldinia cf. concentrica acted against M. javanica in tomatoes (Liarzi et al., 2016).
3.3 Endophytic fungi as weed biocontrol agents
Weeds are among the undesired plants that grow alongside crops in the field, competing for nutrients and space, as well as hosting pathogens that attack crop plants and reduce production (Asim et al., 2022). Fungal endophytes have been shown to have bioherbicidal potential that suppresses and controls weeds while simultaneously stimulating crop plant growth, making them particularly suited for this use due to their dual benefits (Suryanarayanan, 2019; Ahmad Y. et al., 2020). Therefore, the use of EFs provides a more effective and environmentally sound approach to weed control than either synthetic herbicides, which pose risks to human and environmental health, or other biocontrol agents whose long-term efficacy relies upon persistence in introduced settings. The biocontrol of Orobanche using EFs such as Ulocladium spp. and Fusarium spp. resulted in 90% control in tomato and 90–97% control in watermelon, while other EFs, such as Rhizoctonia, Alternaria, and Sclerotinia, were also effective (Nemat Alla et al., 2008). Earlier studies have shown the effective biocontrol of Convolvulus arvensis, Chenopodium album, and Avena fatua through various EF species, with these weeds exhibiting substantial reductions in growth and germination when sprayed or inoculated with EF culture filtrates (Tunali et al., 2009; Akbar and Javaid, 2012).
Endophytic fungi exhibit multiple weed control mechanisms, impacting germination and subsequent growth stages and various physiological and biochemical processes. For example, absorbed fungal metabolites may damage seed cell membranes and disrupt vital processes such as amylase activity and cell division, delaying or preventing germination (Moura et al., 2020). Additionally, intercellular root colonization releases toxic substances, hindering germination and growth by suppressing photosynthesis and phytohormones while enhancing ROS and stress hormones such as ethylene and abscisic acid (Asim et al., 2022). For instance, Diaporthe phaseolorum, T. spirale, and P. simplicissimum have been shown to play crucial roles in reducing photosynthesis and growth in Ipomoea grandifolia and Senna occidentalis through different hydrolytic enzyme production (Moura et al., 2020). Daba et al. (2021) demonstrated that conidial suspensions of EFs exhibit varying herbicidal activities against weed germination and growth, with the herbicidal actions of applied conidia or exudates during fungal growth reducing germination and growth by up to 65%. Asim et al. (2022) also reported that F. oxysporum controlled A. fatua by reducing seed germination, vigor index, root length, shoot length, fresh weight, and dry weight by up to 95%, 100%, 64.21%, 62.5%, 73.68%, and 99%, respectively. Ahmad Y. et al. (2020) found that Alternaria spp. and Drechslera spp. reduced C. album germination by 57 and 75% and A. fatua germination by 44% and 31%, respectively. Bashir et al. (2018) also reported that secondary metabolites of Aspergillus niger significantly decreased the germination and shoot and root biomass of parthenium weeds by 90%, 57%, and 68%, respectively, when using original metabolites.
Endophytic fungi-secreted phytotoxic compounds such as holdysenterine and drechslerol-C, isolated from Drechslera spp. and cyclic tetrapeptide isolated from Alternaria spp. have reduced germination, growth, and chlorophyll content, leading to chlorosis, cell damage, and necrosis in C. album, A. fatua, C. arvensis, and Rumex dentatus (Akbar and Javaid, 2012; Ahmad Y. et al., 2020). Host-specific phytotoxic compounds called chenopodolans have been reported in the EF Phoma chenopodiicola for effective biocontrol of C. album (Ahmad Y. et al., 2020). These phytotoxic compounds usually cause the appearance of necrotic spots surrounded by chlorosis and cell damage on the leaves of target weeds and lack toxicity on nontarget cultivated and wild plants (Cimmino et al., 2015). Cyclic tetrapeptide and AAL toxins from Alternaria spp. also inhibited chlorophyll contents, caused cell damage and electrolyte leakage, and interfered with overall plant metabolism by producing reactive oxygen species, ultimately leading to chlorosis and cell death (Ahmad Y. et al., 2020). Studies have demonstrated that conidial suspensions of Aspergillus spp. have variable herbicidal activities against weed germination and growth, with applied conidia or exudates during fungal growth reducing germination and growth by up to 65% (Bashir et al., 2018; Daba et al., 2021).
Herbicidal compounds secreted by EFs have also been found to potentially compromise cell membrane integrity, resulting in increased permeability, solute leakage, and elevated electrolyte leakage, significantly impacting the overall health and functionality of the affected weeds. For instance, EF-produced phytotoxic compounds, such as bisanthraquinones, octahydronaphthalenes, alkaloids, and molecules possessing chemical scaffolds, are capable of absorbing electrons and affecting redox processes (Ahmad Y. et al., 2020; Moura et al., 2020). EF F. oxysporum also produces polyphenols that reduce A. fatua growth, including quercetagetin, isovitexin, calycosin, dihydroxy-dimethoxyisoflavone, naringenine, vitaxin, cis-cafatric acid, caffeoyl-D-glucose, and p-dyroxy benzoic acid (Asim et al., 2022). Some of these chemicals have also shown allelopathic and herbicidal activity against A. retroflexus, P. oleracea, C. album, and Abutilon theophrasti (Boselli et al., 2021). Cimmino et al. (2015) also found that nonproteic toxic amino acids from the culture of Ascochyta caulina cause cell harm and necrosis in the leaves of C. album, finally causing electrolyte leakage. Toxins such as indole 3-acetic acid (IAA) isolated from A. alternata were also found to damage cells, cause chlorosis and induce electrolyte leakage in various weed species, demonstrating their potential as natural herbicides (Ahmad Y. et al., 2020). Suryanarayanan (2019) reported that EFs produce metabolites that exhibit herbicidal activities and induce chlorosis, finally causing necrosis in Lemna minor. The herbicidal effects of various species of EFs on different weeds, as well as the compounds released by these EFs and their effects on the host weeds, are shown in Table 4.
4 Efficiency of endophytic fungi in plant growth promotion
4.1 Endophytic fungi as inducers of plant growth-promoting phytohormones
Fungal endophytes are microorganisms that enhance plant growth and development either directly through secreting or stimulating vast arrays of phytohormones (Bilal et al., 2018; Yan et al., 2019; Rajani et al., 2021). They can actively or passively regulate plant growth by enhancing the production of plant hormones such as auxin, gibberellic acid (GA), abscisins, ethylene, and IAA (Lata et al., 2018; White et al., 2019; Rigobelo and Baron, 2021). Due to their ability to produce enzymes and other bioactive compounds, EFs such as Penicillium, Piriformospora indica, Sebacina vermifera, and Colletotrichum have more potent plant growth-promoting abilities even in unfavorable environments (Kaur, 2020). A diverse range of phytohormones and phytohormone signaling pathways are utilized in mediating PGP, resulting in greater root growth and, ultimately, higher yield and biomass (Bilal et al., 2018; Abro et al., 2019; Fadiji and Babalola, 2020). Some common plant growth hormones produced by different fungal endophytes in different plants are presented in Table 5. Aamir et al. (2020) further delineated that the most common mechanisms by which EFs provide benefits to plants have been achieved by siderophore production, solubilization of phosphates, N fixation, phytohormones such as IAA production, and 1-aminocyclopropane-1-carboxylate (ACC) deaminase activity. Various mechanisms by which EFs benefit their host plants are presented in Figure 1.
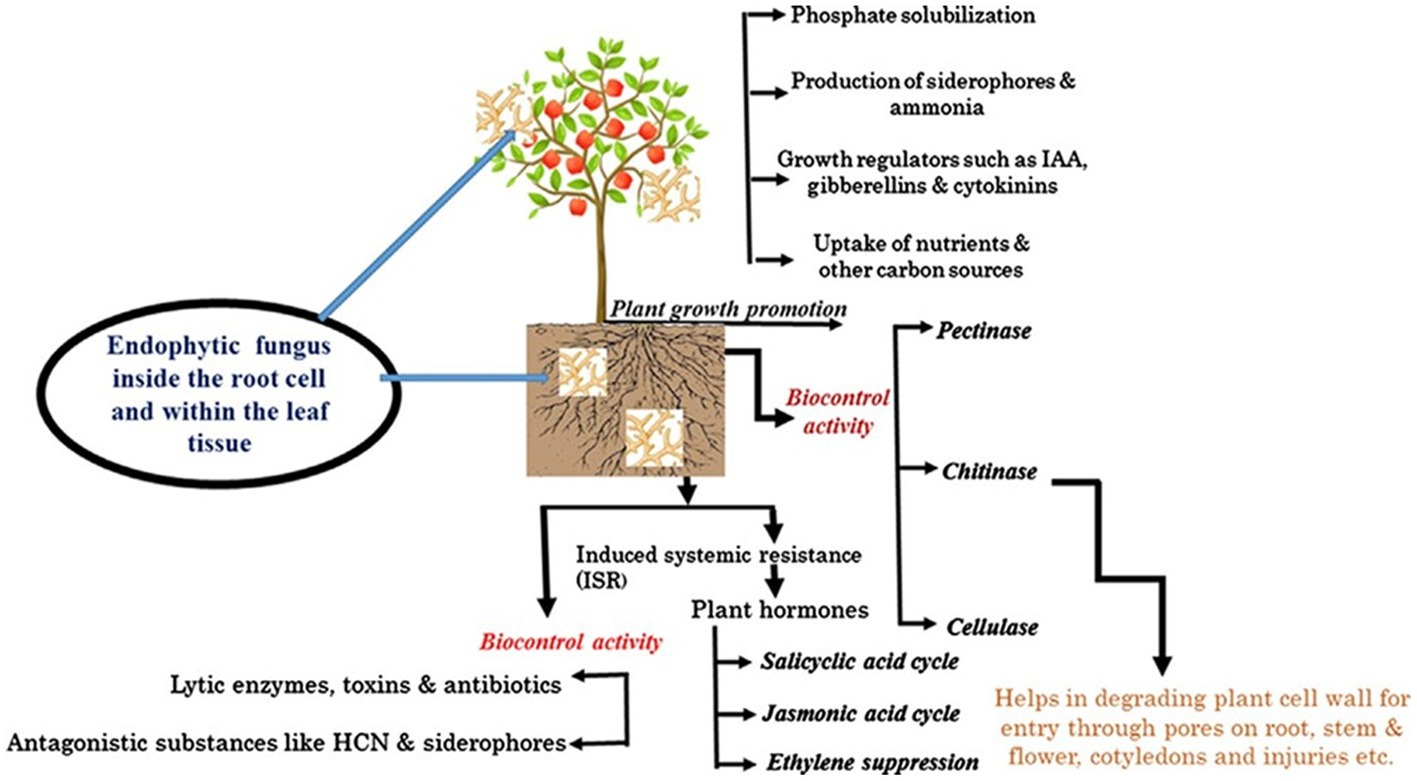
Figure 1. The functional dimension of EFs that localize inside living plant root tissues and within root cells and the mechanisms by which they benefit their host plants. Adapted from Aamir et al. (2020).
Auxins, gibberellins, and cytokinins synthesize EF and are known to serve as chemical mediators and signaling molecules for plant growth (Baron et al., 2020). Endophytic fungi, such as F. fujikuroi, Serendipita indica, and Piriformospora indica, can produce phytohormones such as indole-3-acetic acid (IAA), cytokinin, and gibberellic acid, suggesting that they can regulate host signaling and influence physiological and metabolic activities (Liu et al., 2021). However, PGP is primarily driven by IAA, which positively impacts shoot and root development, including responses to tropism, cell division, cell elongation, vascular tissue differentiation, and root formation (Airin et al., 2023). Khan et al. (2008) found that bioactive gibberellins such as GA1, GA3, GA4, and GA7 in P. citrinum IR-3-3, EF isolated from dune plants, improved seedling length and growth in Waito-c rice dwarf mutant and sandy plant Atriplex gmelinii. Bader et al. (2020) studied Trichoderma strains from soils of Argentine Pampas, revealing high phytohormone production capacities and significantly improved plant height, fresh and dry biomass, chlorophyll content, and higher surface area in inoculated tomato seeds after 45 days. Baron et al. (2020) found that P. lilacinum, P. lavendulum, and Metarhizium marquandii can produce IAA in soybean, bean, and maize, promoting growth by improving parameters such as dry matter, attributed to endophytic colonization of the plants. As a result, sustainable agriculture could rely largely on endophytic fungi, which possess the unique ability to thrive and colonize host plant tissues, promoting the production of various phytohormones with biological activities.
4.2 Endophytic fungi as modulators of nutrient acquisition by plants
Fungal endophytes can also boost plant growth indirectly by assisting the plant in acquiring nutrients (Bilal et al., 2018; Yan et al., 2019; Rajani et al., 2021). In previous studies, EFs that dwell in host plants without developing obvious symptoms have been noted to play an important role in boosting plant growth by aiding nutrient absorption (Molina-Montenegro et al., 2016; Abro et al., 2019; Huang et al., 2020). These microorganisms are capable of enhancing agricultural productivity by increasing plant access to nutrients [N, P, K, Zn, iron (Fe), etc.] producing plant hormones, reducing ethylene, or increasing the water acquisition rate (Baron et al., 2018; Abro et al., 2019; Huang et al., 2020). Various nutrient transporters and processes of translocation have been confirmed in different plants infected with EFs. For instance, the nutrient acquisition mechanism employed by EFs includes the release of nutrients from insects that are decayed by microbes (Behie and Bidochka, 2014; White et al., 2019). This has been confirmed by Behie and Bidochka (2014), who reported the EF transfer of N from insects by some EF species, namely, M. guizhouense, M. flavoviridae, M. robertsii, M. brunneum, M. acridum, Akanthomyces (=Lecanicillium) lecanii and B. bassiana, P. vulgaris (common bean), G. max (soybean), P. virgatum (switchgrass), and T. aestivum (wheat). In this regard, Rigobelo and Baron (2021) showed that some species of EF can kill the larvae of insects and colonize plants endophytically, facilitating the transfer of nutrients from insects to these plants.
Fungal endophytes also help in the biodegradation of the litter of their host plants and decomposition of organic components, comprising cellulose, lignin, and hemicelluloses, which enable nutrient cycling (Lata et al., 2018). As reported by Behie and Bidochka (2014), EFs such as Heteroconium chaetospira have been shown to transfer N gained from decayed OM in the soil to Brassica campestris roots and increase N uptake efficiency. Yang et al. (2014) also indicated that fungal endophyte Phomopsis liquidambari colonization increases N, P, S, Zn, and Mg availability and significantly increases plant root length, root number, height, number of buds, chlorophyll levels and biomass in rice. Lugtenberg et al. (2016) revealed that P. indica increased shoot dry weight and grain yield and appeared to trigger flowering earlier at low temperatures under the lowest nutrient input, therefore helping to decrease fertilizer inputs while maintaining reasonable yields.
In nutrient acquisition, a process known as the ‘rhizophagy cycle’ enables EFs to access nutrients in the soil and then transport them back to the plants, where they penetrate the root cells at the tips of the roots closest to the nutrient exudate zone, where the growing root epidermal cells possess thin cell walls (Lata et al., 2018; White et al., 2019; Rigobelo and Baron, 2021; Singh et al., 2021). Rhizophagy symbiosis is a mutualism that involves nutrition exchange between plants and their EF partners (White et al., 2018, 2019; Rigobelo and Baron, 2021), and this process has been hypothesized by White et al. (2018, 2019), as depicted in Figure 2. Nutrient mining by EFs in the rhizophagy cycle, according to Kumar et al. (2020), involves three major steps: (1) Plant roots exudate organic acids, such as malic, citric, and acetic acids, into the soil, which bind with metals found in the soil (Mg2+, Fe3+, Mn2+, Co2+, Cu2+, Co2+, Ni2+, etc.); (2) the EFs eventually possess transporters that attach to these organic acid-metal complexes and transfer them into the EF cells; and (3) finally, EFs return to plant roots and enter root cells, where they oxidatively take nitrogen and micronutrients, including Fe, Zn, and Mg, from microbes and eject them back into the soil from root hair tips to acquire more nutrients.
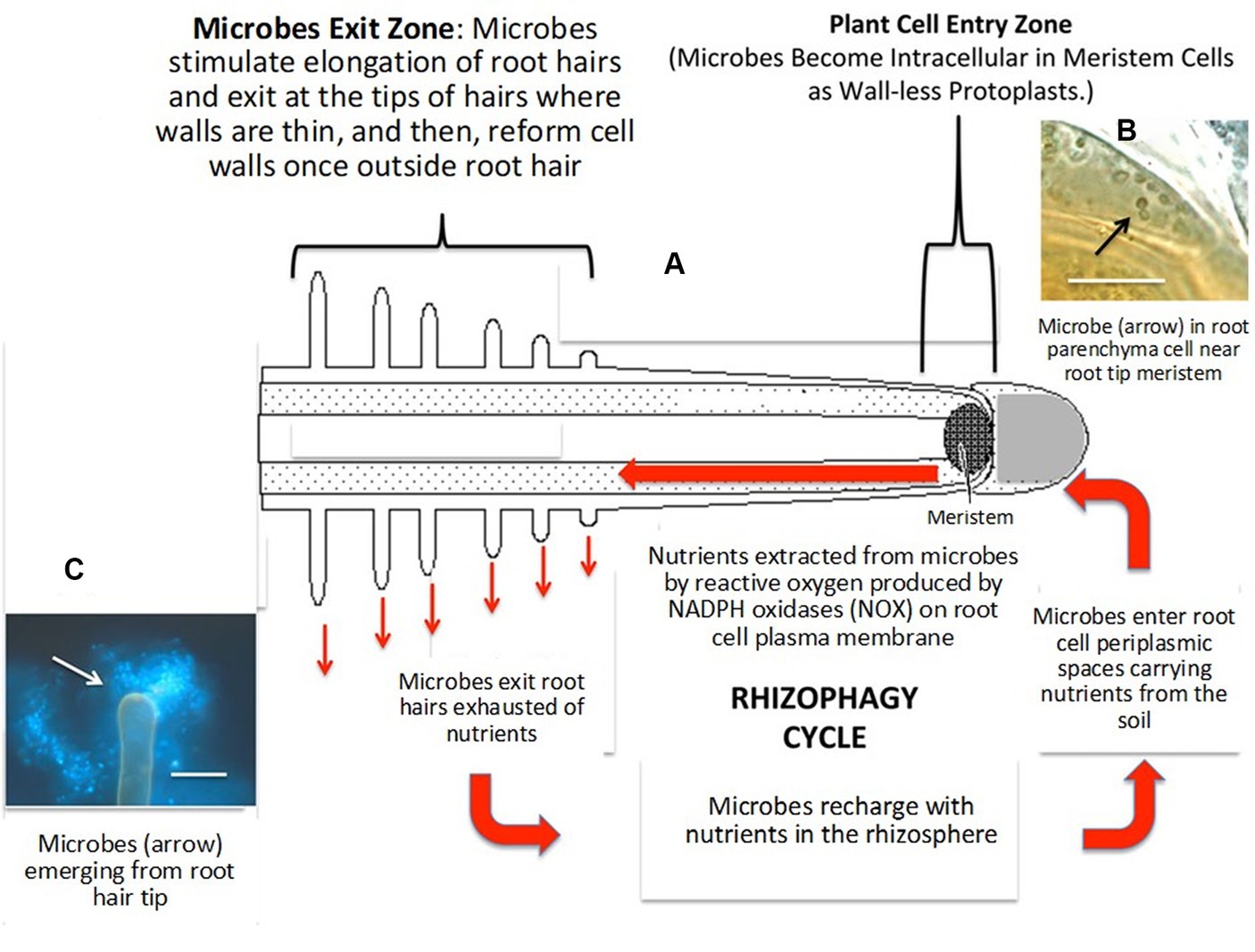
Figure 2. Diagram of the rhizophagy cycle showing microbes entering root cells at the plant root tip meristem and exiting root cells at the tips of elongating root hairs (A), microbes (arrow) in the periplasmic space of parenchyma cells near the plant root tip meristem (B) and microbes (arrow) emerging from the plant root hair tip (C). Adapted from White et al. (2019).
The rhizophagy cycle involves EFs transitioning between the EF/intracellular protoplast phase of the plant root cells and the free-living walled phase of soils, acquiring nutrients in the soil and oxidatively extracting them from the EF/intracellular protoplast phase (White et al., 2019; Kumar et al., 2020). In this cycle, EFs enhance root exudates and change their composition, which boosts microbial activity and nutrient mining. These root exudates contain proteins, vitamins, enzymes, phenolic acids, flavonoids, fatty acids, tannins, nucleotides, alkaloids, steroids, terpenoids, and polyacetylenes in addition to organic acids (White et al., 2018, 2019). Plants use their symbiotic EF to transport nutrients from the soils into the periplasmic spaces of their root cells, liberate nutrients via oxidation, and deposit used EF back into the rhizosphere by extending root hairs. This process has been found to improve nutrient transport into plants, assisting with nutrient acquisition from symbiotic EFs that sequester soil nutrients utilizing siderophores (White et al., 2018). Yung et al. (2021) further delineated that EFs act as the prey of roots in rhizophagy and serve to nourish plant nutrition and growth by improving the mineral nutrition and root elemental composition of host plants. For instance, Yung et al. (2021) reported that EFs, particularly DBF60 (Metapochonia rubescens), DBF79 (Alternaria thlaspis), DBF81 (Trichoderma harzianum), and DBF107 (Cladosporium sp.), isolated from various plant parts improved the root elemental contents of P, K, Mg, Ca, and S by up to five times in some cases.
Furthermore, EFs produce extracellular enzymes such as pectinase, cellulase, lipase, laccase, proteinase, phosphatase, and xylanase, which aid in the breakdown of macromolecules such as sugar-based polymers, lignin, organic phosphate, proteins, and carbohydrates to micromolecules accessible to plants (Kaur, 2020). In particular, EF chitinase enzymes play a vigorous role in the degradation and cycling of C and N from chitin molecules obtained from insect exoskeletons, crustacean shells, and fungal cell walls (White et al., 2019; Kaur, 2020). Chen et al. (2018) showed that Epichloë festucae var. lolii alleviated nutrient deficiency in ryegrass by promoting root metabolic activity and decreasing the stoichiometric ratios of C:N, C:P, and N:P in leaves and roots and the Cu content of roots. This EF also increased the concentration of C, N, P, K, Ca, Mg, Fe, and Mn, the dry weight of leaves and roots, the Cu content of leaves and root activity in the absence of fertilization. Overall, EFs have been confirmed to be a safe option for achieving sustainable farming due to their ability to increase the transfer of access to nutrients and other essential compounds (Lata et al., 2018; Fadiji and Babalola, 2020; Rigobelo and Baron, 2021; Singh et al., 2021).
5 Endophytic fungi conferring plant resilience under climate change conditions
Sustainable agricultural goals aim to address climate change as a significant impediment to food security for an increasing global population, emphasizing the need for climate-resilient production systems as a key dimension of sustainability. Climate change-driven abiotic stresses, such as climatic variability, drought, salinity, heat, osmotic stress, and nutrient shortages, are increasingly affecting and limiting agricultural productivity in this century (Ferus et al., 2019; Fontana et al., 2021). According to Singh et al. (2021), increasing drought, temperature, and soil salinity and decreasing water availability are growing challenges for improvements in crop production. Endophytic fungi, which inhabit almost all plants in natural Earth’s ecosystems, provide different benefits to boost crop performance, yield, and resilience to environmental stresses, hence mitigating the negative effects of climate change (Hereme et al., 2020; Verma et al., 2022). Consequently, the integration of EFs is perhaps a beneficial approach for both mitigating the effects of climate change on main crops and growing agricultural production on marginal lands. Thus, the use of EFs would be a promising approach for improving agricultural productivity by decreasing the reliance on harmful agrochemicals. For example, Tian et al. (2021) noted that after crossbreeding wild rice (African and Asian wild rice) species with grown rice accessions, wild rice contains more root EFs than the cultivated parent rice used to produce crosses in the first generations (F1 offspring), which opens up new research areas for the impacts of breeding on the inheritance of EFs in successive generations. Similarly, Abdelrazek et al. (2020) reported that carrot genotypes affected EF abundance and suggested the possibility of using EFs in carrot breeding programs for improvements in the health and yield of crops. Wang et al. (2020) also reported that Fusarium head blight (FHB) resistance gene Fhb7 (Fhb7) introgressions in wheat confer resistance to both FHB and crown rot in varied wheat backgrounds, offering a solution for Fusarium resistance breeding.
Several laboratory and greenhouse experiments have strongly indicated that endophytes could be used to mitigate stresses in agricultural crops to increase productivity (Chitnis et al., 2020; Singh et al., 2021), as demonstrated in Table 6. The colonization of EFs usually triggers physiological changes in plants, imparting tolerance to various abiotic (oxidative, drought, salinity, high temperature, high CO2, and metal toxicity) stresses (Lata et al., 2018; Morsy et al., 2020; Verma et al., 2022). EFs can enhance plant resilience to climate change by promoting the plant’s own molecule production and/or by producing several compounds on their own, which are crucial for adaptation to adverse environmental conditions (Lata et al., 2018; Morsy et al., 2020). In most cases, EFs aid host plants in responding to stresses by regulating plant growth and development using bioactive substances that can work together with hosts for better performance. They secrete phytohormones, saponins, triterpenoids, ginsenosides, proteolytic enzymes, growth hormones, phosphate-solubilizing factors, active volatile and nonvolatile metabolites, IAA, and ACC deaminase during stress to enhance plant growth and enable plants to overcome abiotic stresses (Moraes et al., 2020; Jagannath et al., 2021; Lu et al., 2021). According to Verma et al. (2022), T. atroviride, T. polysporum, and T. harzianum, which inhabit Phaseolus vulgaris, phosphate solubilizing factors, exude proteolytic enzymes, and active volatile and nonvolatile compounds that enhance plant growth and improve abiotic stress tolerance. Secondary metabolites such as flavonoids, phenylpropanoids, phytoalexins, and carotenoids are found in stressed plants inoculated with EFs, and they help plants tolerate abiotic stress by acting as antioxidants that scavenge ROS (Kaur, 2020; Jagannath et al., 2021). In a recent study, EFs increased the phenol and proline contents in drought-salinity-exposed plants, where phenols signal symbioses, while proline, a stress-associated amino acid, improves drought tolerance by regulating macromolecule stabilization and redox homeostasis (Ballesteros et al., 2023).
Fungal endophytes also indirectly promote plant growth by inducing resistance and promoting antibiotic, secondary metabolite, and siderophore production, which protects plants against abiotic stressors (Singh et al., 2021). Thus, root EF can be leveraged as a biotechnological tool to sustain high ecophysiology and productivity in abiotic stressors (Molina-Montenegro et al., 2016; Ferus et al., 2019). In areas under drought conditions, for instance, EFs offer benefits that are related to reduced lipid peroxidation, higher proline levels, and ion homeostasis upregulation or downregulation (Molina-Montenegro et al., 2016; Morsy et al., 2020). Studies have revealed that EFS confers drought tolerance in crops through enhanced photosynthesis, improved water use efficiency (Dastogeer, 2018; Hereme et al., 2020), improved nutrition and root development (Dastogeer, 2018), and induced stress-responsive gene expression (Molina-Montenegro et al., 2016; Hereme et al., 2020). For instance, under water stress conditions, EFs increased chlorophyll content, total biomass, net photosynthesis, relative water contents, and stomatal conductance in plants compared to nonstressed conditions (Dastogeer, 2018; Ismail et al., 2020). Additionally, EFs also aid plant adaptation to drought endurance by secreting phytohormones, exopolysaccharides, ROS, 1-aminocyclopropane-1-carboxylate deaminase, and volatile compounds while altering root morphology and biosynthesizing anti-stress metabolites (Fontana et al., 2021). Ismail et al. (2020) isolated the EF A. violaceofuscus from the fern Dryopteris flix L. and found that its culture filtrate had a higher concentration of secondary metabolites that improved plant height, chlorophyll contents, soybean seedlings, and sunflower biomass under drought and heat stresses. Drought and heat stresses can also be mediated by EFs through mitogen-activated protein kinase (MAPK) and heat shock proteins (HSPs), respectively (Lata et al., 2018).
Fungal endophytes also have the potential to mitigate soil salinity stresses in various crops (Badawy et al., 2021; Moghaddam et al., 2021). Some of the strategies mediated by EFs to mitigate salt (other abiotic) stresses include (1) proline accumulation within cells (Badawy et al., 2021; Gupta et al., 2021); (2) modulation of plant hormones/phytohormones (Gul et al., 2014; Baron et al., 2020; Illescas et al., 2021); (3) maintenance of ionic homeostasis by modulating ion accumulation, ensuring a low cytosolic Na+:K+ ratio and advancing nutrient uptake in plants (Gupta et al., 2021); (4) accumulation of glycine betaine and polyols (Khan et al., 2011); and (5) production of extracellular enzymes (Jagannath et al., 2021). For instance, Induratia spp. isolated from coffee plants also produce remarkable amounts of extracellular enzymes, such as protease, cellulase, lipase, phosphates and amylase (Monteiro et al., 2020). A recent study examined 203 EFs from 29 species from Baliospermum montanum tissues for extracellular enzymes and showed that 83% of isolates produced amylase, 79% cellulase, 77% phosphatase, 72% protease, and 59% lipase (Jagannath et al., 2021).
Furthermore, EFs can support host plants in responding to various stresses through the involvement and expression of mutualistic genes (Monteiro et al., 2020; Jagannath et al., 2021; Lu et al., 2021). In particular, EFs can upregulate genes involved in secondary metabolite production, osmotic regulation, ion transport, stress signaling pathways, and the synthesis of antistress metabolites and scavenger molecules (Lata et al., 2018; Chitnis et al., 2020; Harman et al., 2021). For instance, Verma et al. (2022) reported that wheat EF altered stress-related gene expression to enhance drought tolerance, while rice EF modified antioxidant defense gene expression to improve oxidative stress tolerance. Several transcriptomic and metabolomic studies have also indicated the involvement of mutualistic gene products in various plant growth and abiotic stressor adaptation pathways. A recent study by Toppo et al. (2023) revealed that T. longibrachiatum T6 upregulated the expression of actin, POD, SOD, and CAT biosynthetic genes to reduce salt stress in wheat seedlings, while T. harzianum upregulated CsAPX and CsGR genes in cucumber seedlings, mitigating various abiotic stresses. Abdelaziz et al. (2017) also showed that EF can help salt-stressed plants from osmotic stress by regulating the expression of the P5CS gene (pyroline-5-carboxylate synthase), which is involved in the biosynthesis of proline, starch-degrading enzyme activation, and glucan-water dikinase. Toppo et al. (2023) further observed that P. funiculosum LHL06 upregulates the GA1, GA3, GA4, GA7, and GA9 genes to reduce metal toxicity by modulating hormonal concentration while downregulating the stress mitigation-related genes G6PDH, GmGST3, GmGST8 and GmSOD1[Cu–Zn]. Fungal endophytes such as Paecilomyces formosus LHL10 and P. funiculosum LHL06 have also shown increased transcript expression of the GmHsp90A2 and GmHsp90A1 genes to enhance soybean growth under high temperature and drought stress (Bilal et al., 2020). In addition, MAPK genes are also key players in the MAPK pathway, which is responsible for producing ROS, signaling pathways, and the synthesis of antioxidants in EFs to help their host plants cope with abiotic stresses (Ogbe et al., 2020). In general, EFs demonstrate remarkable promise as a means to safeguard global agriculture and food security amidst climate change through their multifaceted abilities to promote plant growth and strengthen host plant resilience against environmental stresses.
6 Research gaps and future perspectives
Increasing threats to global food security from climate change, population growth, and multiple stress factors emphasize the urgent need to boost crop yields in vulnerable environments. Fungal endophytes show promise through direct and indirect mechanisms that promote plant growth, pest protection, and climate adaptation, offering a cost-effective and environmentally friendly alternative to improving food production and promoting sustainable agriculture practices. However, their agricultural application remains limited due to regulatory standards and difficulties with mass production, inoculant efficacy, and resistance from consumers and farmers (Khiralla et al., 2016; Ballesteros et al., 2023). Secondary metabolite production from EFs is effective at small-scale setts, but large-scale production has been unsuccessful due to poor yields and performance, particularly for functional polysaccharide biosynthesis. A deeper understanding of EF-produced metabolite structures, microbial interactions, and plant support and/or protective mechanisms could aid integrated management approaches, thereby improving production and benefiting the environment. Further research is also needed on EF manipulation of host morphology and physiology to assess the application potential. Fundamentally, elucidating complex relationships between EFs, crops, soil variables, and soil/plant microbiomes and how these influence morphological, physiological and biochemical responses is essential to optimize EF-mediated adaptations and strengthen sustainable agriculture amid climate threats.
There is also a knowledge gap about how biotic and abiotic stressors may alter plant-EF interactions, potentially leading to mutualistic or parasitic partnerships (Kamran et al., 2021), warranting comprehensive analysis of influential factors to determine the possible interactions. The competition between native microbiomes and introduced EFs, particularly in extreme environments, may lead to decreased effectiveness or negative impacts (Aremu et al., 2017), necessitating studies to confirm effects and identify potential shifts in EF efficacy. Comprehensive metabolomics, genomics, proteomics, and transcriptomics analyses could better elucidate these complex interactions, as well as the diversity and beneficial traits of EFs (Kamran et al., 2021; Verma et al., 2022). Furthermore, EF bioactivity, source, kind, and inoculant production need to be considered when determining the optimal quantity of EFs for plant growth, health, and climate change resilience. Overall, the ultimate goal should be translating endophytes into bioinoculants, biofertilizers and other products using interdisciplinary research and microbial modulation. Despite these aims, smallholder farms remain crucial for global food security, accounting for 50%–70% of global food production (Giller et al., 2021); hence, future efforts must integrate beneficial microbes such as EFs into smallholder agriculture to ensure urgent agricultural transformation.
7 Conclusion
Endophytic fungi are promising tools that can be used as biocontrol agents to regulate plant pest populations, enhance plant growth, and promote plant resilience against the challenges imposed by climate change. These microbes are greatly effective against agriculturally important pests, including insects, plant diseases, nematodes and weeds, while simultaneously having the potential to promote host plant growth and tolerance to various biotic and abiotic stresses. Thus, EFs have enormous potential to be used as effective and eco-friendly replacements for synthetic fertilizers and pesticides in agriculture under rapid climate change scenarios. Overall, EFs can be considered a key device in managing biotic and abiotic stresses due to the production of biologically active substances against both stresses by activating valuable products for agricultural production under rapid climate change scenarios. Generally, species-specific identification of EFs and characterization of their signaling, promotion and stress mitigation mechanisms, development and shelf-life enhancement components as climate-smart biocontrol agents and biofertilizers are highly imperative in agriculture under rapid climate change scenarios.
Author contributions
TF: Conceptualization, Investigation, Methodology, Resources, Supervision, Validation, Visualization, Writing – original draft, Writing – review & editing. EK: Conceptualization, Investigation, Methodology, Resources, Supervision, Validation, Visualization, Writing – original draft, Writing – review & editing. TT: Conceptualization, Resources, Supervision, Writing – original draft, Writing – review & editing. ZB: Conceptualization, Resources, Supervision, Writing – original draft, Writing – review & editing.
Funding
The author(s) declare that no financial support was received for the research, authorship, and/or publication of this article.
Acknowledgments
The authors gratefully acknowledge the financial support for this paper by the Biovision Foundation for Ecological Development (DPP016-push pull scale-up). We acknowledge the Swedish International Development Cooperation Agency (Sida), the Swiss Agency for Development and Cooperation (SDC), the Federal Democratic Republic of Ethiopia, and the Government of the Republic of Kenya.
Conflict of interest
The authors declare that the research was conducted in the absence of any commercial or financial relationships that could be construed as a potential conflict of interest.
Publisher’s note
All claims expressed in this article are solely those of the authors and do not necessarily represent those of their affiliated organizations, or those of the publisher, the editors and the reviewers. Any product that may be evaluated in this article, or claim that may be made by its manufacturer, is not guaranteed or endorsed by the publisher.
Author disclaimer
The views expressed herein do not necessarily reflect the official opinion of the donors.
References
Aamir, M., Rai, K. K., Zehra, A., Kumar, S., Yadav, M., Shukla, V., et al. (2020). “Fungal endophytes: classification, diversity, ecological role, and their relevance in sustainable agriculture” in Microbial endophytes. eds. A. Kumar and V. K. Singh (Cambridge, UK: Woodhead Publishing), 291–323.
Abdelaziz, M. E., Kim, D., Ali, S., Fedoroff, N. V., and Al-Babili, S. (2017). The endophytic fungus Piriformospora indica enhances Arabidopsis thaliana growth and modulates Na+/K+ homeostasis under salt stress conditions. Plant Sci. 263, 107–115. doi: 10.1016/j.plantsci.2017.07.006
Abdelrazek, S., Simon, P., Colley, M., Mengiste, T., and Hoagland, L. (2020). Crop management system and carrot genotype affect endophyte composition and Alternaria dauci suppression. PloS One 15:e0233783. doi: 10.1371/journal.pone.0233783
Abro, M. A., Sun, X., Li, X., Jatoi, G. H., and Guo, L. D. (2019). Biocontrol potential of fungal endophytes against fusarium oxysporum f. sp. cucumerinum causing wilt in cucumber. Plant Pathol. J. 35, 598–608. doi: 10.5423/ppj.Oa.05.2019.0129
Affokpon, A., Djihinto, A. C., Coffi, E. N. D., Coyne, D. L., and Coosemans, J. (2018). Root endophytic status of west African biocontrol agents and implications for root-knot nematode management. Nematropica. 48, 92–100. Available at: https://journals.flvc.org/nematropica/article/view/106936
Agbessenou, A., Akutse, K. S., Yusuf, A. A., Ekesi, S., Subramanian, S., and Khamis, F. M. (2020). Endophytic fungi protect tomato and nightshade plants against Tuta absoluta (Lepidoptera: Gelechiidae) through a hidden friendship and cryptic battle. Sci. Rep. 10:22195. doi: 10.1038/s41598-020-78898-8
Ahmad, Y., Ahmad, M. N., Zia, A., Alam, S. S., Khan, R. A. A., and Riaz, M. (2020). Biocontrol of economically important weed species through endophytic fungi isolated from Parthenium hysterophorus (family: Asteraceae). Egypt J Biol Pest Control 30:138. doi: 10.1186/s41938-020-00339-5
Ahmad, I., Del Mar, J.-G. M., Luthe, D. S., Shakeel, S. N., and Barbercheck, M. E. (2020). Endophytic Metarhizium robertsii promotes maize growth, suppresses insect growth, and alters plant defense gene expression. Biol. Control 144:104167. doi: 10.1016/j.biocontrol.2019.104167
Airin, A. A., Arafat, M. I., Begum, R. A., Islam, M. R., and Seraj, Z. I. (2023). Plant growth-promoting endophytic fungi of the wild halophytic rice Oryza coarctata. Ann. Microbiol. 73:36 (2023). doi: 10.1186/s13213-023-01738-3
Akbar, M., and Javaid, A. (2010). Management of some problematic weeds of wheat by metabolites of Drechslera sp. prepared in malt extract medium. Pak. J. Weed Sci. Res. 16, 145–151. doi: 10.28941/pjwsr.v16i2.307
Akbar, M., and Javaid, A. (2012). Herbicidal activity of fungal culture filtrates against Chenopodium album l. and Avena fatua L. J Anim Plant Sci 22, 977–982. Available at: https://thejaps.org.pk/docs/V-22-4/26.pdf
Akbar, M., Khalil, T., Andolfi, A., and Javaid, A. (2020). Isolation and identification of natural herbicidal compound from a plant pathogenic fungus, Drechslera biseptata. Pak. J. Bot. 52, 2245–2249. doi: 10.30848/PJB2020-6(43)
Akello, J., Dubois, T., Coyne, D., Gold, C. S., and Kyamanywa, S. (2007). “Colonization and persistence of the entomopathogenic fungus, Beauveria bassiana, in tissue culture of banana” in African crop science conference proceedings. eds. Z. A. Kasem, M. M. Addel-Hakim, S. I. Shalabi, A. El-Morsi, and A. M. I. Hamady (El-Minia, Egypt: Quick Color Print), 857–861.
Akello, J., and Sikora, R. (2012). Systemic acropedal influence of endophyte seed treatment on Acyrthosiphon pisum and Aphis fabae offspring development and reproductive fitness. Biol. Control 61, 215–221. doi: 10.1016/j.biocontrol.2012.02.007
Akutse, K. S., Maniania, N. K., Fiaboe, K. K. M., Van Den Berg, J., and Ekesi, S. (2013). Endophytic colonization of Vicia faba and Phaseolus vulgaris (Fabaceae) by fungal pathogens and their effects on the life-history parameters of Liriomyza huidobrensis (Diptera: Agromyzidae). Fungal Ecol. 6, 293–301. doi: 10.1016/j.funeco.2013.01.003
Aldinary, A. M., Abdelaziz, A. M., Farrag, A. A., and Attia, M. S. (2021). Withdrawn: biocontrol of tomato fusarium wilt disease by a new Moringa endophytic aspergillus isolates. Mater Today Proc. doi: 10.1016/j.matpr.2021.03.423
Ambele, C. F., Ekesi, S., Bisseleua, H. D., Babalola, O. O., Khamis, F. M., Djuideu, C. T., et al. (2020). Entomopathogenic fungi as endophytes for biological control of subterranean termite pests attacking cocoa seedlings. J. Fungi. 6:126. doi: 10.3390/jof6030126
Aremu, B. R., Alori, E. T., Kutu, R. F., and Babalola, O. O. (2017). “Potentials of microbial inoculants in soil productivity: an outlook on African legumes” in Microorganisms for green revolution: volume 1: microbes for sustainable crop production. eds. D. G. Panpatte, Y. K. Jhala, R. V. Vyas, and H. N. Shelat (Singapore: Springer), 53–75.
Asim, S., Hussain, A., Murad, W., Hamayun, M., Iqbal, A., Rehman, H., et al. (2022). Endophytic fusarium oxysporum GW controlling weed and an effective biostimulant for wheat growth. Front. Plant Sci. 13:922343. doi: 10.3389/fpls.2022.922343
Atugala, D. M., and Deshappriya, N. (2015). Effect of endophytic fungi on plant growth and blast disease incidence of two traditional rice varieties. J.Natn.Sci.Foundation Sri Lank 43, 173–187. doi: 10.4038/jnsfsr.v43i2.7945
Aybeke, M. (2017). Fusarium infection causes genotoxic disorders and antioxidant-based damages in Orobanche spp. Microbiol. Res. 201, 46–51. doi: 10.1016/j.micres.2017.05.001
Aybeke, M. (2020). Aspergillus alliaceus infection fatally shifts Orobanche hormones and phenolic metabolism. Braz. J. Microbiol. 51, 883–892. doi: 10.1007/s42770-020-00283-4
Aybeke, M., Şen, B., and Ökten, S. (2014). Aspergillus alliaceus, a new potential biological control of the root parasitic weed Orobanche. J. Basic Microbiol. 54, S93–S101. doi: 10.1002/jobm.201300080
Badawy, A. A., Alotaibi, M. O., Abdelaziz, A. M., Osman, M. S., Khalil, A. M. A., Saleh, A. M., et al. (2021). Enhancement of seawater stress tolerance in barley by the endophytic fungus aspergillus ochraceus. Meta 11:428. doi: 10.3390/metabo11070428
Bader, A. N., Salerno, G. L., Covacevich, F., and Consolo, V. F. (2020). Native Trichoderma harzianum strains from Argentina produce indole-3 acetic acid and phosphorus solubilization, promote growth and control wilt disease on tomato (Solanum lycopersicum L.). J King Saud Univer Sci 32, 867–873. doi: 10.1016/j.jksus.2019.04.002
Bajaj, R., Chen, S., Hu, W., Huang, Y., Prasad, R., Kumar, V., et al. (2017). “Protocol for biocontrol of soybean cyst nematode with root endophytic fungi” in Modern tools and techniques to understand microbes. eds. A. Varma and A. K. Sharma (Cham: Springer International Publishing), 401–412.
Ballesteros, G. I., Newsham, K. K., Acuña-Rodríguez, I. S., Atala, C., Torres-Díaz, C., and Molina-Montenegro, M. A. (2023). Extreme environments as sources of fungal endophytes mitigating climate change impacts on crops in Mediterranean-type ecosystems. Plants People Planet. doi: 10.1002/ppp3.10415
Baltruschat, H., Fodor, J., Harrach, B. D., Niemczyk, E., Barna, B., Gullner, G., et al. (2008). Salt tolerance of barley induced by the root endophyte Piriformospora indica is associated with a strong increase in antioxidants. New Phytol. 180, 501–510. doi: 10.1111/j.1469-8137.2008.02583.x
Barelli, L., Moonjely, S., Behie, S. W., and Bidochka, M. J. (2016). Fungi with multifunctional lifestyles: endophytic insect pathogenic fungi. Plant Mol. Biol. 90, 657–664. doi: 10.1007/s11103-015-0413-z
Baron, N. C., Costa, N. T. A., Mochi, D. A., and Rigobelo, E. C. (2018). First report of aspergillus sydowii and aspergillus brasiliensis as phosphorus solubilizers in maize. Ann. Microbiol. 68, 863–870. doi: 10.1007/s13213-018-1392-5
Baron, N. C., De Souza, P. A., and Rigobelo, E. C. (2020). Purpureocillium lilacinum and Metarhizium marquandii as plant growth-promoting fungi. PeerJ 8:e9005. doi: 10.7717/peerj.9005
Barra-Bucarei, L., González, M. G., Iglesias, A. F., Aguayo, G. S., Peñalosa, M. G., and Vera, P. V. (2020). Beauveria bassiana multifunction as an endophyte: growth promotion and biologic control of Trialeurodes vaporariorum, (Westwood) (Hemiptera: Aleyrodidae) in tomato. Insects 11:591. doi: 10.3390/insects11090591
Bashir, U., Khan, A., and Javaid, A. (2018). Herbicidal activity of aspergillus Niger metabolites against parthenium weed. Planta Daninha 36:10025. doi: 10.1590/S0100-83582018360100025
Behie, S. W., and Bidochka, M. J. (2014). Ubiquity of insect-derived nitrogen transfer to plants by endophytic insect-pathogenic fungi: an additional branch of the soil nitrogen cycle. Appl. Environ. Microbiol. 80, 1553–1560. doi: 10.1128/aem.03338-13
Behie, S. W., Jones, S. J., and Bidochka, M. J. (2015). Plant tissue localization of the endophytic insect pathogenic fungi Metarhizium and Beauveria. Fungal Ecol. 13, 112–119. doi: 10.1016/j.funeco.2014.08.001
Bilal, L., Asaf, S., Hamayun, M., Gul, H., Iqbal, A., Ullah, I., et al. (2018). Plant growth promoting endophytic fungi Asprgillus fumigatus TS1 and fusarium proliferatum BRL1 produce gibberellins and regulates plant endogenous hormones. Symbiosis 76, 117–127. doi: 10.1007/s13199-018-0545-4
Bilal, S., Shahzad, R., Imran, M., Jan, R., Kim, K. M., and Lee, I. J. (2020). Synergistic association of endophytic fungi enhances Glycine max L. resilience to combined abiotic stresses: heavy metals, high temperature and drought stress. Ind. Crop Prod. 143:111931. doi: 10.1016/j.indcrop.2019.111931
Billar de Almeida, A., Concas, J., Campos, M. D., Materatski, P., Varanda, C., Patanita, M., et al. (2010). Endophytic fungi as potential biological control agents against grapevine trunk diseases in Alentejo region. Biology 9:420. doi: 10.3390/biology9120420
Biswas, C., Dey, P., Satpathy, S., Satya, P., and Mahapatra, B. S. (2013). Endophytic colonization of white jute (Corchorus capsularis) plants by different Beauveria bassiana strains for managing stem weevil (Apion corchori). Phytoparasitica. 41, 17–21. doi: 10.1007/s12600-012-0257-x
Boari, A., and Vurro, M. (2004). Evaluation of fusarium spp. and other fungi as biological control agents of broomrape (Orobanche ramosa). Biol. Control 30, 212–219. doi: 10.1016/j.biocontrol.2003.12.003
Bogner, C. W., Kariuki, G. M., Elashry, A., Sichtermann, G., Buch, A. K., Mishra, B., et al. (2016). Fungal root endophytes of tomato from Kenya and their nematode biocontrol potential. Mycol Progress 15, 1–7. doi: 10.1007/s11557-016-1169-9
Boselli, R., Anders, N., Fiorini, A., Ganimede, C., Faccini, N., Marocco, A., et al. (2021). Improving weed control in sustainable agro-ecosystems: role of cultivar and termination timing of rye cover crop. Italian J. Agron. 16:1807. doi: 10.4081/ija.2021.1807
Branine, M., Bazzicalupo, A., and Branco, S. (2019). Biology and applications of endophytic insect-pathogenic fungi. PLoS Pathog. 15:e1007831. doi: 10.1371/journal.ppat.1007831
Cassidy, E. S., West, P. C., Gerber, J. S., and Foley, J. A. (2013). Redefining agricultural yields: from tonnes to people nourished per hectare. Environ. Res. Lett. 8:034015. doi: 10.1088/1748-9326/8/3/034015
Chen, D., Zhang, P., Liu, T., Wang, X. F., Li, Z. X., Li, W., et al. (2018). Insecticidal activities of chloramphenicol derivatives isolated from a marine alga-derived endophytic fungus, Acremonium vitellinum, against the cotton bollworm, Helicoverpa armigera (Hübner) (Lepidoptera: Noctuidae). Molecules 23:2995. doi: 10.3390/molecules23112995
Cherry, A. J., Banito, A., Djegui, D., and Lomer, C. (2004). Suppression of the stem-borer Sesamia calamistis (Lepidoptera; Noctuidae) in maize followingseed dressing, topical application and stem injection with African isolates of Beauveria bassiana. Int. J. Pest Manag. 50, 67–73. doi: 10.1080/09670870310001637426
Chhipa, H., and Deshmukh, S. K. (2019). Diversity of endophytic Fungi and their role in artificial agarwood production in Aquilaria tree. Adv Front Mycol Mycotechnol Basic Appl Aspects Fungi, 479–494. doi: 10.1007/978-981-13-9349-5_19
Chitnis, V. R., Suryanarayanan, T. S., Nataraja, K. N., Prasad, S. R., Oelmüller, R., and Shaanker, R. U. (2020). Fungal endophyte-mediated crop improvement: the way ahead. Front. Plant Sci. 11:561007. doi: 10.3389/fpls.2020.561007
Cimmino, A., Masi, M., Evidente, M., Superchi, S., and Evidente, A. (2015). Fungal phytotoxins with potential herbicidal activity: chemical and biological characterization. Nat. Prod. Rep. 32, 1629–1653. doi: 10.1039/c5np00081e
Clement, S. L., Elberson, L. R., Bosque-Pérez, N. A., and Schotzko, D. J. (2005). Detrimental and neutral effects of wild barley–Neotyphodium fungal endophyte associations on insect survival. Entomol. Exp. Appl. 114, 119–125. doi: 10.1111/j.1570-7458.2005.00236.x
Cobian, G. M., Egan, C. P., and Amend, A. S. (2019). Plant–microbe specificity varies as a function of elevation. ISME J. 13, 2778–2788. doi: 10.1038/s41396-019-0470-4
Daba, A., Berecha, G., Tadesse, M., and Belay, A. (2021). Evaluation of the herbicidal potential of some fungal species against Bidens pilosa, the coffee farming weeds. Saudi J Biol Sci. 28, 6408–6416. doi: 10.1016/j.sjbs.2021.07.011
Dastogeer, K. M. G. (2018). Influence of fungal endophytes on plant physiology is more pronounced under stress than well-watered conditions: a meta-analysis. Planta 248, 1403–1416. doi: 10.1007/s00425-018-2982-y
El-Mahdy, O. M., Mohamed, H. I., and Mogazy, A. M. (2021). Biosorption effect of aspergillus Niger and Penicillium chrysosporium for cd-and Pb-contaminated soil and their physiological effects on Vicia faba L. Environ. Sci. Pollut. Res. 28, 67608–67631. doi: 10.1007/s11356-021-15382-4
Fadiji, A. E., and Babalola, O. O. (2020). Elucidating mechanisms of endophytes used in plant protection and other bioactivities with multifunctional prospects. Front. Bioeng. Biotechnol. 8:467. doi: 10.3389/fbioe.2020.00467
Fan, Y., Liu, X., Keyhani, N. O., Tang, G., Pei, Y., Zhang, W., et al. (2017). Regulatory cascade and biological activity of Beauveria bassiana oosporein that limits bacterial growth after host death. Proc. Natl. Acad. Sci. U. S. A. 114, E1578–E1586. doi: 10.1073/pnas.1616543114
Farhat, H., Urooj, F., Sohail, N., Ansari, M., and Ehteshamul-Haque, S. (2022). Evaluation of nematicidal potential of endophytic fungi associated with healthy plants and GC–MS profiling of metabolites of endophytic fusarium solani. S. Afr. J. Bot. 146, 146–161. doi: 10.1016/j.sajb.2021.10.011
Ferus, P., Barta, M., and Konôpková, J. (2019). Endophytic fungus Beauveria bassiana can enhance drought tolerance in red oak seedlings. Trees 33, 1179–1186. doi: 10.1007/s00468-019-01854-1
Fontana, D. C., De Paula, S., Torres, A. G., De Souza, V. H. M., Pascholati, S. F., Schmidt, D., et al. (2021). Endophytic fungi: biological control and induced resistance to phytopathogens and abiotic stresses. Pathogens 10:570. doi: 10.3390/pathogens10050570
Fuchs, B., and Krauss, J. (2019). Can Epichloë endophytes enhance direct and indirect plant defence? Fungal Ecol. 38, 98–103. doi: 10.1016/j.funeco.2018.07.002
Gafur, A. (2023). “Red root rot disease of tropical estate forests: pathogen identification, dispersal and management” in Detection, diagnosis and Management of Soil-borne Phytopathogens. eds. U. B. Singh, R. Kumar, and H. B. Singh (Singapore, Singapore: Springer Nature), 159–178.
Gafur, A., Naz, R., Nosheen, A., and Sayyed, R. Z. (2023). “Role of plant growth promoting microbes in managing soil-borne pathogens in forestry” in Plant growth promoting microorganisms of arid region. eds. R. Mawar, R. Z. Sayyed, S. K. Sharma, and K. S. Sattiraju (Singapore, Singapore: Springer Nature), 213–227.
Galindo-Solís, J. M., and Fernández, F. J. (2022). Endophytic fungal terpenoids: natural role and bioactivities. Microorganisms 10:339. doi: 10.3390/microorganisms10020339
Galletti, S., Paris, R., and Cianchetta, S. (2020). Selected isolates of Trichoderma gamsii induce different pathways of systemic resistance in maize upon fusarium verticillioides challenge. Microbiol. Res. 233:126406. doi: 10.1016/j.micres.2019.126406
Giller, K. E., Delaune, T., Silva, J. V., Descheemaeker, K., van de Ven, G., Schut, A. G., et al. (2021). The future of farming: who will produce our food? Food Secur 13, 1073–1099. doi: 10.1007/s12571-021-01184-6
Goettel, M. S. (2008). Are entomopathogenic fungi only entomopathogens? A preamble. J. Invertebr. Pathol. 98:255. doi: 10.1016/j.jip.2008.06.001
Gul, H. T., Saeed, S., and Khan, F. A. (2014). Entomopathogenic fungi as effective insect pest management tactic: a review. Appl Sci Bus Econ 1, 10–18.
Gupta, S., Schillaci, M., Walker, R., Smith, P. M. C., Watt, M., and Roessner, U. (2021). Alleviation of salinity stress in plants by endophytic plant-fungal symbiosis: current knowledge, perspectives and future directions. Plant and Soil 461, 219–244. doi: 10.1007/s11104-020-04618-w
Gurulingappa, P., Sword, G. A., Murdoch, G., and McGee, P. A. (2010). Colonization of crop plants by fungal entomopathogens and their effects on two insect pests when in planta. Biol. Control 55, 34–41. doi: 10.1016/j.biocontrol.2010.06.011
Hamayun, M., Hussain, A., Khan, S. A., Kim, H. Y., Khan, A. L., Waqas, M., et al. (2017). Gibberellins producing endophytic fungus Porostereum spadiceum AGH786 rescues growth of salt affected soybean. Front. Microbiol. 8:686. doi: 10.3389/fmicb.2017.00686
Harman, G. E., Doni, F., Khadka, R. B., and Uphoff, N. (2021). Endophytic strains of Trichoderma increase plants' photosynthetic capability. J. Appl. Microbiol. 130, 529–546. doi: 10.1111/jam.14368
Hassanein, N., El-Gendy, M., and Abdelhameed, N. (2020). Molecular typing, biodiversity, and biological control of endophytic fungi of Triticum aestivum L. against phytopathogenic fungi of wheat. Biotechnologia 101, 283–299. doi: 10.5114/bta.2020.100421
He, W., Guo, L., Wang, L., Zhao, Q., Guo, L., Cao, W., et al. (2019). Host genotype and precipitation influence of fungal endophyte symbiosis and mycotoxin abundance in a locoweed. Int. J. Mol. Sci. 20:5285. doi: 10.3390/ijms20215285
Hereme, R., Morales-Navarro, S., Ballesteros, G., Barrera, A., Ramos, P., Gundel, P. E., et al. (2020). Fungal endophytes exert positive effects on Colobanthus quitensis under water stress but neutral under a projected climate change scenario in Antarctica. Front. Microbiol. 11:264. doi: 10.3389/fmicb.2020.00264
Hernández-Rosas, F., Figueroa-Rodríguez, K. A., García-Pacheco, L. A., Velasco-Velasco, J., and Sangerman-Jarquín, D. M. (2020). Microorganisms and biological pest control: an analysis based on a bibliometric review. Agronomy 10:1808. doi: 10.3390/agronomy10111808
Huang, L. Q., Niu, Y. C., Su, L., Deng, H., and Lyu, H. (2020). The potential of endophytic fungi isolated from cucurbit plants for biocontrol of soilborne fungal diseases of cucumber. Microbiol. Res. 231:126369. doi: 10.1016/j.micres.2019.126369
Hughes, A. R., Moore, A. F. P., and Gehring, C. (2020). Plant response to fungal root endophytes varies by host genotype in the foundation species Spartina alterniflora. Am. J. Bot. 107, 1645–1653. doi: 10.1002/ajb2.1573
Ikram, M., Ali, N., Jan, G., Jan, F. G., Rahman, I. U., Iqbal, A., et al. (2018). IAA producing fungal endophyte Penicillium roqueforti Thom., enhances stress tolerance and nutrients uptake in wheat plants grown on heavy metal contaminated soils. PloS One 13:e0208150. doi: 10.1371/journal.pone.0208150
Illescas, M., Pedrero-Méndez, A., Pitorini-Bovolini, M., Hermosa, R., and Monte, E. (2021). Phytohormone production profiles in Trichoderma species and their relationship to wheat plant responses to water stress. Pathogens 10:991. doi: 10.3390/pathogens10080991
Ismail, I., Hamayun, M., Hussain, A., Khan, S. A., Iqbal, A., and Lee, I. J. (2020). An endophytic fungus aspergillus violaceofuscus can be used as heat stress adaptive tool for Glycine max L. and Helianthus annuus L. J. Appl. Bot. Food Qual. 93, 112–120. doi: 10.5073/JABFQ.2020.093.014
Jaber, L. R. (2018). Seed inoculation with endophytic fungal entomopathogens promotes plant growth and reduces crown and root rot (CRR) caused by fusarium culmorum in wheat. Planta 248, 1525–1535. doi: 10.1007/s00425-018-2991-x
Jaber, L. R., and Araj, S. E. (2018). Interactions among endophytic fungal entomopathogens (Ascomycota: Hypocreales), the green peach aphid Myzus persicae Sulzer (Homoptera: Aphididae), and the aphid endoparasitoid Aphidius colemani Viereck (Hymenoptera: Braconidae). Biol. Control 116, 53–61. doi: 10.1016/j.biocontrol.2017.04.005
Jagannath, S., Konappa, N., Lokesh, A., Bhuvaneshwari, D. T., Udayashankar, A. C., Chowdappa, S., et al. (2021). Bioactive compounds guided diversity of endophytic fungi from Baliospermum montanum and their potential extracellular enzymes. Anal. Biochem. 614:114024. doi: 10.1016/j.ab.2020.114024
Jain, P., and Pundir, R. K. (2017). “Potential role of endophytes in sustainable agriculture-recent developments and future prospects” in Endophytes: Biology and biotechnology. ed. D. K. Maheshwari (Cham: Springer International Publishing), 145–169.
Jin, H. Q., Liu, H. B., Xie, Y. Y., Zhang, Y. G., Xu, Q. Q., Mao, L. J., et al. (2018). Effect of the dark septate endophytic fungus Acrocalymma vagum on heavy metal content in tobacco leaves. Symbiosis 74, 89–95. doi: 10.1007/s13199-017-0485-4
Kamran, M., Imran, Q. M., Ahmed, M. B., Falak, N., Khatoon, A., and Yun, B. (2021). Endophyte-mediated stress tolerance in plants: a sustainable strategy to enhance resilience and assist crop improvement. Cells 11:3292. doi: 10.3390/cells11203292
Kaur, T. (2020). “Fungal endophyte-host plant interactions: role in sustainable agriculture” in Sustainable crop production. eds. M. Hasanuzzaman, M. C. M. T. Filho, M. Fujita, and T. A. R. Nogueira (Rijeka: IntechOpen), 14.
Keyser, C. A., Thorup-Kristensen, K., and Meyling, N. V. (2014). Metarhizium seed treatment mediates fungal dispersal via roots and induces infections in insects. Fungal Ecol. 11, 122–131. doi: 10.1016/j.funeco.2014.05.005
Khan, A. L., Hamayun, M., Ahmad, N., Hussain, J., Kang, S. M., Kim, Y. H., et al. (2011). Salinity stress resistance offered by endophytic fungal interaction between Penicillium minioluteum LHL09 and Glycine max. L. J. Microbiol. Biotechnol. 21, 893–902. doi: 10.4014/jmb.1103.03012
Khan, S. A., Hamayun, M., Yoon, H., Kim, H. Y., Suh, S. J., Hwang, S. K., et al. (2008). Plant growth promotion and Penicillium citrinum. BMC Microbiol. 10, 8:231. doi: 10.1186/1471-2180-8-231
Khan, A. L., Waqas, M., and Lee, I. J. (2014). Resilience of Penicillium resedanum LK6 and exogenous gibberellin in improving Capsicum annuum growth under abiotic stresses. J. Plant Res. 128, 259–268. doi: 10.1007/s10265-014-0688-1
Khan, B., Yan, W., Wei, S., Wang, Z., Zhao, S., Cao, L., et al. (2019). Nematicidal metabolites from endophytic fungus Chaetomium globosum YSC5. FEMS Microbiol. Lett. 366:fnz169. doi: 10.1093/femsle/fnz169
Khiralla, A., Spina, R., Yagi, S., Mohamed, L., and Laurain-Mattar, D. (2016). “Endophytic fungi: occurrence, classification, function and natural products” in Endophytic fungi: Diversity, characterization and biocontrol. ed. E. Hughes (New York, NY: Nova Science), 1–19.
Kiarie, S., Nyasani, J. O., Gohole, L. S., Maniania, N. K., and Subramanian, S. (2020). Impact of fungal endophyte colonization of maize (Zea mays L.) on induced resistance to thrips- and aphid-transmitted viruses. Plan. Theory 9:416. doi: 10.3390/plants9040416
Klieber, J., and Reineke, A. (2016). The entomopathogen Beauveria bassiana has epiphytic and endophytic activity against the tomato leaf miner Tuta absoluta. J. Appl. Entomol. 140, 580–589. doi: 10.1111/jen.12287
Kumar, A., Droby, S., Singh, V. K., Singh, S. K., and White, J. F. (2020). Entry, colonization, and distribution of endophytic microorganisms in plants. Microbial Endophyt 2020, 1–33. doi: 10.1016/B978-0-12-819654-0.00001-6
Lata, R., Chowdhury, S., Gond, S. K., and White, J. F. (2018). Induction of abiotic stress tolerance in plants by endophytic microbes. Lett. Appl. Microbiol. 66, 268–276. doi: 10.1111/lam.12855
Latz, M. A. C., Kerrn, M. H., Sørensen, H., Collinge, D. B., Jensen, B., Brown, J. K. M., et al. (2021). Succession of the fungal endophytic microbiome of wheat is dependent on tissue-specific interactions between host genotype and environment. Sci. Total Environ. 759:143804. doi: 10.1016/j.scitotenv.2020.143804
Le, K. D., Kim, J., Nguyen, H. T., Yu, N. H., Park, A. R., Lee, C. W., et al. (2021). Streptomyces sp. JCK-6131 protects plants against bacterial and fungal diseases via two mechanisms. Front. Plant Sci. 12:726266. doi: 10.3389/fpls.2021.726266
Le, H. T. T., Padgham, J. L., Hagemann, M. H., Sikora, R. A., and Schouten, A. (2016). Developmental and behavioural effects of the endophytic fusarium moniliforme Fe14 towards Meloidogyne graminicola in rice. Ann. Appl. Biol. 169, 134–143. doi: 10.1111/aab.12287
Lestari, S. M., Hidayat, S. H., and Widodo, W. (2018). Determination of endophytic fungi as induce resistance agent of chilli pepper against pepper yellow leaf curl disease. AGRIVITA J. Agri Sci 40, 249–256. doi: 10.17503/agrivita.v40i2.989
Liarzi, O., Bucki, P., Braun Miyara, S., and Ezra, D. (2016). Bioactive volatiles from an endophytic Daldinia cf. concentrica isolate affect the viability of the plant parasitic nematode Meloidogyne javanica. PloS One 11:e0168437. doi: 10.1371/journal.pone.0168437
Liu, X., Zhou, Y., Cui, L., Wang, L., and Wang, H. (2021). Biotransformation ability of endophytic fungi: from species evolution to industrial applications. Appl. Microbiol. Biotechnol. 105, 7095–7113. doi: 10.1007/s00253-021-11554-x
Lopez, D. C., and Sword, G. A. (2015). The endophytic fungal entomopathogens Beauveria bassiana and Purpureocillium lilacinum enhance the growth of cultivated cotton (Gossypium hirsutum) and negatively affect survival of the cotton bollworm (Helicoverpa zea). Biol. Control 89, 53–60. doi: 10.1016/j.biocontrol.2015.03.010
Lu, D., Ma, Z., Xu, X., and Yu, X. (2016). Isolation and identification of biocontrol agent Streptomyces rimosus M527 against fusarium oxysporum f. sp. Cucumerinum. J. Basic Microbiol. 56, 929–933. doi: 10.1002/jobm.201500666
Lu, X., Sun, Y., Li, Y., Zhang, X., Zhao, Y., and Feng, B. (2021). Terpenoid derivatives from the endophytic fungus fusarium sp. HJT-P-2 of Rhodiola angusta Nakai. Phytochem. Lett. 45, 48–51. doi: 10.1016/j.phytol.2021.07.011
Lugtenberg, B. J., Caradus, J. R., and Johnson, L. J. (2016). Fungal endophytes for sustainable crop production. FEMS Microbiol. Ecol. 92:fiw194. doi: 10.1093/femsec/fiw194
Maciá-Vicente, J. G., Rosso, L. C., Ciancio, A., Jansson, H. B., and Lopez-Llorca, L. V. (2009). Colonization of barley roots by endophytic fusarium equiseti and Pochonia chlamydosporia: effects on plant growth and disease. Ann. Appl. Biol. 155, 391–401. doi: 10.1111/j.1744-7348.2009.00352.x
Mantzoukas, S., and Eliopoulos, P. A. (2020). Endophytic entomopathogenic fungi: a valuable biological control tool against plant pests. Appl. Sci. 10:360. doi: 10.3390/app10010360
Mejía, L. C., Rojas, E. I., Maynard, Z., Bael, S. V., Arnold, A. E., Hebbar, P., et al. (2008). Endophytic fungi as biocontrol agents of Theobroma cacao pathogens. Biol. Control 46, 4–14. doi: 10.1016/j.biocontrol.2008.01.012
Menjivar, B. R. D. (2010) The systemic activity of mutualistic endophytic fungi in Solanaceae and Cucurbitaceae plants on the behavior of the phloem-feeding insects Trialeurodes vaporariorum, Aphis gossypii and Myzus persicae (Doctoral dissertation, Universitäts-und Landesbibliothek Bonn).
Miao, G. P., Han, J., Zhang, K. G., Wang, S. C., and Wang, C. R. (2019). Protection of melon against fusarium wilt-root knot nematode complex by endophytic fungi Penicillium brefeldianum HS-1. Symbiosis 77, 83–89. doi: 10.1007/s13199-018-0565-0
Moghaddam, M. S. H., Safaie, N., Soltani, J., and Hagh-Doust, N. (2021). Desert-adapted fungal endophytes induce salinity and drought stress resistance in model crops. Plant Physiol. Biochem. 160, 225–238. doi: 10.1016/j.plaphy.2021.01.022
Molina-Montenegro, M. A., Oses, R., Torres-Díaz, C., Atala, C., Zurita-Silva, A., and Ruiz-Lara, S. (2016). Root-endophytes improve the ecophysiological performance and production of an agricultural species under drought condition. AoB Plants 8:plw062. doi: 10.1093/aobpla/plw062
Monteiro, M., Tavares, D., Nery, E., Queiroz, M., Pereira, O., and Cardoso, P. (2020). Enzyme production by Induratia spp. isolated from coffee plants in Brazil. Braz. Arch. Biol. Technol. 63:673. doi: 10.1590/1678-4324-2020180673
Moraes, G. K., Ferraz, L. F., and Chapla, V. M. (2020). Volatile organic compounds of endophytic fungi and biotechnological applications. Rev Virtual Quim 12, 1498–1510. doi: 10.21577/1984-6835.20200116
Morales-Sánchez, V., Fe Andrés, M., Díaz, C. E., and González-Coloma, A. (2020). Factors affecting the metabolite productions in endophytes: biotechnological approaches for production of metabolites. Curr. Med. Chem. 27, 1855–1873. doi: 10.2174/0929867326666190626154421
Morsy, M., Cleckler, B., and Armuelles-Millican, H. (2020). Fungal endophytes promote tomato growth and enhance drought and salt tolerance. Plan. Theory 9:877. doi: 10.3390/plants9070877
Mota, S. F., Pádua, P. F., Ferreira, A. N., Gomes L, D. B. W., Dias, M. A., Souza, E. A., et al. (2021). Biological control of common bean diseases using endophytic Induratia spp. Biol. Control 159:104629. doi: 10.1016/j.biocontrol.2021.104629
Moura, M. S., Lacerda, J. W., Siqueira, K. A., Bellete, B. S., Sousa, P. T. Jr., Dall Óglio, E. L., et al. (2020). Endophytic fungal extracts: evaluation as photosynthesis and weed growth inhibitors. J. Environ. Sci. Health B 55, 470–476. doi: 10.1080/03601234.2020.1721981
Muñoz-Guerrero, J., Guerra-Sierra, B. E., and Alvarez, J. C. (2021). Fungal endophytes of Tahiti lime (Citrus citrus × latifolia) and their potential for control of Colletotrichum acutatum J. H. Simmonds causing anthracnose. Front. Bioeng. Biotechnol. 9:650351. doi: 10.3389/fbioe.2021.650351
Mutune, B., Ekesi, S., Niassy, S., Matiru, V., Bii, C., and Maniania, N. K. (2016). Fungal endophytes as promising tools for the management of bean stem maggot Ophiomyia phaseoli on beans Phaseolus vulgaris. J. Pestic. Sci. 89, 993–1001. doi: 10.1007/s10340-015-0725-4
Muvea, A. M., Meyhöfer, R., Maniania, N. K., Poehling, H. M., Ekesi, S., and Subramanian, S. (2015). Behavioral responses of Thrips tabaci Lindeman to endophyte-inoculated onion plants. J. Pestic. Sci. 88, 555–562. doi: 10.1007/s10340-015-0645-3
Nassimi, Z., and Taheri, P. (2017). Endophytic fungus Piriformospora indica induced systemic resistance against rice sheath blight via affecting hydrogen peroxide and antioxidants. Biocontrol Sci. Tech. 27, 252–267. doi: 10.1080/09583157.2016.1277690
Nemat Alla, M. M., Shabana, Y. M., Serag, M. M., Hassan, N. M., and El-Hawary, M. M. (2008). Granular formulation of fusarium oxysporum for biological control of faba bean and tomato Orobanche. Pest Manag. Sci. 64, 1237–1249. doi: 10.1002/ps.1625
Nuraini, F. R., Setyaningsih, R., and Susilowati, A. (2017). Screening and characterization of endophytic fungi as antagonistic agents toward fusarium oxysporum on eggplant (Solanum melongena). Biodiversitas J Biol Divers 18, 1377–1384. doi: 10.13057/biodiv/d180413
Ogbe, A. A., Finnie, J. F., and Van Staden, J. (2020). The role of endophytes in secondary metabolites accumulation in medicinal plants under abiotic stress. S. Afr. J. Bot. 134, 126–134. doi: 10.1016/j.sajb.2020.06.023
Poveda, J., Abril-Urias, P., and Escobar, C. (2020). Biological control of plant-parasitic nematodes by filamentous fungi inducers of resistance: Trichoderma, mycorrhizal and endophytic fungi. Front. Microbiol. 11:992. doi: 10.3389/fmicb.2020.00992
Qayyum, M. A., Wakil, W., Arif, M. J., Sahi, S. T., and Dunlap, C. A. (2015). Infection of Helicoverpa armigera by endophytic Beauveria bassiana colonizing tomato plants. Biol. Control. 90, 200–207. doi: 10.1016/j.biocontrol.2015.04.005
Quesada-Moraga, E., López-Díaz, C., and Landa, B. B. (2014). The hidden habit of the entomopathogenic fungus Beauveria bassiana: first demonstration of vertical plant transmission. PloS One 9:e89278. doi: 10.1371/journal.pone.0089278
Rajani, P., Rajasekaran, C., Vasanthakumari, M. M., Olsson, S. B., Ravikanth, G., and Shaanker, R. U. (2021). Inhibition of plant pathogenic fungi by endophytic Trichoderma spp. through mycoparasitism and volatile organic compounds. Microbiol. Res. 242:126595. doi: 10.1016/j.micres.2020.126595
Rasool, S., Vidkjaer, N. H., Hooshmand, K., Jensen, B., Fomsgaard, I. S., and Meyling, N. V. (2021). Seed inoculations with entomopathogenic fungi affect aphid populations coinciding with modulation of plant secondary metabolite profiles across plant families. New Phytol. 229, 1715–1727. doi: 10.1111/nph.16979
Rigobelo, E. C., and Baron, N. C. (2021). Endophytic fungi: a tool for plant growth promotion and sustainable agriculture. Mycology 13, 39–55. doi: 10.1080/21501203.2021.1945699
Rodriguez, R. J., Henson, J., Van Volkenburgh, E., Hoy, M., Wright, L., Beckwith, F., et al. (2008). Stress tolerance in plants via habitat-adapted symbiosis. ISME J. 2, 404–416. doi: 10.1038/ismej.2007.106
Rondot, Y., and Reineke, A. (2019). Endophytic Beauveria bassiana activates expression of defence genes in grapevine and prevents infections by grapevine downy mildew Plasmopara viticola. Plant Pathol. 68, 1719–1731. doi: 10.1111/ppa.13089
Saad, M. M. G., and Badry, H. H. (2020). Phytohormones producing fungal endophytes enhance nutritional status and suppress pathogenic fungal infection in tomato. J. Agric. Sci. Technol. 22, 1383–1395. Available at: http://dorl.net/dor/20.1001.1.16807073.2020.22.5.1.5
Sallam, N., Ali, E. F., Seleim, M. A. A., and Bagy, H. M. M. K. (2021). Endophytic fungi associated with soybean plants and their antagonistic activity against Rhizoctonia solani. Egypt J Biol Pest Control 31:54. doi: 10.1186/s41938-021-00402-9
Satheesan, J., and Sabu, K. K. (2020). “Endophytic fungi for a sustainable production of major plant bioactive compounds” in Plant-derived bioactives: Production, properties and therapeutic applications. ed. M. K. Swamy (Singapore: Springer), 195–207.
Sayed, S., El-Shehawi, A., Al-Otaibi, S., El-Shazly, S., Al-Otaibi, S., Ibrahim, R., et al. (2020). Isolation and efficacy of the endophytic fungus, Beauveria bassiana (Bals.) Vuillemin on grapevine aphid, Aphis illinoisensis Shimer (Hemiptera: Aphididae) under laboratory conditions. Egypt J Biol Pest Control 30, 1–7. doi: 10.1186/s41938-020-00234-z
Schouten, A. (2016). Mechanisms involved in nematode control by endophytic fungi. Annu. Rev. Phytopathol. 54, 121–142. doi: 10.1146/annurev-phyto-080615-100114
Sharma, S., Kour, D., Rana, K. L., Dhiman, A., Thakur, S., Thakur, P., et al. (2019). “Trichoderma: biodiversity, ecological significances, and industrial applications” in Recent advancement in white biotechnology through fungi: volume 1: diversity and enzymes perspectives. eds. A. N. Yadav, S. Mishra, S. Singh, and A. Gupta (Cham: Springer International Publishing), 85–120.
Shymanovich, T., and Faeth, S. H. (2018). Anti-insect defenses of Achnatherum robustum (sleepygrass) provided by two Epichloë endophyte species. Entomol. Exp. Appl. 166, 474–482. doi: 10.1111/eea.12692
Singh, N., Singh, A., and Dahiya, P. (2021). “Plant growth-promoting endophytic fungi from different habitats and their potential applications in agriculture” in Recent trends in mycological research: volume 1: agricultural and medical perspective. ed. A. N. Yadav (Cham: Springer International Publishing), 69–87.
Sinno, M., Ranesi, M., Gioia, L., D’Errico, G., and Woo, S. L. (2020). Endophytic fungi of tomato and their potential applications for crop improvement. Agriculture 10:587. doi: 10.3390/agriculture10120587
Sreeja, K., Anandaraj, M., and Bhai, R. S. (2016). In vitro evaluation of fungal endophytes of black pepper against Phytophthora capsici and Radopholus similis. J Spices Aromat Crops 25, 113–122. Available at: https://updatepublishing.com/journal/index.php/josac/article/view/5174
Sujatha, H. S., Murali, M., and Amruthesh, K. N. (2021). Fungal endophytes as growth promoters and inducers of resistance in tomato (Lycopersicon esculentum mill.) against Alternaria solani. Int J Life Sci Pharma Res 11, L227–L235. doi: 10.22376/ijpbs/lpr.2021.11.2.L227-235
Sun, C., Johnson, J. M., Cai, D., Sherameti, I., Oelmüller, R., and Lou, B. (2010). Piriformospora indica confers drought tolerance in Chinese cabbage leaves by stimulating antioxidant enzymes, the expression of drought-related genes and the plastid-localized CAS protein. J. Plant Physiol. 167, 1009–1017. doi: 10.1016/j.jplph.2010.02.013
Suryanarayanan, T. S. (2019). Endophytes and weed management: a commentary. Plant Physiol Rep 24, 576–579. doi: 10.1007/s40502-019-00488-2
Swarnakumari, N., and Kalaiarasan, P. (2017). Mechanism of nematode infection by fungal antagonists, Purpureocillium lilacinum (Thom) Samson and Pochonia chlamydosporia (Goddard) Zare & Gams 2001. Pest Manag Hortic Ecosyst 23, 165–169.
Syamsia, S., Idhan, A., Firmansyah, A. P., Noerfitryani, N., Rahim, I., Kesaulya, H., et al. (2021). Combination on endophytic fungal as the plant growth-promoting fungi (PGPF) on cucumber (Cucumis sativus). Biodiversitas J Biol Divers 22, 1194–1202. doi: 10.13057/biodiv/d220315
Tefera, T., and Vidal, S. (2009). Effect of inoculation method and plant growth medium on endophytic colonization of sorghum by the entomopathogenic fungus Beauveria bassiana. BioControl 54, 663–669. doi: 10.1007/s10526-009-9216-y
Tian, L., Wang, E., Lin, X., Ji, L., Chang, J., Chen, H., et al. (2021). Wild rice harbors more root endophytic fungi than cultivated rice in the F1 offspring after crossbreeding. BMC Genomics 22:278. doi: 10.1186/s12864-021-07587-1
Tian, X., Yao, Y., Chen, G., Mao, Z., Wang, X., and Xie, B. (2014). Suppression of Meloidogyne incognita by the endophytic fungus Acremonium implicatum from tomato root galls. Int J Pest Manag 60, 239–245. doi: 10.1080/09670874.2014.958604
Toffa, J., Loko, Y. L. E., Kpindou, O. K. D., Zanzana, K., Adikpeto, J., Gbenontin, Y., et al. (2021). Endophytic colonization of tomato plants by Beauveria bassiana Vuillemin (Ascomycota: Hypocreales) and leaf damage in Helicoverpa armigera (Hübner) (Lepidoptera: Noctuidae) larvae. Egypt J Biol Pest Control 31:82. doi: 10.1186/s41938-021-00431-4
Toghueo, R. M. K., Eke, P., Zabalgogeazcoa, Í., De Aldana, B. R. V., Nana, L. W., and Boyom, F. F. (2016). Biocontrol and growth enhancement potential of two endophytic Trichoderma spp. from Terminalia catappa against the causative agent of common bean root rot (fusarium solani). Biol. Control 96, 8–20. doi: 10.1016/j.biocontrol.2016.01.008
Tolba, S. R. T., Rosso, L. C., Pentimone, I., Colagiero, M., Moustafa, M. M. A., Elshawaf, I. I. S., et al. (2021). Root endophytism by Pochonia chlamydosporia affects defense-gene expression in leaves of monocot and dicot hosts under multiple biotic interactions. Plants 10:718. doi: 10.3390/plants10040718
Toppo, P., Subba, R., Roy, K., Mukherjee, S., and Mathur, P. (2023). Elucidating the strategies for isolation of endophytic fungi and their functional attributes for the regulation of plant growth and resilience to stress. J. Plant Growth Regul. 42, 1342–1363. doi: 10.1007/s00344-022-10638-w
Trifonova, Z., Tsvetkov, I., Bogatzevska, N., and Batchvarova, R. (2014). Efficiency of Pseudomonas spp. for bio control of the potato cyst nematode Globodera rostochiensis (Woll.). Bulg J Agric Sci 2, 666–669.
Tunali, B., Kansu, B., and Berner, D. K. (2009). Biological control studies on Convolvulus arvensis L. with fungal pathogens. J Turk Phytopathol 38, 1–8.
Urooj, F., Farhat, H., Tariq, A., Moin, S., Sohail, N., Sultana, V., et al. (2021). Role of endophytic Penicillium species and Pseudomonas monteilii in inducing the systemic resistance in okra against root rotting fungi and their effect on some physiochemical properties of okra fruit. J. Appl. Microbiol. 130, 604–616. doi: 10.1111/jam.14894
Van Dessel, P., Coyne, D., Dubois, T., De Waele, D., and Franco, J. (2011). In vitro nematicidal effect of endophytic fusarium oxysporum against Radopholus similis, Pratylenchus goodeyi and Helicotylenchus multicinctus. Nematropica 41, 154–160.
Vega, F. E., Posada, F., Aime, M. C., Pava-Ripoll, M., Infante, F., and Rehner, S. A. (2008). Entomopathogenic fungal endophytes. Biol. Control 46, 72–82. doi: 10.1016/j.biocontrol.2008.01.008
Verma, A., Shameem, N., Jatav, H. S., Sathyanarayana, E., Parray, J. A., Poczai, P., et al. (2022). Fungal endophytes to combat biotic and abiotic stresses for climate-smart and sustainable agriculture. Front. Plant Sci. 13:953836. doi: 10.3389/fpls.2022.953836
Vu, T., Hauschild, R., and Sikora, R. A. (2006). Fusarium oxysporum endophytes induced systemic resistance against Radopholus similis on banana. Nematology 8, 847–852. doi: 10.1163/156854106779799259
Wang, J. L., Li, T., Liu, G. Y., Smith, J. M., and Zhao, Z. W. (2016). Unraveling the role of dark septate endophyte (DSE) colonizing maize (Zea mays) under cadmium stress: physiological, cytological and genic aspects. Sci. Rep. 6:22028. doi: 10.1038/srep22028
Wang, C., and St Leger, R. J. (2007). A scorpion neurotoxin increases the potency of a fungal insecticide. Nat. Biotechnol. 25, 1455–1456. doi: 10.1038/nbt1357
Wang, H., Sun, S., Ge, W., Zhao, L., Hou, B., Wang, K., et al. (2020). Horizontal gene transfer of Fhb7 from fungus underlies fusarium head blight resistance in wheat. Science 368:eaba5435. doi: 10.1126/science.aba5435
Waqas, M., Khan, A. L., Hamayun, M., Shahzad, R., Kim, Y. H., Choi, K. S., et al. (2015a). Endophytic infection alleviates biotic stress in sunflower through regulation of defence hormones, antioxidants and functional amino acids. Eur. J. Plant Pathol. 141, 803–824. doi: 10.1007/s10658-014-0581-8
Waqas, M., Khan, A. L., Shahzad, R., Ullah, I., Khan, A. R., and Lee, I. J. (2015b). Mutualistic fungal endophytes produce phytohormones and organic acids that promote japonica rice plant growth under prolonged heat stress. J. Zhejiang Univ. Sci. B 16, 1011–1018. doi: 10.1631/jzus.B1500081
Waweru, B., Turoop, L., Kahangi, E., Coyne, D., and Dubois, T. (2014). Non-pathogenic fusarium oxysporum endophytes provide field control of nematodes, improving yield of banana (Musa sp.). Biol. Control 74, 82–88. doi: 10.1016/j.biocontrol.2014.04.002
Wearn, J. A., Sutton, B. C., Morley, N. J., and Gange, A. C. (2012). Species and organ specificity of fungal endophytes in herbaceous grassland plants. J. Ecol. 100, 1085–1092. doi: 10.1111/j.1365-2745.2012.01997.x
Wei, Q. Y., Li, Y. Y., Xu, C., Wu, Y. X., Zhang, Y. R., and Liu, H. (2020). Endophytic colonization by Beauveria bassiana increases the resistance of tomatoes against Bemisia tabaci. Arthropod Plant Interact. 14, 289–300. doi: 10.1007/s11829-020-09746-9
Wei, F., Zhang, Y., Shi, Y., Feng, H., Zhao, L., Feng, Z., et al. (2019). Evaluation of the biocontrol potential of endophytic fungus fusarium solani CEF559 against Verticillium dahliae in cotton plant. Biomed. Res. Int. 2019, 1–12. doi: 10.1155/2019/3187943
Wei, Y., Zhao, Y., Zhou, D., Qi, D., Li, K., Tang, W., et al. (2020). A newly isolated Streptomyces sp. YYS-7 with a broad-spectrum antifungal activity improves the banana plant resistance to fusarium oxysporum f. sp. cubense tropical race 4. Front. Microbiol. 11:1712. doi: 10.3389/fmicb.2020.01712
White, J. F., Kingsley, K. L., Verma, S. K., and Kowalski, K. P. (2018). Rhizophagy cycle: an oxidative process in plants for nutrient extraction from symbiotic microbes. Microorganisms 6:95. doi: 10.3390/microorganisms6030095
White, J. F., Kingsley, K. L., Zhang, Q., Verma, R., Obi, N., Dvinskikh, S., et al. (2019). Review: endophytic microbes and their potential applications in crop management. Pest Manag. Sci. 75, 2558–2565. doi: 10.1002/ps.5527
Wu, L. S., Dong, W. G., Si, J. P., Liu, J. J., and Zhu, Y. Q. (2020). Endophytic fungi, host genotype, and their interaction influence the growth and production of key chemical components of Dendrobium catenatum. Fungal Biol. 124, 864–876. doi: 10.1016/j.funbio.2020.07.002
Yan, X. N., Sikora, R. A., and Zheng, J. W. (2011). Potential use of cucumber (Cucumis sativus L.) endophytic fungi as seed treatment agents against root-knot nematode Meloidogyne incognita. J. Zhejiang Univ. Sci. B 12, 219–225. doi: 10.1631/jzus.B1000165
Yan, L., Zhu, J., Zhao, X., Shi, J., Jiang, C., and Shao, D. (2019). Beneficial effects of endophytic fungi colonization on plants. Appl. Microbiol. Biotechnol. 103, 3327–3340. doi: 10.1007/s00253-019-09713-2
Yang, B., Wang, X. M., Ma, H. Y., Jia, Y., Li, X., and Dai, C. C. (2014). Effects of the fungal endophyte Phomopsis liquidambari on nitrogen uptake and metabolism in rice. Plant Growth Regul. 73, 165–179. doi: 10.1007/s10725-013-9878-4
Yuan, X. L., Wang, X. F., Xu, K., Li, W., Chen, D., and Zhang, P. (2020). Characterization of a new insecticidal anthraquinone derivative from an endophyte of Acremonium vitellinum against Helicoverpa armigera. J. Agric. Food Chem. 68, 11480–11487. doi: 10.1021/acs.jafc.0c05680
Yung, L., Sirguey, C., Azou-Barré, A., and Blaudez, D. (2021). Natural fungal endophytes from Noccaea caerulescens mediate neutral to positive effects on plant biomass, mineral nutrition and Zn phytoextraction. Front. Microbiol. 12:689367. doi: 10.3389/fmicb.2021.689367
Zanudin, N. A. B. M., Hasan, N., and Mansor, P. B. (2020). Antagonistic activity of fungal endophytes isolated from Garcinia atroviridis against Colletotrichum gloeosporioides. Hayati J Biosci 27, 209–214. doi: 10.4308/hjb.27.3.209
Zhang, X. Y., Liu, Z. L., Sun, B. D., Niu, S. B., Wang, M. H., Tan, X. M., et al. (2019). Bioactive resorcylic acid lactones with different ring systems from desert plant endophytic fungus Chaetosphaeronema hispidulum. J. Agric. Food Chem. 66, 8976–8982. doi: 10.1021/acs.jafc.8b02648
Zhou, W., Wheeler, T. A., Starr, J. L., Valencia, C. U., and Sword, G. A. (2018). A fungal endophyte defensive symbiosis affects plant-nematode interactions in cotton. Plant and Soil 422, 251–266. doi: 10.1007/s11104-016-3147-z
Keywords: agricultural pests, colonization, growth promoters, mutualistic endophytes, nutrient acquisition
Citation: Fite T, Kebede E, Tefera T and Bekeko Z (2023) Endophytic fungi: versatile partners for pest biocontrol, growth promotion, and climate change resilience in plants. Front. Sustain. Food Syst. 7:1322861. doi: 10.3389/fsufs.2023.1322861
Edited by:
Sudeep Tiwari, University of Nebraska-Lincoln, United StatesReviewed by:
Abdul Gafur, SMF Corporate R&D Advisory Board, IndonesiaSwarnalee Dutta, Jeonbuk National University, Republic of Korea
Navin Kumar, Agricultural Research Organization (ARO), Israel
Copyright © 2023 Fite, Kebede, Tefera and Bekeko. This is an open-access article distributed under the terms of the Creative Commons Attribution License (CC BY). The use, distribution or reproduction in other forums is permitted, provided the original author(s) and the copyright owner(s) are credited and that the original publication in this journal is cited, in accordance with accepted academic practice. No use, distribution or reproduction is permitted which does not comply with these terms.
*Correspondence: Tarekegn Fite, tfduressa@gmail.com; Erana Kebede, eranak.neda@gmail.com
†ORCID: Tarekegn Fite orcid.org/0000-0003-3492-4933
Erana Kebede orcid.org/0000-0002-3584-6757