- 1Departamento de Ciências do Solo, Universidade Federal Rural do Rio de Janeiro, Seropédica, Brazil
- 2Departamento de Fitotecnia, Universidade Federal Rural do Rio de Janeiro, Seropédica, Brazil
- 3Embrapa Agrobiologia, Seropédica, Brazil
- 4Embrapa Solos, Seropédica, Brazil
Dark septate endophytic (DSE) fungi are facultative biotrophs that associate with hundreds of plant species, contributing to their growth. These fungi may therefore aid in the search for sustainable agricultural practices. However, several ecological functions of DSE fungi need further clarification. The present study investigated the effects of DSE fungi inoculation on nutrient recovery efficiency, nutrient accumulation, and growth of tomato plants fertilized with organic and inorganic N sources. Two experiments were carried out under greenhouse conditions in a randomized blocks design, with five replicates of tomato seedlings grown in pots filled with non-sterile sandy soil. Tomato seedlings (cv. Santa Clara I-5300) inoculated with DSE fungi (isolates A101, A104, and A105) and without DSE fungi (control) were transplanted to pots filled with 12 kg of soil which had previously received finely ground plant material [Canavalia ensiformis (L.)] that was shoot enriched with 0.7 atom % 15N (organic N source experiment) or ammonium sulfate-15N enriched with 1 atom % 15N (mineral N source experiment). Growth indicators, nutrient content, amount of nitrogen (N) in the plant derived from ammonium sulfate-15N or C. ensiformis-15N, and recovery efficiency of 15N, P, and K by plants were quantified 50 days after transplanting. The treatment inoculated with DSE fungi and supplied with an organic N source showed significantly higher recovery efficiency of 15N, P, and K. In addition, the 15N, N, P, K, Ca, Mg, Fe, Mn, and Zn content, plant height, leaf number, leaf area (only for the A104 inoculation), and shoot dry matter increased. In contrast, the only positive effects observed in the presence of an inorganic N source were fertilizer-K recovery efficiency, content of K, and leaf area when inoculated with the fungus A104. Inoculation with A101, A104, and A105 promoted the growth of tomato using organic N source (finely ground C. ensiformis-15N plant material).
Introduction
In agriculture, especially in low-input or organic cultivation, plants can benefit from interactions with microorganisms, such as arbuscular mycorrhizal fungi (AMF) (He and Nara, 2007). In this case, their extraradicular mycelium provides a wide nutrient interception area and a high nutrient uptake efficiency, decreasing demands on applied fertilizers and reducing soil nutrient loss, in addition to improving plant nutrition and growth (Hogberg, 1989; Finlay and Söderström, 1992; He and Nara, 2007; Cavagnaro et al., 2015). Furthermore, evidence exists that AMF can acquire nutrients from organic matter, probably after its mineralization, and transfer them to the host plant (Hodge et al., 2001; Hodge and Fitter, 2010). However, fundamental studies and production of a high quality inoculum (free of impurities) of AMF have been limited by the fact that such fungi are obligate biotrophs, requiring establishment of effective associations with metabolically active roots for their development and multiplication (Sylvia and Jarstfer, 1994).
Dark septate endophytic (DSE) fungi are quite diverse facultative biotrophic ascomycetes characterized by dark pigmentation, melanized septate hyphae, and formation of microsclerotia inside the plant roots. Such fungi are capable of colonizing root tissues intra and intercellularly of more than 600 plant species [including Solanum lycopersicum (L.) and those non-mycorrhizal ones] without causing pathologies. They can also act as plant growth promoters (Jumpponen and Trappe, 1998; Upson et al., 2009; Andrade-Linares et al., 2011a,b; Yuan et al., 2011; Mahmoud and Narisawa, 2013).
Tomato plants belongs to the family Solanaceae and have been determined to be the second most economically important vegetable worldwide after the potato (Foolad, 2004; Reis Filgueira, 2008). Tomato fruits are an important source of lycopene, which exhibits antioxidant, hypolipidemic, and anti-carcinogenic properties (Viuda-Martos et al., 2014). In addition, tomatoes are cholesterol free and rich in mineral salts, vitamins A and C, and fibers (Block et al., 1992; Gerster, 1997; Rao and Agarwal, 2000; Viuda-Martos et al., 2014).
Studies have suggested that DSE fungi are capable of accessing complex carbon (C), nitrogen (N), and phosphorus (P) compounds within the soil when associated with plants (Mandyam and Jumpponen, 2005), making nutrients available to their host plant (Mandyam, 2008). This is probably because they produce hydrolytic enzymes, which induce release of nutrients absorbed by plants. Additionally, organic compounds taken up by DSE fungi, including amino acids and small peptides, are transferred directly to host plants (Jumpponen and Trappe, 1998), resulting in better use of organic nutrient sources (Reeve et al., 2008).
In previous studies, three isolates of DSE fungi, A101, A104, and A105 obtained from wild rice (Oryza glumaepatula [Steud.]) were determined by ITS phylogeny to belong to the order Pleosporales (suborder Massarineae) (A104 and A105) and to an unknown taxon (A101) (Ribeiro, 2011; Vergara et al., 2017). These fungi colonize wild [Oryza glumaepatula (Steud.)] and commercial [Oryza sativa (L.)] rice and tomato (cv. Santa Clara I-5300) plants, with no apparent symptoms of disease (Ribeiro, 2011; Vergara et al., 2017). Furthermore, a neutral effect was observed in the N content of tomato plants inoculated with these fungi when NH4NO3 (an inorganic source) was supplied to the plants.
In the present study, the effects of inoculation with three DSE fungi on tomato plants (cv. Santa Clara I-5300) was evaluated in non-sterile sand soil in greenhouse conditions. Plant growth, nutrient accumulation (N, P, K, Ca, Mg, Fe, Mn, and Zn), the amount of N in the plants derived from ammonium sulfate-15N or from a finely ground legume Canavalia ensiformis-15N, and recovery efficiency of 15N, P, and K by plants were determined. Thus, the present study investigated the effects of DSE inoculation on nutrient recovery efficiency, which is the proportion of a given fertilizer nutrient taken up by a plant, nutrient accumulation, and growth of tomato plants fertilized with organic and inorganic N sources.
Materials and Methods
Canavalia ensiformis (L.), a plant that is extensively used in tropical agriculture as a green manure for nutritional enrichment of soils (Rodrigues et al., 2004), and ammonium sulfate were used as N source for tomato plants inoculated with DSE fungi. To study the differences between these N sources, two experiments were conducted in parallel: (i) an experiment with the inorganic N source, ammonium sulfate; and (ii) an experiment with the organic N source, C. ensiformis. Ammonium sulfate is an important source of N, but also provides sulfur for plants. On the other hand, green manure C. ensiformis (a legume) contributes considerable amounts of N to the soil-plant system due to its association with N2 fixing bacteria (Perin et al., 2003). C. ensiformis also maintains soil moisture (Tejada et al., 2008) and mobilizes nutrients such as P and some micronutrients (Lambers et al., 2015).
Fertilization and Liming of the Soil for Both Experiments
For both experiment, soil samples were collected from an organic production system located at Seropédica Municipality, RJ, Brazil, at 0–20 cm depth. The soil was classified as Haplic Planosol (according to Brazilian Soil Taxonomy, or Planosol, under World Reference Base-FAO). Soil chemical analysis showed the following properties: pH = 5.61 in water; exchangeable Al3+ = 0.0 and H+Al = 2.95 cmolc dm−3 (centimoles of charge per dm3 soil); Ca+2 = 1.21 and Mg+2 = 0.65 cmolc dm−3; available P = 10.27 and K+ = 63.92 mg L−1; total N = 0.07% and C = 0.54%. The soil was classified as sandy soil (3% clay, 5% silt, and 92% sandy). For each experimental unit, a pot with a 14 L capacity was filled with 12 kg of a sieved and homogenized soil sample. Two months after lime addition (equivalent of 1.94 t ha−1; MineralCal) to correct for Ca+2 and Mg+2 deficiencies, the soil was fertilized with the equivalent of 320 kg P2O5 ha−1 (simple superphosphate), 68 kg K2O ha−1 (potassium sulfate), and 30 kg ha−1 micronutrient fertilizer as F.T.E BR-12 (fritted trace elements), according to recommendations for tomato crops (Macebo et al., 2013). Potassium was applied in two stages, 25 (27 kg K2O ha−1) and 40 (41 kg K2O ha−1) days after transplanting (DAT).
Nitrogen Fertilization Labeled by 15N
Ammonium sulfate was used as an inorganic fertilizer source. Application of ammonium sulfate enriched with 1 atom % 15N (ammonium sulfate-15N) was split into two equal levels (0.428 g per pot, equivalent to 15 kg ha−1 N), at 25 and 45 DAT (Macebo et al., 2013). On the day of fertilizer-15N application, 15N-labeled ammonium sulfate was dissolved in distilled water and 500 mL of the homogenized solution was evenly distributed in pots for soil labeling standardization by soil drenching.
For the experiment with an organic N source, dry, finely ground shoot biomass of Canavalia ensiformis (L.) was used. C. ensiformis plants were previously grown in 15N-enriched soil for use as green manure, and their dry shoot (dried 72 h at 65°C) enriched with 0.7 atom % 15N (C. ensiformis-15N) was sampled at around 60–70 days after germination (flowering period). The dry shoot of C. ensiformis was finely ground and then sterilized by gamma irradiation (25 kGy). Macro- (g kg−1) and micronutrient (mg kg−1) concentrations for C. ensiformis-15N were: N = 23.8; P = 2.0; K = 5.8; Ca = 12.3; Mg = 3.2; S = 1.9; Cu = 10.0; Fe = 792.0; Zn = 39.0; Mn = 50.0; B = 27.0; and C = 38.2%. Each pot was filled with 12 kg soil receiving 37.81 g of finely dry ground biomass of C. ensiformis, equivalent to 150 kg N ha−1, which was applied and homogenized before planting.
Inoculum Preparation and Inoculation
The fungal isolates used in the two experiments were obtained from O. glumaepatula and identified through ITS phylogeny (Ribeiro, 2011; Vergara et al., 2017). These fungi are deposited in the Centro de Recursos Biológicos Johanna Döbereiner (www.embrapa.br/agrobiologia/crb-jd) culture collection (A101, A104, and A105). The ITS region sequences are deposited in GenBank (KR817246 = A101, KR817249 = A104, and KR817250 = A105). The inoculum was obtained according to Andrade-Linares et al. (2011b), with some modifications. Each isolate was grown in a 300 mL Erlenmeyer flask containing 150 mL potato dextrose agar (PDA) medium for 2 weeks at 28°C under 80 rpm shaking. The fresh mycelium was filtered and washed with sterile distilled water until the liquid became clear to avoid transfer of any material from the PDA medium to the inoculum. Then, the mycelium was weighed and part of it was mixed with sterile distilled water for 1 min at minimum speed using a mixer (Arno Optimix Plus, model LN27, Brazil) operating at laminar flow to prevent contamination. The viability of each isolate was verified by plating the mycelium in the PDA medium. For inoculation, suspensions were adjusted with sterile distilled water to a concentration of 1% (w/v).
Experimental Design and Growth Conditions
Two experiments with tomato seedlings were carried out in parallel, in randomized blocks, with five replicates with one plant each (n = 5), under greenhouse conditions at Embrapa Agrobiologia, in Seropédica Municipality, RJ, Brazil. For both experiments, treatments consisted of tomato (S. lycopersicum cv. Santa Clara I-5300) plants grown with no inoculation (control) and inoculated with DSE fungi (A101, A104, and A105). All treatments using an inorganic N source received ammonium sulfate-15N as the sole N source, while treatments using organic N source received C. ensiformis-15N. Santa Clara I-5300 is an indeterminate tomato cultivar belonging to the Santa Cruz group, which has been grown in the Brazilian Center-South region since 1940 (Reis Filgueira, 2008). Tomato seeds were washed with 70% alcohol for 3 min and disinfected with 2.5% sodium hypochlorite for 3 min, followed by eight successive washes in sterile distilled water. Then, seeds were pre-germinated in water agar (8 g L−1) at 28°C to select homogenous plants for both experiments.
Tomato seedlings showing 1–2 leaves were inoculated with DSE fungi by root immersion in the mycelial suspension (1% w/v), while control plants only received sterile distilled water. The soil (12 kg) of inoculation treatments was also drenched by the 500 mL suspension (1% w/v) containing the fresh mycelium, while the control group only received sterile distilled water. Pots were watered daily with 500 mL distilled water to maintain soil moisture near field capacity.
Colonization and Pathogenicity Observations
To confirm whether the three DSE fungi colonized the inner roots endophytically, the roots of tomato plants inoculated with A101, A104, and A105 were cleaned and fixed in 50% ethanol. Then the samples were soaked in 2.5% potassium hydroxide (KOH) overnight. Subsequently, roots were acidified with 1% hydrochloric acid overnight at room temperature, followed by staining with 0.01% (w/v) methyl blue (a mixture of 10:9:1 glycerol/distilled water/hydrochloric acid; Phillips and Hayman, 1970). Finally, roots were placed into 50% ethanol to de-stain. Root sections (approximately 3 cm) were placed on slides with glycerin and hyphal structures were viewed with an Axioplan light microscope (Carl Zeiss, Jena, 151 Germany) equipped with an Axiocam MRC5 digital camera (Carl Zeiss). To prepare cross-sections of colonized roots for light microscopy, samples were dehydrated twice in an ethanol series of 70, 90, and 100% for 1 h each. After dehydration, the colonized roots were infiltrated with historesin (Leica, Wetzlar, Germany) and 100% ethanol (1:1, v/v) for 12 h and then with 100% historesin for 24 h before being embedded in historesin. Sections (approximately 5 μm) were obtained using a rotary microtome (Leica) (Vergara et al., 2017). Samples were observed and images were analyzed as described above. Symptoms were evaluated on scale of 0–3 (0: no visible symptoms; 1: light yellowing; 2: yellowing and late growth; 3: wilting or death) (Diene et al., 2013; Mahmoud and Narisawa, 2013).
Measurements
Aboveground dry biomass, plant height, stem diameter, leaf number, and total leaf area (LI-3100C area meter, LI-COR, Nebraska, USA) of tomato plants were measured at 50 DAT in both experiments. Dry shoot tissue (dried at 65°C, 72 h) was ground in a Wiley-type laboratory mill (<40 mesh) followed by a rolling mill to decrease sample grain size (Smith and Um, 1990).
The concentrations of N, P, K, Ca, Mg, Zn, Fe, Mn, and the 15N abundance (atom % 15N excess) were determined in the aboveground tissues. Micronutrient concentrations were quantified in an aqua regia extract (ISO 12914, 2012) by a plasma detector (PerkinElmer® Optima™ 8,300), while macronutrient concentrations were obtained according to Tedesco (1982).
15N abundance was quantified using continuous-flow isotope ratio mass spectrometry (Finnigan DeltaPlus mass spectrometer coupled to the output of a Carlo Erba EA 1108 total C and N analyzer—Finnigan MAT, Bremen, Germany) (Boddey et al., 1994). Macro- and micronutrients content (mg plant−1) were estimated according to equation (1), using the concentration and dry matter (mg plant−1) accumulated by the tomato plants in each treatment.
The fraction (%) and amount (mg plant−1) of N in the plant derived from fertilizer-15N or finely ground C. ensiformis-15N, as well as the recovery efficiency of 15N, were measured using Equations (2–4), according to International Atomic Energy Agency (2001). The atoms % 15N excess was obtained by the difference between 15N abundance in plants and the 15N natural abundance in the air (0.3663% atoms).
The apparent nutrient recovery efficiency of P and K in shoots was calculated according to Baligar and Fageria (1997). Apparent nutrient recovery efficiency reflects the efficiency of plants obtaining nutrients from soil per unit of nutrient applied, as described by Equation (5).
Statistical Analysis
For each experiment, data were individually submitted to tests for homogeneity (Bartlett) and normality (Shapiro-Wilk) of variances. Because N recovery efficiency from the mineral N source did not meet the assumptions of variance analysis, box-cox transformation was applied (Box and Cox, 1964; Venables and Ripley, 2002; Osborne, 2010). Then the data variance was measured (ANOVA). When ANOVA indicated significant differences, means of treatments were separated through the least significant difference (LSD) calculated by the t-test (p < 0.05). The software R-project version R 3.4.1 (R Development Core Team, 2017) with RStudio (version 1.0.153 and the package agricolae; de Mendiburu, 2017) was used for analysis and data are shown as mean ± standard error.
Results
Colonization and Pathogenicity Observations
The three isolates (A101, A104, and A105) (Figures 1A–N) colonized the root tissue of tomato plants with hyphae colonizing epidermis (Figures 1A,B,E,F,J,M,N), cortex (Figures 1C,H,K,L), and forming microsclerotia-like structures (Figures 1A,C,E–N), with no symptoms of apparent disease. In addition, the melanized septate hyphae of A101 (Figure 1B) and A105 (Figures 1J,M) fungi surrounded cells in the epidermis. A105 was also able to surround cells in the cortex (Figure 1K). The three fungi differed in their colonization patterns. While A101 colonized the region between the cortex and vascular bundle (Figure 1D), A104 formed a network in the epidermis connecting several cells (Figure 1E with details in Figure 1F), and A105 formed abundant vesicles in the epidermis (Figure 1M) and cortex (Figure 1K).
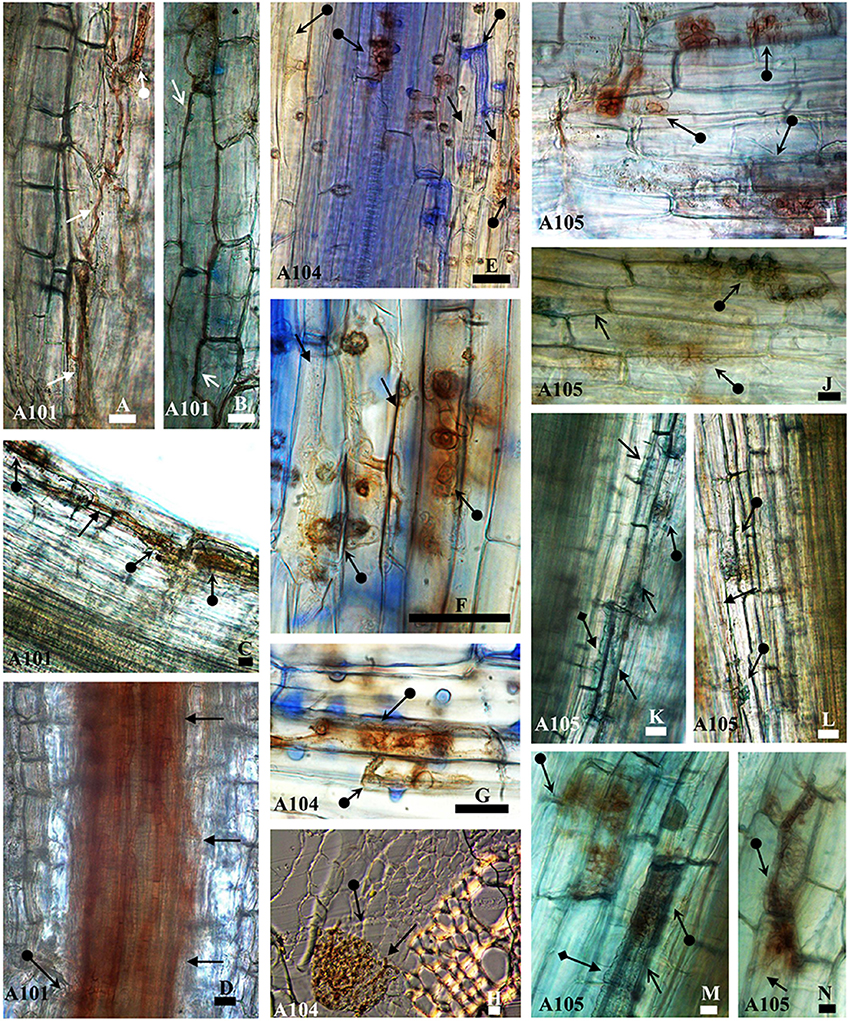
Figure 1. Morphological aspects of tomato roots (Santa Clara I-5300 variety) inoculated with the fungi A101, A104, and A105 at 50 DAT. Melanized septate hyphae surround (arrowheads) or not (arrow) cells in the epidermis (B, J, M, and A, E, F, N, respectively) and in the cortex (K, C, and, H, L, respectively) and in the region between the cortex and vascular bundle (D). Microsclerotia-like structures (arrow with circle at the base) formed by the fungi in the epidermis (A, E–G,I,J,M, and N), in the cortex (C, H, K, L), and in the region between cortex and vascular bundle (D). Hyaline vesicles (arrow with diamond base) formed in the epidermis (M) and the cortex (K). Samples were stained with 0.01% methyl blue, except (H), which was obtained from an unstained cross section. Bar = 20 μm.
DSE Inoculation Effects on Plant Growth
The three DSE isolates (A101, A104, and A105) promoted tomato growth without causing any typical disease symptoms using finely ground C. ensiformis-15N as the organic N source. Tomato plants exhibited significant increases in aboveground dry biomass (25, 34, and 41% increases for A101, A104, and A105, respectively), plant height, and leaf number, relative to the uninoculated treatment (Table 1). A104 stood out from other isolates, because the leaf number and total leaf area of inoculated plants also exhibited significant increases of 50 and 67%, respectively, in comparison to uninoculated control plants (Table 1). However, inoculation had no effect on stem diameter. Likewise, DSE-tomato interaction had no effect on aboveground dry biomass, leaf number, and stem diameter of the tomato plants when inorganic N was supplied as ammonium sulfate-15N (Table 2). However, significant differences were detected for total leaf area, especially in the DSE-tomato interaction compared with control plants (Table 2).
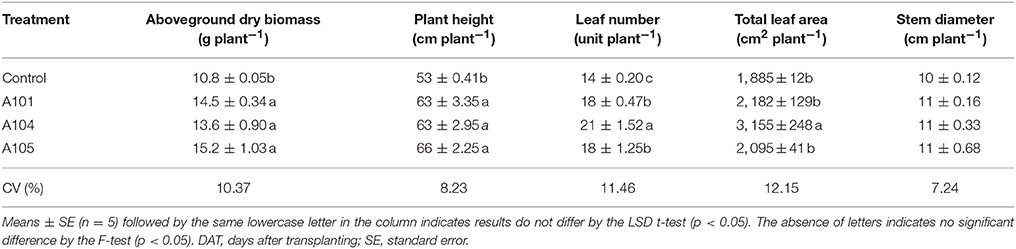
Table 1. Growth indicators of tomato plants at 50 DAT with no plant inoculation (control) and inoculation with dark septate endophyte (DSE) fungi and fertilized with an organic N source (finely ground Canavalia ensiformis [L.]-15N).
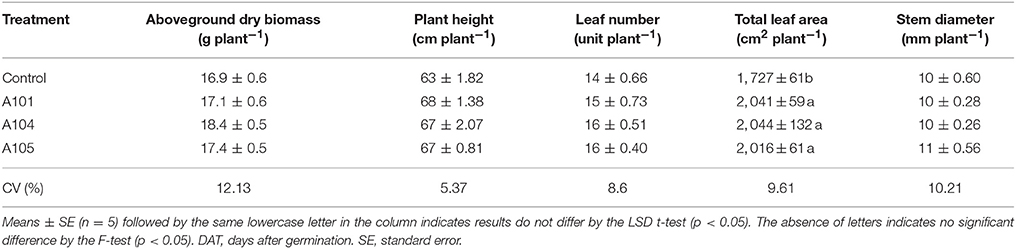
Table 2. Growth indicators of tomato plants at 50 DAT with no plant inoculation (control) and inoculation with DSE fungi and fertilization with an inorganic N source (ammonium sulfate-15N).
Fertilizer-15N, -P, and -K Recovery Efficiency
In general, both uninoculated and inoculated tomato plants recovered N from the inorganic source (ammonium sulfate-15N) more efficiently than from the organic source (finely ground C. ensiformis-15N) (Figures 2A–D). However, the effect of DSE inoculation on 15N recovered was evident (and significant) only when tomato plants were fertilized by finely ground C. ensiformis-15N (Figures 2A–D). The amount of nitrogen in the plant derived from C. ensiformis-15N and the recovery efficiency of 15N from this source significantly increased in the DSE-tomato plants (by 20–30%) compared to the uninoculated control plants (Figures 2C,D). Conversely, tomato plants fertilized with the inorganic source showed no significant differences among the treatments for the amount of nitrogen in the plant derived from fertilizer-15N and for the fertilizer-15N recovery efficiency.
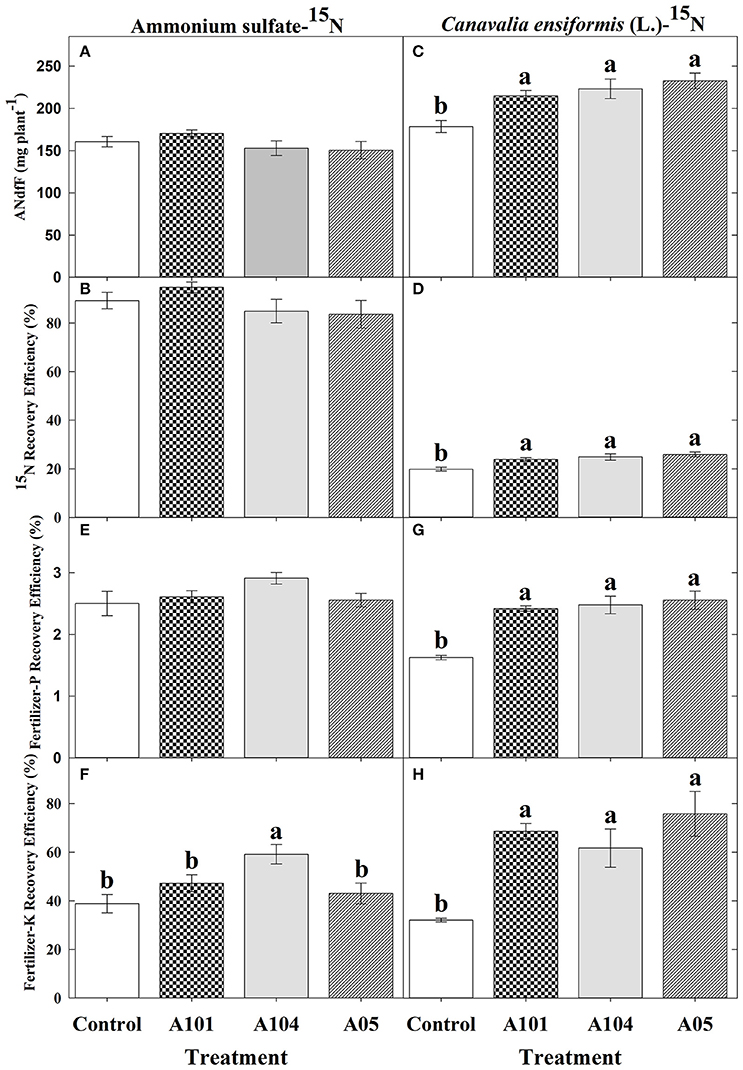
Figure 2. Amount of nitrogen (N) in the plant derived from ammonium sulfate-15N (A) or finely ground C. ensiformis (L.)-15N (C), recovery efficiency of ammonium sulfate-15N (B) or finely ground C. ensiformis-15N (D), apparent recovery efficiency of fertilizer-P [(E) for ammonium sulfate-15N and (G) for C. ensifomis-15N] and -K [(F) for ammonium sulfate-15N and (H) for C. ensifomis-15N] at 50 DAT by tomato (cv. Santa Clara I-5300) plants with no inoculation (control) and inoculated with dark septate endophytic (DSE) fungi A101, A104, and A105, and fertilized with an inorganic (ammonium sulfate-15N) or an organic (finely ground C. ensiformis [L.]-15N) N source. Among treatments, values followed by the same lowercase letter do not differ by LSD t-test (p < 0.05). The absence of letters indicates no significant difference by the F-test (p < 0.05). Error bars represent standard error of mean (n = 5).
In the presence of C. ensiformis-15N, DSE isolates A101, A104, and A105 significantly increased the fertilizer-P and -K apparent recovery efficiency by 49, 52, and 57% and by 114, 92, and 136%, respectively, relative to uninoculated control plants (Figure 2E–H). However, in the presence of ammonium sulfate-15N, only the fertilizer-K apparent recovery efficiency was increased by 52% with the fungus A104.
Macro- and Micronutrients Accumulation
Inoculation treatments did not affect the contents of N, P, Ca, Mg, Fe, Mn, and Zn (Figures 3A,B,D–H) when ammonium sulfate-15N was applied, which corroborates the observed fertilizer-15N (Figure 2B) and -P (Figure 2E) recovery efficiencies. However, inoculation with the fungus A104 led to a significantly higher K accumulation (an increase of about 30%) compared to the control and other treatments (Figure 3C), corroborating the higher fertilizer-K apparent recovery efficiency in this treatment (Figure 2F). On the other hand, DSE-tomato interaction led to a significant accumulation of N, P, K, Ca, Mg, Fe (Figures 4A–F), and Zn (Figure 4H) compared with control plants when organic N source was supplied to tomato plants. Furthermore, inoculation with A105 resulted in higher Mn (Figure 4G) accumulation compared with control plants, but a similar Mn accumulation to the other inoculation treatments. N, P, and K contents increased by 24–33%, 33–39%, and 62–74%, respectively, corroborating the finely ground C. ensiformis-15N (Figure 2D), fertilizer-P (Figure 2G), and -K (Figure 2H) recovery efficiencies. Ca, Fe, Mn, and Zn contents increased by 25–41%, 72–178%, 17–31%, and 41–46%, respectively, while Mg content increased by 30%.
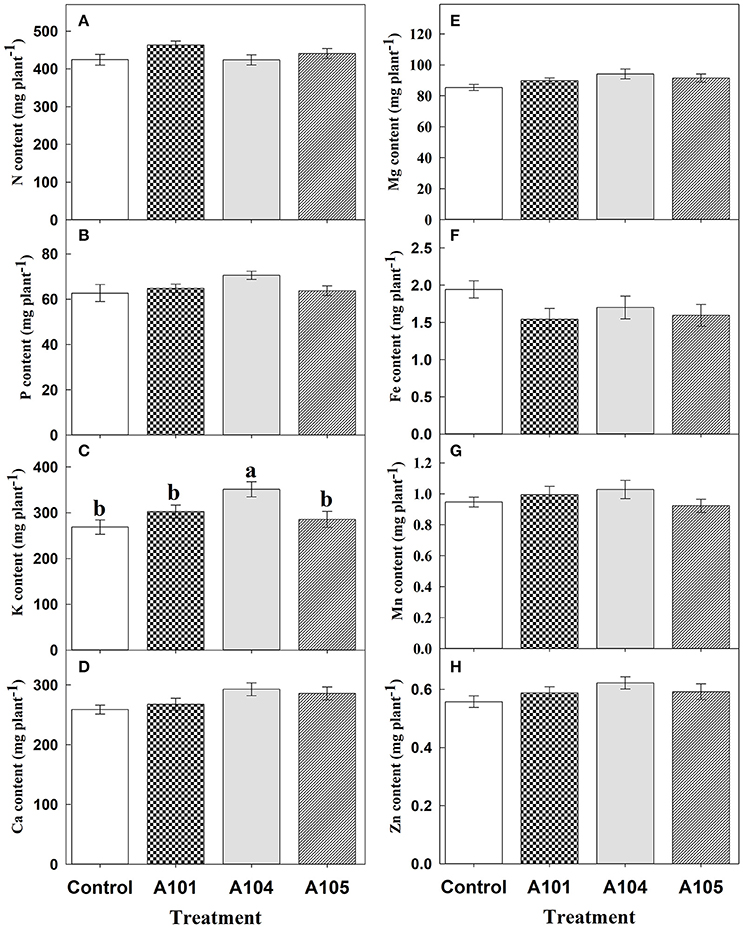
Figure 3. Contents of N (A), P (B), K (C), Ca (D), Mg (E), Fe (F), Mn (G), and Zn (H) at 50 DAT for tomato (cv. Santa Clara I-5300) plants with no inoculation (control) and inoculated with dark septate endophytic (DSE) fungi A101, A104, and A105, and fertilized with inorganic N source (ammonium sulfate-15N). Among the treatments, values followed by the same lowercase letter do not differ by LSD t-test (p < 0.05). The absence of letters indicates no significant difference by the F-test (p < 0.05). Error bars represent standard error of mean (n = 5).
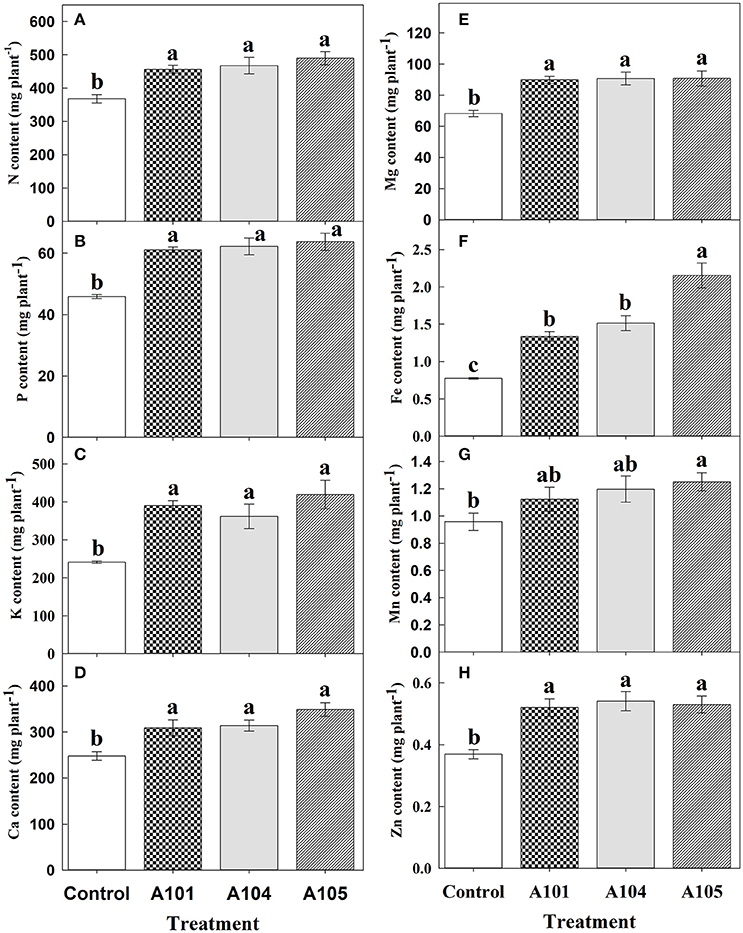
Figure 4. Contents of N (A), P (B), K (C), Ca (D), Mg (E), Fe (F), Mn (G), and Zn (H) at 50 DAT for tomato (cv. Santa Clara I-5300) plants with no inoculation (control) and inoculated with dark septate endophytic (DSE) fungi A101, A104, and A105, and fertilized with organic N source (finely ground Canavalia ensiformis [L.]-15N). Among the treatments, values followed by the same lowercase letter do not differ by LSD t-test (p < 0.05). Error bars represent standard error of mean (n = 5).
Discussion
The DSE fungi tested in this study were obtained from wild rice (O. glumaepatula) collected from the Amazon region and identified by the ITS phylogeny to belong to order Pleosporales (suborder Massarineae) (A104 and A105) and an unknown taxon (A101) (Ribeiro, 2011; Vergara et al., 2017). DSE fungi were able to colonize the roots of tomato plants with no negative symptoms, even when the hyphae colonized the epidermis and the cortex of root plants and formed microsclerotia in the roots cells. Other studies have also reported colonization by DSE fungi in epidermis and cortex cells of tomato plants with no symptoms of pathologies (Andrade-Linares et al., 2011a; Mahmoud and Narisawa, 2013).
Similar to previous works on plant responses to inoculation with DSE fungi (Usuki and Narisawa, 2007; Diene et al., 2013; Mahmoud and Narisawa, 2013; Qin et al., 2017), the present study showed that inoculation with DSE fungi led to an increase in aboveground dry biomass of tomato plants supplied with an organic N source (finely ground C. ensiformis-15N). The same effect was not observed in plants treated with an inorganic N source (ammonium sulfate-15N). Conversely, Andrade-Linares et al. (2011b) reported that the DSE fungus Leptodontidium orchidicola was capable of increasing the shoot dry matter of tomato plants, as well as the fruit mass and glucose content, even in plants grown with an inorganic N source (NH4).
A meta-analysis was performed from 18 studies carried out independently and confirmed that plants inoculated with carefully-selected DSE fungi responded positively when supplied with organic N sources, showing increases in dry matter and contents of N and P ranging from 26 to 106% (Newsham, 2011). Similarly, in the present study, which was carried out under greenhouse and non-sterile soil conditions, the DSE-tomato interaction was more efficient with use of N from an organic source. Increases were observed in the amount of nitrogen in the plant derived from finely ground C. ensiformis-15N, in the recovery efficiency of 15N, P, and K, in the contents of N, P, K, Ca, and Mg, plant height, leaf number, and total leaf area (A104), leading to increases in aboveground dry biomass ranging from 25 to 41% in comparison with control plants. Such results indicate that the DSE fungi A101, A104, and A105 can enhance tomato plant nutrition and growth when fertilized with an organic N source. Conversely, plants supplied with an inorganic N source only showed a positive inoculation effect for fertilizer-K recovery efficiency by plants, K content, and leaf area (A104), while other evaluated variables showed no effect. This suggested that the cultivar Santa Clara I-5300 does not need the fungus to absorb readily assimilable N as N-, since breeding programs have frequently selected for plants more responsive to mineral fertilization (Borlaug and Dowswell, 1997). In a previous study performed under controlled hydroponic conditions, significant increases were not also observed in shoot N content using inorganic N sources (Torres-Júnior, 2014), although experimental conditions were different from those of the present study.
The ability of DSE fungi to promote growth and increase nutrients contents in tomato plants fertilized with finely ground C. ensiformis-15N suggests that these fungi are capable of degrading organic C, N, and P compounds, increasing macro and micronutrient availabilities to plants. Indeed, studies have reported that DSE fungi degrade organic compounds including cellulose, starch, proteins, lipids, amino acids, gelatin, urea, and pectin under in vitro conditions (Caldwell et al., 2000; Menkis et al., 2004; Mandyam et al., 2010; Surono and Narisawa, 2017). Additionally, DSE fungi promote the growth of several plant species supplied only with an organic N source (amino acids) or organic P source (phytate) under in vitro conditions (Usuki and Narisawa, 2007; Diene et al., 2013; Mahmoud and Narisawa, 2013; Surono and Narisawa, 2017). Recently, three isolates of Phialocephala fortinii led to the growth of Asparagus officinalis (L.) in agar medium supplied only with corn steep liquor (0.1%) or with corn steep liquor amended with inorganic nutrients (Surono and Narisawa, 2017). Although the preference in degrading a specific organic nutrient source can differ among species of DSE fungi (Diene et al., 2013; Surono and Narisawa, 2017), studies have suggested that DSE fungi are capable of mineralizing organic compounds containing N and P, making them available to plants. A recent study demonstrated that DSE fungi are capable of mineralizing organic P compounds under in vitro conditions (Della Monica et al., 2015). However, the mechanisms leading to an increase in the growth of plants inoculated with DSE fungi still need further research.
The highest K content and fertilized-K apparent recovery efficiency was observed for fertilization with ammonium sulfate-15N (for the fungus A104) and with finely ground C. ensiformis-15N (in all inoculation treatments), indicating that the DSE-tomato interaction was more responsive to K compare to other evaluated nutrients. This may be because K is the most required cation for plants and the most abundant cation in cytosol and plant dry matter (Marschner, 1995; Meurer, 2006). K acts in several physiological processes in plants, including photosynthesis, activation of more than 60 enzymatic systems, regulation of stomatal opening and closure, cell growth and elongation by generating cell turgor, and protein synthesis (Marschner, 1995).
During uptake K is transported from the soil solution to the root surface mainly by diffusion. Mass flow can significantly contribute to transport when the K concentration is high in the soil solution (Barber, 1995; Ruiz et al., 1999). However, diffusion is limited to very short distances from the root surface, usually around 1–4 mm (Meurer, 2006; Zeiger et al., 2017). Thus, the high K content and fertilizer-K apparent recovery efficiency observed under inoculation treatments strongly indicated that these fungi helped tomato plants absorb K through transposition of the K depletion zone and K interception in remote locations unattainable by the root surface.
Higher K and N contents are directly related to a greater total leaf area, since K acts on cell extension and N acts on leaf elongation, leading to a greater leaf area (Meiri et al., 1992; Chapman and Lemaire, 1993; Neto et al., 2007) and thus to greater plant exposition to sunlight (Neto et al., 2007). Contrary to previous studies (Melin, 1922; Wilcox and Wang, 1987; Stoyke and Currah, 1993; Mandyam et al., 2010; Diene et al., 2013), negative effects of inoculation with DSE fungi on plant growth were not observed.
Dark septate endophytic (DSE) fungi can also facilitate the uptake of micronutrients, including Fe, present in the soil (Bartholdy et al., 2001; Haselwandter, 2009). In this study, inoculation led to an increase in Fe content from 72 to 178%, and in Mn and Zn contents from 17–25% to 41–46%, respectively. These increases in Fe, Mn, and Zn contents under inoculation are related to a better use of these micronutrients in their source (soil, FTE BR12, and C. ensifomis-15N). Bartholdy et al. (2001) observed that DSE Phialocephala fortinii synthesized siderophore hydroxamate which led to an increase in Fe (III) uptake by host plants. When absorbed by plants, Fe acts on redox reactions in hemoproteins, such as cytochromes, and in non-heme proteins, such as ferredoxin (Dechen and Nachtigall, 2006). Ferredoxin and cytochromes are carriers of electrons during photosynthesis (Dechen and Nachtigall, 2006). Zn and Mn also act on enzymes involved in C metabolism. For instance, Zn is a structural component of carbonic anhydrase, which catalyzes CO2 dissolution (previous to its assimilation), and activates triphosphate dehydrogenase, an essential enzyme for glycolysis (Dechen and Nachtigall, 2006). Mn acts on photosystem II (in the Mn complex), which is responsible for water photolysis (Broadley et al., 2012). Currently, Mn has been recognized as an indicator of P acquisition efficiency by plants (Lambers et al., 2015). Thus, an increase in Fe, Mn, and Zn contents corroborates previous studies (Zhang et al., 2012, 2017; Ban et al., 2017) which suggested involvement of DSE fungi in photosynthetic activity by increasing chlorophyll levels, photochemical efficiency of photosystem II, net photosynthetic rate, stomatal conductance, and transpiration rate, leading to higher glucose (Andrade-Linares et al., 2011b) and soluble sugar contents (Vergara et al., 2017).
In general, tomato plants fertilized with finely ground C. ensiformis-15N showed significant differences among inoculation treatments, especially fungus A104, which had greater leaf number and area, and fungus A105, which had a higher Fe content. On the other hand, plants treated with ammonium sulfate-15N showed significant differences for K content and fertilizer-K apparent recovery efficiency, with an emphasis on fungus A104. These differences suggest that plant responses to inoculation with DSE depend on each fungus isolate, as it has already been observed in AMF inoculation (Mensah et al., 2015).
Conclusion
Our findings indicated that tomato plants inoculated with DSE fungi acquired macro and micronutrients more efficiently, especially when an organic N source is used, resulting in increased plant growth. This finding was demonstrated using finely ground 15N-labeled C. ensiformis or ammonium sulfate-15N applied to soil. The A104 isolate seems to be the best option for inoculation of tomato cv. Santa Clara I-5300. However, a more detailed understanding of biochemical and molecular DSE-tomato interactions is still needed.
Author Contributions
CV, KA, SU, NS, FB, PM, LS, and JZ designed, performed experiments and analyzed data. CV, KA, FB, GX, and JZ conceived the experiments and wrote the paper. All authors read, edited, and approved the final manuscript.
Funding
Empresa Brasileira de Pesquisa Agropecuária. Coordenação de Aperfeiçoamento de Pessoal de Nível Superior. Conselho Nacional de Desenvolvimento Científico e Tecnológico. Fundação Carlos Chagas Filho de Amparo à Pesquisa do Estado do Rio de Janeiro.
Conflict of Interest Statement
The authors declare that the research was conducted in the absence of any commercial or financial relationships that could be construed as a potential conflict of interest.
Acknowledgments
We would like to thank the University Federal Rural do Rio de Janeiro (UFRRJ), the Brazilian Agricultural Research Corporation (Embrapa), the Foundation for Support of Research in the State of Rio de Janeiro (FAPERJ), the Brazilian National Council for Scientific and Technological Development (CNPq) and the Coordination for the Improvement of Higher Education Personnel (CAPES) for economical support. We also thank Dr. Ednaldo Araújo for kindly have provided the material of C. ensiformis enriched with 15N.
References
Andrade-Linares, D. R., Grosch, R., Franken, P., Rexer, K. H., Kost, G., Restrepo, S., et al. (2011a). Colonization of roots of cultivated Solanum lycopersicum by dark septate and other ascomycetous endophytes. Mycologia 103, 710–721. doi: 10.3852/10-329
Andrade-Linares, D. R., Grosch, R., Restrepo, S., Krumbein, A., and Franken, P. (2011b). Effects of dark septate endophytes on tomato plant performance. Mycorrhiza 21, 413–422. doi: 10.1007/s00572-010-0351-1
Baligar, V. C., and Fageria, N. K. (1997). “Nutrient use efficiency in acid soils: nutriente management and plant use efficiency,” in Plant-Soil Interactions at low pH: Sustainable Agricultural and Forestry Production, ed A. C. Muniz (Campinas: Brazilian Soil Science Society), 75–93.
Ban, Y., Xu, Z., Yang, Y., Zhang, H., Chen, H., and Tang, M. (2017). Effect of dark septate endophytic fungus Gaeumannomyces cylindrosporus on plant growth, photosynthesis and Pb tolerance of maize (Zea mays L.). Pedosphere 27, 283–292. doi: 10.1016/S1002-0160(17)60316-3
Barber, (1995). Soil Nutrient Bioavailability. A Mechanistic Approach. New York, NY: Wiley and Sons.
Bartholdy, B., Berreck, M., and Haselwandter, K. (2001). Hydroxamate siderophore synthesis by Phialocephala fortinii, a typical dark septate fungal root endophyte. BioMetals 14, 33–42. doi: 10.1023/A:1016687021803
Block, G., Patterson, B., and Subar, A. (1992). Fruit, vegetables, and cancer prevention: a review of the epidemiological evidence. Nutr. Cancer 18, 1–29. doi: 10.1080/01635589209514201
Boddey, R. M., Alves, B. J. R., and Urquiaga, S. (1994). “Quantificação da fixação biológica de nitrogênio associada a plantas utilizando o isótopo 15N,” in Manual de Métodos Empregados em Estudos de Microbiologia Agrícola, Vol. 46, eds M. Hungria and R. S. Araujo (Brasília: Embrapa-SPI, Documentos), 471–494.
Borlaug, N. E., and Dowswell, C. R. (1997). “The acid lands: one of agriculture's last frontiers,” in Plant-Soil Interactions at Low pH: Sustainable Agriculture and Forestry Production, eds A. C. Moniz, A. M. C. Furlani, R. E. Schaffert, N. K. Fageria, C. A. Rosolem, and H. Cantarella (Campinas, SP: Brazilian Soil Science Society), 5–15.
Box, G. E., and Cox, D. R. (1964). An analysis of transformations. J. R. Stat. Soc. Series B 26, 211–252.
Broadley, M., Brown, P., Cakmak, I., Rengel, Z., and Zhao, F. (2012). “Function of nutrients: micronutrients,” in Marschner's Mineral Nutrition of Higher Plants, 3 Edn., ed H. Marschner (Cambridge, MA: Academic Press; Elsevier), 191–248.
Caldwell, B. A., Jumpponen, A., and Trappe, J. M. (2000). Utilization of major detrital substrates by dark-septate, root endophytes. Mycologia 92, 230–230. doi: 10.2307/3761555
Cavagnaro, T. R., Bender, S. F., Asghari, H. R., and van der Heijden, M. G. A. (2015). The role of arbuscular mycorrhizas in reducing soil nutrient loss. Trends Plant Sci. 20, 283–290. doi: 10.1016/j.tplants.2015.03.004
Chapman, D. F., and Lemaire, G. (1993). “Morphogenetic and structural determinants of plant regrowth after defoliation,” in Proceedings of the XVII International Grassland Congress, Palmerston North, Hamilton, Lincoln and Rockhampton (Palmerston North, NZ: The Association), 95–104.
de Mendiburu, F. (2017). Agricolae: Statistical Procedures for Agricultural Research, R Package version 1.,2-6. Lima-PERU. Available online at: https://CRAN.R-project.org/package=agricolae
Dechen, A. R., and Nachtigall, G. R. (2006). “Micronutrientes,” in Nutrição Mineral de Plantas, 1st Edn., ed M. S. Fernandes (Viçosa: Sociedade Brasileira de Ciência do Solo), 253–280.
Della Monica, I. F., Saparrat, M. C. N., Godeas, A. M., and Scervino, J. M. (2015). The co-existence between DSE and AMF symbionts affects plant P pools through P mineralization and solubilization processes. Fungal Ecol. 17, 10–17. doi: 10.1016/j.funeco.2015.04.004
Diene, O., Wang, W., and Narisawa, K. (2013). Pseudosigmoidea ibarakiensis sp. nov., a dark septate endophytic fungus from a cedar forest in Ibaraki, Japan. Microbes Environ. 28, 381–387. doi: 10.1264/jsme2.ME13002
Finlay, R., and Söderström, B. (1992). “Mycorrhiza and carbon flow to the soil”, in Mycorrhizal Functioning: An Integrative Plant-Fungal Process, ed M. Allen (New York, NY: Springer), 134–160.
Foolad, M. (2004). Recent advances in genetics of salt tolerance in tomato. Plant Cell Tissue Organ Cult. 76, 101–119. doi: 10.1023/B:TICU.0000007308.47608.88
Gerster, H. (1997). The potential role of lycopene for human health. J. Am. Coll. Nutr. 16, 109–126. doi: 10.1080/07315724.1997.10718661
Haselwandter, K. (2009). Mycorrhizal fungi: colonisation pattern of alpine plants and ecological significance of siderophore release. Aspects Appl. Biol. 98, 105–108.
He, X., and Nara, K. (2007). Element biofortification: can mycorrhizas potentially offer a more effective and sustainable pathway to curb human malnutrition? Trends Plant Sci. 12, 331–333. doi: 10.1016/j.tplants.2007.06.008
Hodge, A., Campbell, C. D., and Fitter, A. H. (2001). An arbuscular mycorrhizal fungus accelerates decomposition and acquires nitrogen directly from organic material. Nature 413, 297–299. doi: 10.1038/35095041
Hodge, A., and Fitter, A. H. (2010). Substantial nitrogen acquisition by arbuscular mycorrhizal fungi from organic material has implications for N cycling. Proc. Natl. Acad. Sci. U.S.A. 107, 13754–13759. doi: 10.1073/pnas.1005874107
Hogberg, P. (1989). “Root symbioses of trees in savannas,” in Mineral Nutrients in Tropical Forest and Savanna Ecosystems. Special Publication of the British Ecological Society, Vol. 9, ed J. Proctor (Oxford, UK: Blackwell Scientific Publishing House), 121–136.
International Atomic Energy Agency (2001). Training Course Series No 14. Use of Isotope and Radiation Methods in Soil and Water Management and Crop Nutrition. Vienna: IAEA.
ISO 12914 (2012). Soil Quality-Microwave-Assisted Extraction of the Aqua Regia Soluble Fraction for the Determination of Elements. Berlin.
Jumpponen, A., and Trappe, J. M. (1998). Dark septate endophytes: a review of facultative biotrophic root-colonizing fungi. New Phytol. 140, 295–310. doi: 10.1046/j.1469-8137.1998.00265.x
Lambers, H., Hayes, P. E., Laliberté, E., Oliveira, R. S., and Turner, B. L. (2015). Leaf manganese accumulation and phosphorus-acquisition efficiency. Trends Plant Sci. 20, 83–90. doi: 10.1016/j.tplants.2014.10.007
Macebo, J. R. D., Gouveia, R. F. D., Bhering, S. B., Freire, L. R., Lima, E., Zonta, E., et al. (2013). “Recomendações de adubos, corretivos e de manejo da matéria orgânica para as principais culturas do Estado do Rio de Janeiro,” in Manual de Calagem e Adubação do Estado do Rio de Janeiro 1st Edn., eds L. R. Freire, F. d. C. Balieiro, E. Zonta, L. H. C. d. Anjos, M. G. Pereira, E. Lima et al. (Brasília: Editora Universidade Rural), 430.
Mahmoud, R. S., and Narisawa, K. (2013). A new fungal endophyte, Scolecobasidium humicola, promotes tomato growth under organic nitrogen conditions. PLoS ONE 8:e78746. doi: 10.1371/journal.pone.0078746
Mandyam, K. (2008). Dark Septate Fungal Endophytes From a Tallgrass Prairie and their Continumum of Interactions with Host Plants. Kansas State University.
Mandyam, K., and Jumpponen, A. (2005). Abundance and possible functions of the root-colonizing dark septate endophytic fungi. Stud. Mycol. 53, 173–190. doi: 10.3114/sim.53.1.173
Mandyam, K., Loughin, T., and Jumpponen, A. (2010). Isolation and morphological and metabolic characterization of common endophytes in annually burned tallgrass prairie. Mycologia 102, 813–821. doi: 10.3852/09-212
Meiri, A., Silk, W. K., and Läuchli, A. (1992). Growth and deposition of inorganic nutrient elements in developing leaves of Zea mays L. Plant Physiol. 99, 972–978. doi: 10.1104/pp.99.3.972
Melin, E. (1922). On the mycorrhizas of Pinus sylvestris L. and Picea abies Karst. A preliminary note. J. Ecol. 9, 254–257.
Menkis, A., Allmer, J., Vasiliauskas, R., Lygis, V., Stenlid, J., and Finlay, R. (2004). Ecology and molecular characterization of dark septate fungi from roots, living stems, coarse and fine woody debris. Mycol. Res. 108, 965–973. doi: 10.1017/S0953756204000668
Mensah, J. A., Koch, A. M., Antunes, P. M., Kiers, E. T., Hart, M., and Bücking, H. (2015). High functional diversity within species of arbuscular mycorrhizal fungi is associated with differences in phosphate and nitrogen uptake and fungal phosphate metabolism. Mycorrhiza 25, 533–546. doi: 10.1007/s00572-015-0631-x
Meurer, E. J. (2006). “Potássio,” in Nutrição Mineral de Plantas 1st Edn., ed M. S. Fernandes (Viçosa: Sociedade Brasileira de Ciência do Solo), 282–295.
Surono and Narisawa, K. (2017). The dark septate endophytic fungus Phialocephala fortinii is a potential decomposer of soil organic compounds and a promoter of Asparagus officinalis growth. Fungal Ecol. 28, 1–10. doi: 10.1016/j.funeco.2017.04.001
Neto, D. C., Monteiro, F. A., and Dechen, A. R. (2007). Características produtivas do capim-tanzânia cultivado com combinações de potássio e de magnésio. Acta Scientiarum. Agronomy 29, 459–467. doi: 10.4025/actasciagron.v29i4.399
Newsham, K. K. (2011). A meta-analysis of plant responses to dark septate root endophytes. New Phytol. 190, 783–793. doi: 10.1111/j.1469-8137.2010.03611.x
Osborne, J. W. (2010). Improving your data transformations: applying the box-cox transformation. Pract Assess. Res. Eval. 15, 1–9.
Perin, A., Guerra, J. G. M., and Teixeira, M. G. (2003). Cobertura do solo e acumulação de nutrientes pelo amendoim forrageiro. Pesquisa Agropecuária Brasileira 38, 791–796. doi: 10.1590/S0100-204X2003000700002
Phillips, J. M., and Hayman, D. S. (1970). Improved procedures for clearing roots and staining parasitic and vesicular-arbuscular mycorrhizal fungi for rapid assessment of infection. T Brit. Mycol. Soc. 55, 157–160. doi: 10.1016/S0007-1536(70)80110-3
Qin, Y., Pan, X., Kubicek, C., Druzhinina, I., Chenthamara, K., Labbé, J., et al. (2017). Diverse plant-associated pleosporalean fungi from saline areas: ecological tolerance and nitrogen-status dependent effects on plant growth. Front. Microbiol. 8:158. doi: 10.3389/fmicb2017.00158
R Development Core Team (2017). R: A Language and Environment for Statistical Computing. R Foundation for Statistical Computing. Vienna. Available online at: https://www.r-project.org/
Rao, A. V., and Agarwal, S. (2000). Role of antioxidant lycopene in cancer and heart disease. J. Am. College Nutr. 19, 563–569. doi: 10.1080/07315724.2000.10718953
Reeve, J. R., Smith, J. L., Carpenter-Boggs, L., and Reganold, J. P. (2008). Soil-based cycling and differential uptake of amino acids by three species of strawberry (Fragaria spp.) plants. Soil Biol. Biochem. 40, 2547–2552. doi: 10.1016/j.soilbio.2008.06.015
Reis Filgueira, F. A. (2008). Novo Manual de Olericultura: Agrotecnologia Moderna na Produção e Comercialização de Hortaliças. Viçosa, MG: Editora UFV.
Ribeiro, K. G. (2011). Fungos Endofíticos dark Septates em Arroz Silvestre Oryza glumaepatula Steund. Dissertation, Universidade Federal de Roraima.
Rodrigues, J., Alves, R., Lopes, O., Teixeira, R., and Rosa, E. (2004). A Importância do feijão de porco (Canavalia ensiformis DC) como cultura intercalar em rotação com milho e feijão caupi em cultivo de coqueirais no município de Ponta-de-Pedras/Marajó-PA. Embrapa Amazônia Oriental-Comunicado Técnico (Belém, PA: INFOTECA-E).
Ruiz, H. A., Miranda, J., and Conceição, J. C. S. (1999). Contribuição dos mecanismos de fluxo de massa e de difusão para o suprimento de K, Ca e Mg a plantas de arroz. Rev. Brasileira de Ciência do Solo 23, 1015–1018. doi: 10.1590/S0100-06831999000400029
Smith, J., and Um, M. H. (1990). Rapid procedures for preparing soil and KCl extracts for 15N analysis. Commun. Soil Sci. Plant Analysis 21, 2173–2179. doi: 10.1080/00103629009368368
Stoyke, G., and Currah, R. S. (1993). Resynthesis in pure culture of a common subalpine fungus-root association using Phialocephala fortinii and Menziesia ferruginea (Ericaceae). Arctic Alpine Res. 25, 189–189. doi: 10.2307/1551812
Sylvia, D. M., and Jarstfer, A. G. (1994). “Production of inoculum and inoculation with arbuscular Mycorrhizal fungi,” in Management of Mycorrhizas in Agriculture, Horticulture and Forestry, eds A. D. Robson, L. K. Abbott, and N. Malajczuk (Netherlands: Kluwer), 231–238.
Tedesco, M. J. (1982). Extração simultânea de N, P, K, Ca, e Mg em tecido de plantas por disgestão com H2O2-H2SO4. Porto Alegre, RS: UFRGS.
Tejada, M., Gonzalez, J., García-Martínez, A., and Parrado, J. (2008). Effects of different green manures on soil biological properties and maize yield. Bioresour. Technol. 99, 1758–1767. doi: 10.1016/j.biortech.2007.03.052
Torres-Júnior, C. V. (2014). Influência dos Fungos dark Septate Sobre Absorção de Nutrientes e Crescimento de Plantas de Arroz e tomate. Seropédica, RJ: Universidade Federal Rural de Rio de Janeiro.
Upson, R., Read, D. J., and Newsham, K. K. (2009). Nitrogen form influences the response of Deschampsia antarctica to dark septate root endophytes. Mycorrhiza 20, 1–11. doi: 10.1007/s00572-009-0260-3
Usuki, F., and Narisawa, K. (2007). A mutualistic symbiosis between a dark septate endophytic fungus, Heteroconium chaetospira, and a nonmycorrhizal plant, Chinese cabbage. Mycologia 99, 175–184. doi: 10.1080/15572536.2007.11832577
Venables, W., and Ripley, B. (2002). Modern Applied Statistics with S, 4th Edn. New York, NY: Springer-Verlag. 498.
Vergara, C., Araujo, K. E. C., Alves, L. S., de Souza, S. R., Santos, L. A., Santa-Catarina, C., et al. (2017). Contribution of dark septate fungi to the nutrient uptake and growth of rice plants. Braz. J. Microbiol. doi: 10.1016/j.bjm.2017.04.010. [Epub ahead of print].
Viuda-Martos, M., Sanchez-Zapata, E., Sayas-Barberá, E., Sendra, E., Pérez-Álvarez, J. A., and Fernández-López, J. (2014). Tomato and tomato byproducts. Human health benefits of lycopene and its application to meat products: a review. Crit. Rev. Food Sci. Nutr. 54, 1032–1049. doi: 10.1080/10408398.2011.623799
Wilcox, H. E., and Wang, C. J. K. (1987). Mycorrhizal and pathological associations of dematiaceous fungi in roots of 7-month-old tree seedlings. Can. J. Forest Res. 17, 884–899. doi: 10.1139/x87-140
Yuan, Z. L., Su, Z. Z., Mao, L. -J., Peng, Y.-Q., Yang, G. M., Lin, F. -C., et al. (2011). Distinctive endophytic fungal assemblage in stems of wild rice (Oryza granulata) in China with special reference to two species of Muscodor (Xylariaceae). J. Microbiol. 49, 15–23. doi: 10.1007/s12275-011-0213-3
Zeiger, E., Møller, I. M., Murphy, A., and Taiz, L. (2017). Fisiologia e Desenvolvimento Vegetal. Porto Alegre: Artmed.
Zhang, H. -H., Tang, M., Chen, H., and Wang, Y.-J. (2012). Effects of a dark-septate endophytic isolate LBF-2 on the medicinal plant Lycium barbarum L. J. Microbiol. 50, 91–96. doi: 10.1007/s12275-012-1159-9
Keywords: Solanum lycopersicum (L.), 15N, DSE fungi, nutrient recovery efficiency, potassium, phosphorus, Canavalia ensiformis (L.) -15N
Citation: Vergara C, Araujo KEC, Urquiaga S, Schultz N, Balieiro FC, Medeiros PS, Santos LA, Xavier GR and Zilli JE (2017) Dark Septate Endophytic Fungi Help Tomato to Acquire Nutrients from Ground Plant Material. Front. Microbiol. 8:2437. doi: 10.3389/fmicb.2017.02437
Received: 01 September 2017; Accepted: 23 November 2017;
Published: 11 December 2017.
Edited by:
Brigitte Mauch-Mani, University of Neuchâtel, SwitzerlandReviewed by:
Gwen-Aelle Grelet, Landcare Research, New ZealandMiroslav Vosatka, Institute of Botany (ASCR), Czechia
Copyright © 2017 Vergara, Araujo, Urquiaga, Schultz, Balieiro, Medeiros, Santos, Xavier and Zilli. This is an open-access article distributed under the terms of the Creative Commons Attribution License (CC BY). The use, distribution or reproduction in other forums is permitted, provided the original author(s) or licensor are credited and that the original publication in this journal is cited, in accordance with accepted academic practice. No use, distribution or reproduction is permitted which does not comply with these terms.
*Correspondence: Jerri E. Zilli, jerri.zilli@embrapa.br