- Department of Entomology and Plant Pathology, College of Aburaihan, University of Tehran, Tehran, Iran
Endophytic fungi are microorganisms with the ability to colonize plants for the entire or at least a significant part of their life cycle asymptomatically, establishing a plant-fungus association. They play an important role in balancing ecosystems, as well as benefiting host through increasing plant growth, and protecting the host plants from abiotic and biotic stresses using various strategies. In the present study, endophytic fungi were isolated from wild and endemic apple cultivars, followed by characterizing their antifungal effect against Venturia inaequalis. To characterize the endophytic fungi, 417 fungal strains were separated from 210 healthy fruit, leaf, and branch samples collected from the north of Iran. Among the purified fungal isolates, 33 fungal genera were identified based on the morphological characteristics, of which 38 species were detected according to the morphological features and molecular data of ITS, tef-1α, and gapdh genomic regions (related to the genus). The results represented that most of the endophytic fungi belonged to Ascomycota (67.8%), 31.4% of isolates were mycelia sterilia, while the others were Basidiomycota (0.48%) and Mucoromycota (0.24%). Additionally, Alternaria, Cladosporium, and Nigrospora were determined as the dominant genera. The antifungal properties of the identified isolates were evaluated against V. inaequalis in vitro to determine the release of media-permeable metabolites, Volatile Organic Compounds (VOCs), chitinase, and cellulase as antifungal mechanisms, as well as producing phosphate solubilisation as growth-promoting effect. Based on the results of metabolite and VOC tests, the six isolates of Acremonium sclerotigenum GO13S1, Coniochaeta endophytica 55S2, Fusarium lateritium 61S2, Aureobasidium microstictum 7F2, Chaetomium globosum 2S1 and Ch. globosum 3 L2 were selected for greenhouse tests. Further, Co. endophytica 55S2 and F. lateritium 61S2 could solubilize inorganic phosphate. All isolates except Ch. globosum 3 L2 exhibited cellulase activity, while chitinase activity was observed in Ch. globosum 2S1, Ch. globosum 3 L2, and F. lateritium 61S2. Finally, Co. endophytica 55S2 and Ch. globosum 2S1 completely controlled the disease on the apple seedling leaves under greenhouse conditions.
Introduction
Apple (Malus sp.), which belongs to the Rosaceae family, is considered as the most common and culturally important fruit crop worldwide, as well as one of the superior crops in Iran due to its nutritional and export value (Ebrahimi et al., 2016). The apple scab caused by Venturia inaequalis (Cooke) G. Winter is among the most main diseases in the apple-growing regions across the globe (Tenzer and Gessler, 1997), especially the areas with cool and wet spring, as well as early summer (MacHardy, 1996). The management of this disease is often based on the repeated fungicide application which is expensive and time-consuming. However, some fungicides may lose their efficacy following the development of resistance in the fungus causing apple scab. Nevertheless, the need for nonchemical control methods to reduce the crop losses is becoming increasingly important for protecting the environment and human health (Wenneker and Thomma, 2020). In this regard, a global trend tries to explore the new alternatives to synthetic fungicides, which minimize the risks associated with the development of the populations insensitive to the chemical compounds and are consistent with the food safety standards (Chen et al., 2019). Biological control is an excellent and effective alternative way to control plant diseases (Zhang et al., 2018). In addition, endophytes are a potential source of biological control agents, which are already adapted to live and persist in the plant with minimal adverse effects.
The endophytes are the microorganisms living inside plant tissues for the entire or at least a large part of their life cycle without causing any symptom or adverse harm to the host (Petrini, 1991; Saikkonen et al., 1998). The endophytic fungi can be considered as potential biological competitors since they have evolved to exploit the same resources as plant pathogens (Silva et al., 2018). They contribute to plant health by producing protective metabolites, inducing biotic and abiotic stress resistance in host plants, and improving their growth with phytohormones (Rai et al., 2014; Terhonen et al., 2019). The microorganisms can potentially protect plants from pathogenic fungi through a diverse array of modes of action such as direct inhibition via competition, antibiosis or mycoparasitism, and indirect inhibition by induced resistance (Latz et al., 2018). Recently, endophytic fungi stood out as the most common group of microorganisms elaborated in the laboratory to produce a variety of secondary metabolites such as alkaloids, flavonoids, terpenoids, volatile organic compounds (VOC), phenols and its derivatives, and different enzymes (Zhang et al., 2006; Strobel, 2018) with potential use in industrial application or as fungicides to control the growth of phytopathogenic fungi (Meenavalli et al., 2011; Toghueo et al., 2017). These microorganisms inside the plant are able to degrade a portion of plant lignin and cellulose (Li et al., 2021) which helps host plants to protect themselves against invasive pathogens (Marques et al., 2018). Also, endophytes with the ability to secrete extracellular chitinase would decompose chitin, a β-(1,4)-linked polymer of N-acetyl-D-glucosamine, and the cell wall structure of most phytopathogenic fungi along with other versatile bioactive compounds (Hartl et al., 2012). Endophytes contribute to several processes related to plant growth and development similar to the rhizospheric microbes, such as, nitrogen fixation, phosphate solubilization, etc. (Santoyo et al., 2016). Inorganic phosphate solubilization, through microorganisms, is one of the major mechanisms involved in plant growth (Adhikari and Pandey, 2019). Solubilization of inorganic insoluble phosphate salts by different microorganisms depends on their ability to produce organic acids in the respective environment. These organic acids decrease the pH of the soil or any medium, providing the facility to exchange the metal part of insoluble phosphates to potassium or sodium, resulting in the formation of soluble phosphate salts (Singh and Satyanarayana, 2011; Rinu et al., 2012) which is absorbable for plants.
Several studies have assessed the endophytic fungal communities of apple (e.g., Camatti-Sartori et al., 2005; Alijani et al., 2016a; Liu et al., 2017; Muresan, 2017; Afandhi et al., 2018; Liu et al., 2018; Arrigoni et al., 2020; Olivieri et al., 2021), some of which have focused on the biological control potential of the endophytes against apple fungal diseases. Based on the results, apple tissues are colonized by a diverse array of fungal taxa (e.g., Alternaria Nees, Arthrinium Kunze, Aspergillus P. Micheli ex Haller, Biscogniauxia Kuntze, Botryosphaeria Ces. & De Not., Chaetomium Kunze, Colletotrichum Corda, Dicyma Boulanger, Doratomyces Corda, Epicoccum Link, Neosetophoma Gruyter, Aveskamp & Verkley, Paraconiothyrium Verkley, Penicillium Link, Stemphylium Wallr., Trichoderma Pers., Trichothecium Link, Xylaria Hill ex Schrank, Sporobolomyces Kluyver & C.B. Niel, Rhodotorula F.C. Harrison, Debaryomyces Klöcker and Cryptococcus Kütz and etc). Alijani et al. (2016a) purified about 350 isolates from the shoots, leaves, and barks of endemic and commercial apple trees in West Azerbaijan province, Iran and identified 24 species belonging to 10 genera of Ascomycota. They examined the antagonistic properties of the isolates against Diplodia bulgarica A.J.L. Phillips, J. Lopes & Bobev, the causal agent of apple canker disease, in vitro and found various interactions in the paired combinations of endophytic fungi and D. bulgarica (Alijani et al., 2016b). Liu et al. (2017) highlighted the biological control potential of 81 endophytic fungi from apple shoots to preserve apple trees against Neonectria ditissima (Tul. & C. Tul.) Samuels & Rossman infection in vitro. They mentioned that 18 fungal isolates inhibited the radial growth of three N. ditissima isolates, among which 15 selected ones were identified as Epicoccum, Chaetomium, Biscogniauxia, Neosetophoma, and Penicillium species. In another study, a collection of 60 endophytic fungal isolates was obtained from apple trees in Canada, 60% of which were Penicillium or Trichoderma species (Muresan, 2017). Furthermore, 55 isolates significantly inhibited V. inaequalis growth in vitro, the most effective one of which was Fusarium oxysporum Schltdl. isolate FRS09 with 83% inhibition.
The endophytic fungi play roles in protecting the plant from herbivorous insects and diseases, as well as supporting the absorption process of the nutrients required for photosynthesis (Gimenez et al., 2007; Rozpądek et al., 2015; Vergara et al., 2017; Li et al., 2018). Given the crucial role of endophytic fungi, the present study focused on the endophytic fungi from wild and endemic apple cultivars in the north of Iran. In the study, the endophytic fungi were isolated from the healthy leaves, fruits, and branches of the cultivars, and tested for their efficacy against V. inaequalis and ability to prevent apple scab infection and its symptoms. The fungi were identified with consistent biological control capacity against apple scab in vitro and greenhouse tests. The results provide evidence that the naturally-occurring endophytic fungi can be a novel source for biological control agents.
Materials and methods
Sampling and endophytic fungi isolation
The healthy leaf, fruit, and branch samples of wild and endemic apple cultivars were collected from 70 trees in the north of Iran (Guilan, Mazandaran and Golestan provinces) during July–September 2019. They were placed in paper bags and stored at 4°C. Additionally, plant materials were disinfected based on the method modified by Strobel and Daisy (2003) (Ebrahimi et al., 2021). The fungi were separated on the three media of Water Agar (WA), Corn Meal Agar (CMA), and Potato Dextrose Agar (PDA), and hyphal tip method was applied for purification. All of the identified isolates were deposited in the Fungal Culture Collection (IRAN) of the Iranian Research Institute of Plant Protection (Tehran, Iran).
Endophytic fungi identification
The appearance of colony, structure and color of mycelium, type of teleomorph and/or anamorph, and morphology of ascoma, conidiomata, conidia, and conidiophores (e.g., size, color, shape, and ornamentation), as well as conidiogenous cells, and spore production mechanism were studied for examining fungi morphologically (Ellis, 1971, 1976; Sutton, 1980; Sivanesan, 1987; Klich and Pitt, 1988; Klich, 2002; Leslie and Summerell, 2006; Simmons, 2007). Each of the fungal isolates was separately sub-cultured on the PDA; Czapek Yeast Extract Agar (CYA; sucrose 30 g, yeast extract 5 g, K2HPO4 1 g, NaNO3 3 g, KCl 0.05 g, MgSO4.7H2O 0.05 g, FeSO4.7H2O 1 mg, agar 20 g/l, final pH 6.0–6.5); Carnation Leaf Agar (CLA; autoclaved Carnation leaves pieces on nearly solid 2% WA; agar 20 g/l water); Corn Meal Agar (CMA; corn meal infusion from 50 g solids, agar 20 g/l); Oatmeal Agar (OA; oat meal infusion from 30 g solids, agar 20 g/l); Potato Carrot Agar (PCA; potato infusion from 20 g solids, carrot infusion from 20 g solids, agar 20 g/l); Malt Extract Agar (MEA; malt extract 30 g, peptone 5 g, agar 20 g/l); Synthetic Nutrient Agar (SNA; KH2PO4 1 g, KNO3 1 g, MgSO4.7H2O 0.5 g, KCl 0.5 g, Glucose 0.2 g, Sucrose 0.2 g, agar 20 g/l); and Tap Water Agar plus wheat straw (TWA; agar 20 g/l tap water plus autoclaved wheat straw) for inducing sporulation. Further, microscopic slides were prepared in lacto-phenol or lacto-phenol cotton blue solutions after 7, 14, and/or 30 days (due to the fungal species), followed by assessment under a BH2 light microscope (Olympus, Japan). The isolates were classified into morphotypes according to their morphological appearance, and at least one isolate of each morphotype was kept for molecular identification.
Furthermore, the rapid simplified DNA extraction protocol provided by Cenis (1992) was employed for the DNA extraction from seven-day-old fresh mycelia. The fungal isolates were molecularly identified based on the Internal Transcribed Spacer (ITS)-rDNA, glyceraldehyde-3-phosphate dehydrogenase (gapdh), and translation elongation factor 1-alpha (tef-1α) sequences which were, respectively, amplified by using the ITS1/ITS4 (White et al., 1990), EF1/EF2 (O'Donnell et al., 1998) and gpd1/gpd2 (Berbee et al., 1999) primer pairs related to the fungal genus. In the present study, the reaction mixture and PCR conditions for ITS, tef-1α, and gapdh were the same as those presented by Ebrahimi and Fotouhifar (2016), O'Donnell et al. (1998), and Song et al. (2019), respectively. PCR products were purified and directly sequenced in one direction with ITS1, EF1, and gpd1 primers by BGI Company (Denmark), respectively.
The ITS, tef-1α, and gapdh sequences were compared with those of the most closely-related fungal species according to the NCBI BLAST program, relevant websites, observed colony, and spore morphology to confirm the taxonomic status of the intended fungal isolates. Finally, the sequence data were deposited in the GenBank database.
For phylogenetic analyses, sequences of genomic regions of gapdh, tef-1α, and ITS from different species were aligned with the homologous reference sequences of the respective genomic regions of related species obtained from GenBank (Supplementary Table S1) using ClustalW (Thompson et al., 1994). Maximum likelihood (ML) (Felsenstein, 1981) analysis was done by heuristic search with MEGA software ver. 7 (Kumar et al., 2016). Models TN93 + G, K2 + G and K2 + I were recommended by MEGA as the optimal nucleotide substitution models for gapdh, tef-1α, and ITS data, respectively. Characters were treated as un-weighted and unordered with gaps treated as missing data. Confidence of individual clades was assessed by ML bootstrap analysis (Felsenstein, 1985) with 1,000 replicates.
Biocontrol experiments in vitro
Cellophane membrane-based method
The cellophane membrane-based method (Dennis and Webster, 1971) was performed in duplicate to screen the endophytic isolates based on their antagonism against V. inaequalis (IRAN 16870 F). Then, the isolates expressing the visually-detected antifungal activity (Table 1) were subjected to a secondary screening (the cellophane membrane-based method in triplicate). In this technique, a sterile cellophane membrane of the same diameter as a Petri plate was overlaid on the PDA medium by using sterile forceps. A disc of endophytic isolate was inoculated at the membrane center and maintained at 20°C for 3–5 days related to isolate growth speed. Following incubation, the isolate culture along with the membrane was carefully removed from the plate, and a plug of V. inaequalis was positioned on the plate and kept at 20°C for 1 month. The colony diameter of V. inaequalis was measured and compared to the value obtained by culturing V. inaequalis on fresh PDA plates (control treatment). The percentage of growth inhibition was calculated by using the formula n = (a − b)/a × 100, where n is considered as the growth inhibition percentage, a indicates the colony diameter of uninhibited V. inaequalis, and b shows the colony diameter of antagonist-treated V. inaequalis (Etebarian et al., 2005).
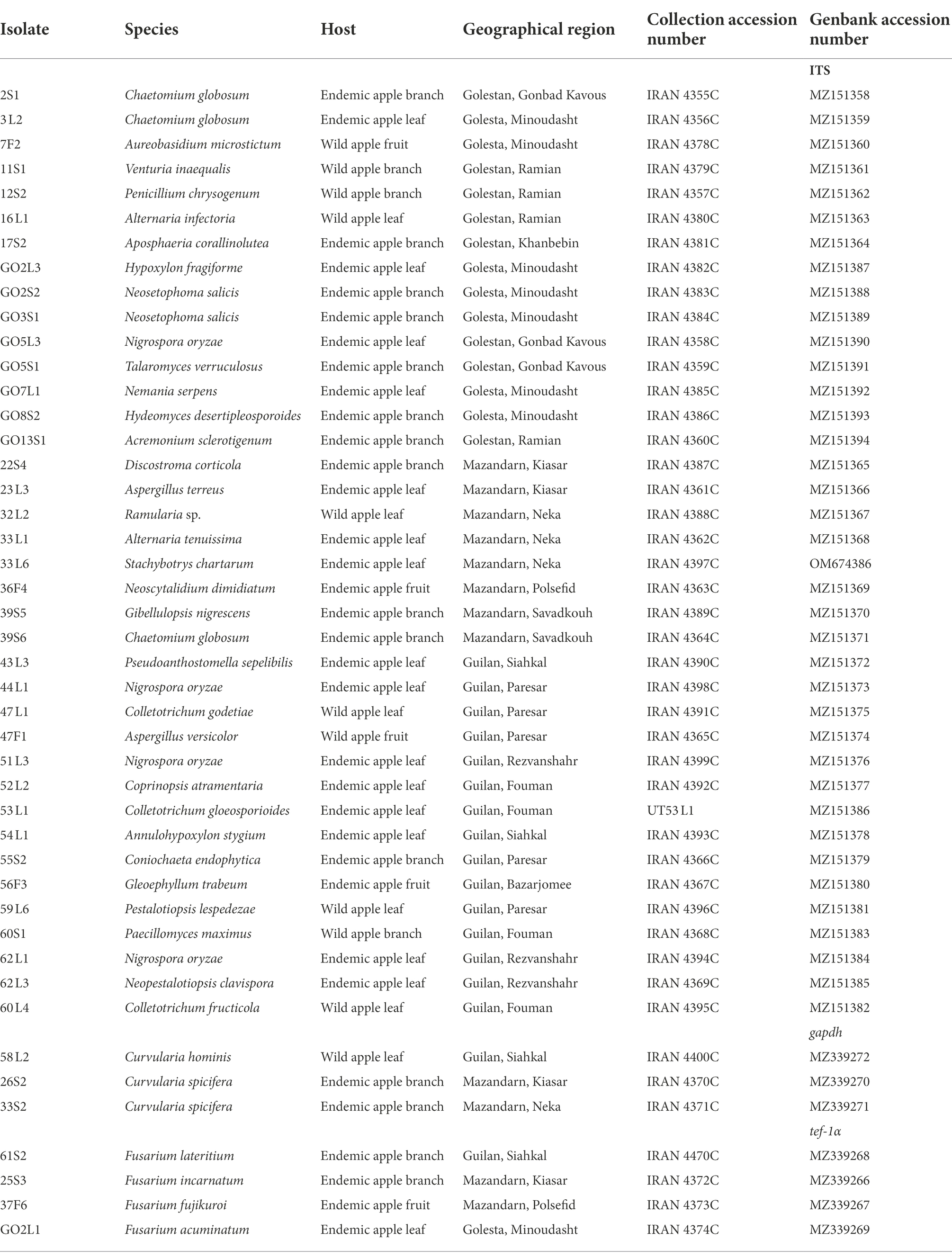
Table 1. Identified endophytic fungi of Iranian endemic and wild apple based on morphology and by ITS, translation elongation factor 1-alpha (tef-1α) and glyceraldehyde-3-phosphate dehydrogenase (gapdh) sequences.
Volatile organic compound-mediated interactions
In addition, the effect of endophyte Volatile Organic Compounds (VOCs) on V. inaequalis was examined by using sandwiched Petri plates explained by Lillbro (2005). After inoculating the endophytic isolates and V. inaequalis on the PDA plates, V. inaequalis plates were placed on top of an isolate plate, sealed with Parafilm, and incubated at 20°C. Further, plates with V. inaequalis were sandwiched with uninoculated PDA plates as a control treatment. Each treatment was repeated three times. The colony diameter of V. inaequalis was obtained after 1 month and the above-mentioned formula was used to compute the growth inhibition percentage.
Chitinase activity
The method of Hsu and Lockwood (1975) was applied to determine chitinase production. Briefly, the endophytic isolates were grown on the chitin agar containing 0.4% colloidal chitin and 1.5% agar adjusted to pH 7.2. Furthermore, the colloidal chitin was prepared according to Berger and Reynolds (1958). The plates were incubated for 5 days at 25°C. The ability of chitinase production was revealed by a clear halo around the colonies. Ultimately, the ratio of the clear zone diameter to colony diameter was calculated as chitinase activity.
Cellulase activity
Regarding congo red cellulase activity, all fungal isolates were developed on the carboxymethyl cellulose (CMC) agar medium consisting of 0.4 g/l KH2PO4, 0.02 g/l CaCl2, 0.02 g/l NaCl, 0.02 g/l FeSO4 7H2O, 2.5 g/l CMC, and 15.0 g/l agar at 25°C for 7 days. The pH was set to 7.2 by using 1 M NaOH. To visualize the hydrolysis zone, agar medium was flooded with an aqueous congo red solution (1 mg/ml) for 20 min. Then, congo red solution was poured off, and the plates were further treated by flooding with 1 M NaCl for 15 min. The cellulase activity was obtained by measuring the diameter of the clear zone around each colony. In fact, it was computed as the ratio of the clear zone diameter to colony diameter (Majidi et al., 2011).
Phosphate solubilization
To assess phosphate solubilization ability, a five-mm agar plug of the endophytic isolate was placed in the center of a plate containing the Sperber medium (Sperber, 1958) prepared with insoluble phosphate. Before autoclaving, the medium pH was adjusted to 7.2. The cultures were incubated at 25°C for 7 days. Then, solubilization index was evaluated based on the ratio of the clear zone diameter to colony diameter.
Greenhouse experiments
Additionally, the two-year-old seedlings of Golab apple cultivar., which is sensitive to apple scab disease, were utilized in this study. The pathogenicity test was first conducted to ensure the pathogenicity of V. inaequalis isolate and optimize the greenhouse conditions for further tests. The conidia suspension (105 conidia/ml) of V. inaequalis was sprayed on the apple leaves. Further, all of the inoculated branches were individually covered with plastic bags for maintaining a relative humidity of 100% for 48 h. Then, the pots were kept in a greenhouse with the humidity of >80% at 18–20°C, followed by the daily monitoring of the symptom appearance.
The selected endophytic isolates were tested for biological control potential. For this purpose, the apple seedlings were inoculated with endophytic isolates at a final concentration of 108 conidia/ml. At 48 h after antagonist inoculation, V. inaequalis suspension was inoculated. To do this, as we needed much amount of pathogen suspension, hyphal suspension of the pathogen was prepared from 3-week-old colony grown on PDA (in 6 cm plates) at 25°C and homogenized in distilled water. The culture of the isolate was put into a mortar and ground with a small amount of sterile water to make a syrupy suspension, and it was sprayed on the apple leaves. Each branch was covered with a plastic bag for 48 h and then kept in a greenhouse with the humidity of >80% at 18–20°C. Three seedlings were sprayed with V. inaequalis and sterile distilled water as the positive and negative controls, respectively. Each treatment was performed on three seedlings, the tests were repeated twice, and the symptom appearance was examined daily. The infection percentage was determined by measuring individual leaf area and disease symptoms after 1 month of pathogen inoculation. Finally, the disease inhibition rate of each antagonist was calculated compared to the control. To meet Koch’s postulates, the pathogen isolate was reidentified morphologically based on structures produced on leaf spots.
Statistical analysis
The experiments were conducted in a completely randomized design and complete randomized block design for in vitro and in vivo tests, respectively. Data were analyzed by using SAS software version 9.0. All the data were subjected to the analysis of variance (ANOVA) followed by Duncan’s multiple range test for determining mean differences (Steel and Torrie, 1980).
Results
Endophytic fungal isolates
A total of 70 apple samples (each consisted of branch, leaf, and fruit) were collected, of which 28 (21 endemic and 7 wild apple), 22 (10 endemic and 12 wild apple), and 20 (15 endemic and 5 wild apple) were gathered from Golestan, Mazandaran, and Guilan provinces, respectively (Figure 1). In addition, 417 endophytic fungal isolates were obtained, among which 129 (16% leaf, 11% branch, 4% fruit), 172 (24% leaf, 11% branch, 6% fruit), and 116 (18% leaf, 5% branch, 5% fruit) were, respectively, related to Golestan, Mazandaran, and Guilan provinces (Figures 1, 2).
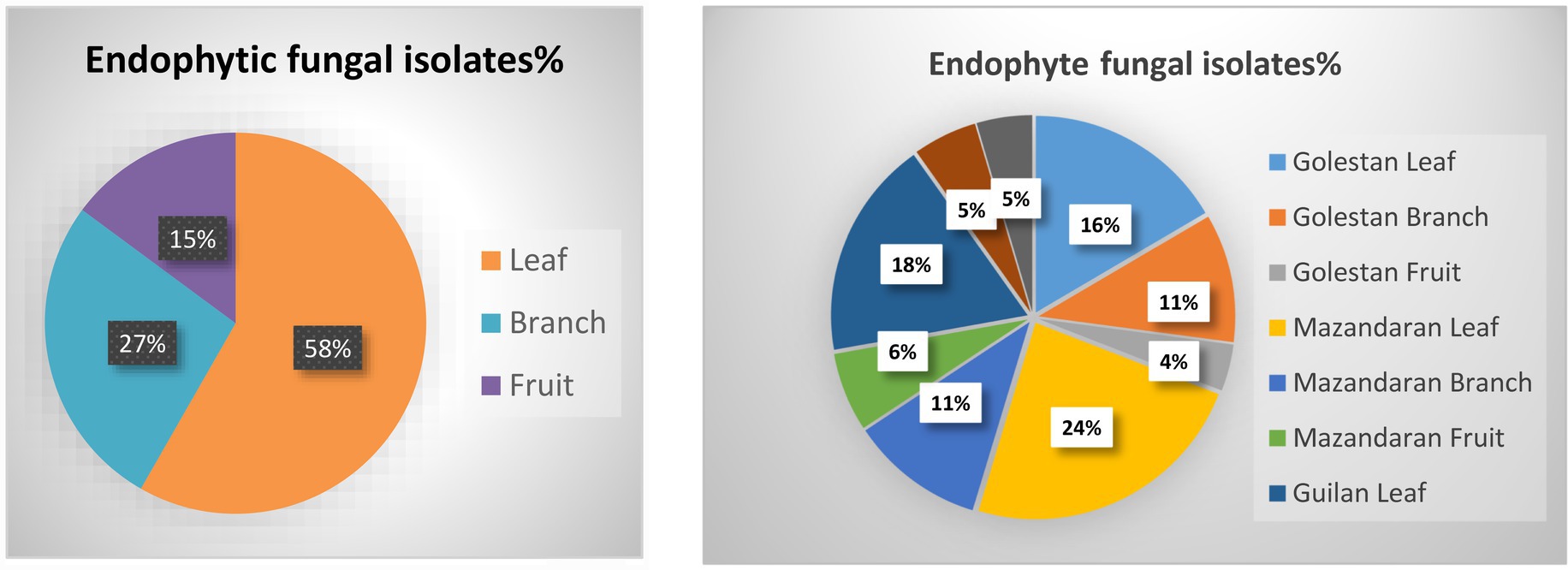
Figure 1. Endophytic fungal isolates were obtained from leaf, branch and fruit samples of wild and endemic apple cultivars collected from Golestan, Mazandaran and Guilan provinces of Iran.
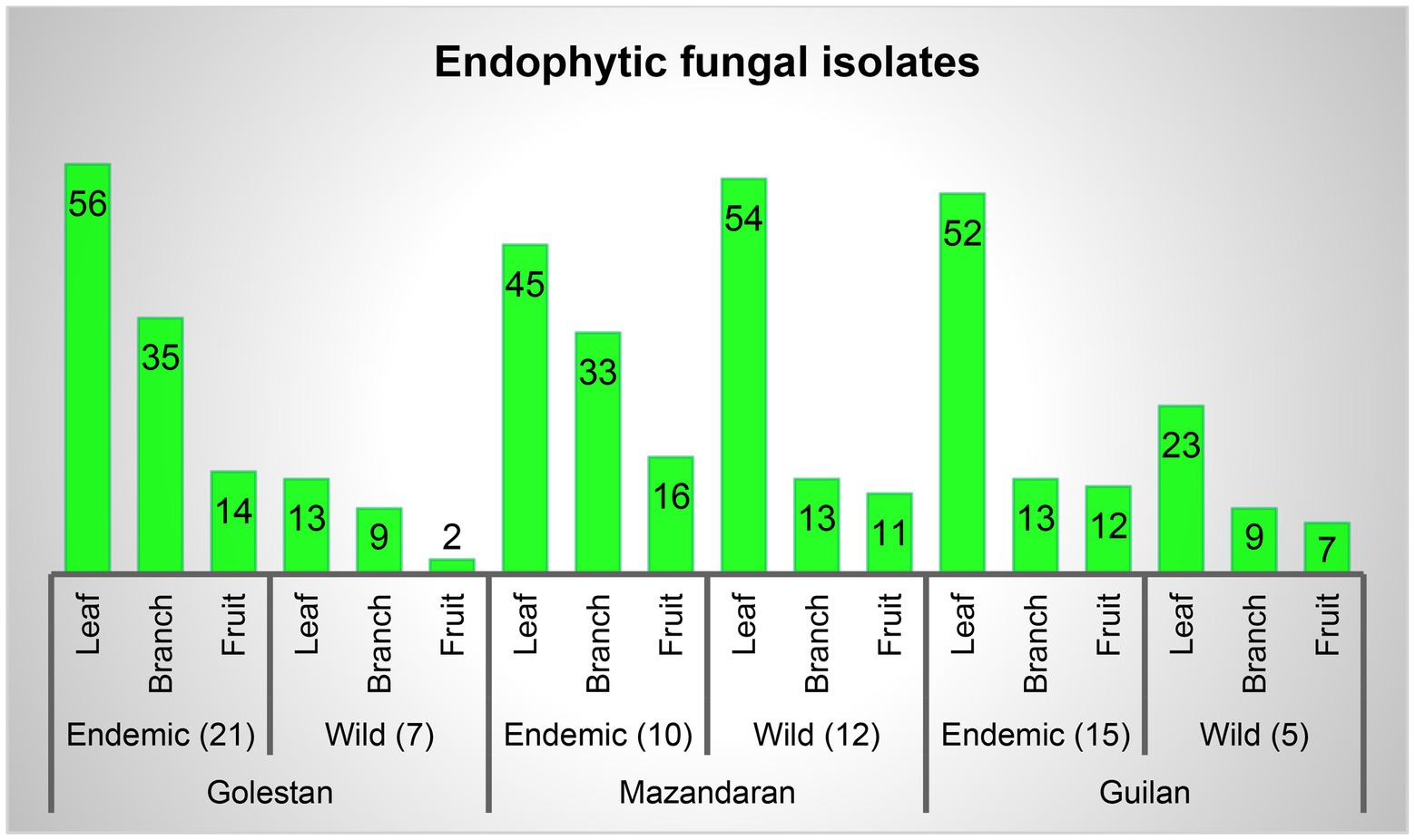
Figure 2. Endophytic fungal isolates percentage in leaf, branch and fruit samples, and separately in each province.
Based on the morphological characteristics, 33 fungal genera were detected in the purified fungal isolates (Figure 3). Among these genera, 38 species were identified by considering the morphological properties and molecular data of gapdh (Figure 4), tef-1α (Figure 5), and ITS (Figure 6) genomic regions (Table 1). The results indicated the assignment of the detected isolates to Ascomycota (67.8%), mycelia sterilia (31.4%), Basidiomycota (0.48%), and Mucoromycota (0.24%). The species of Coprinopsis atramentaria (Bull.) Redhead, Vilgalys & Moncalvo, and Gloeophyllum trabeum (Pers.) Murrill, N. Amer. Fl. belonged to orders Agaricales and Gloeophyllales from the class Agaricomycetes of Basidiomycota, respectively. The identified species of Ascomycota were categorized into the three classes of Sordariomycetes, Dothideomycetes, and Eurotiomyetes (Figure 6). Further, the Sordariomycetes included seven separate groups related to orders Amphisphaeriales, Xylariales, Hypocreales, Glomerellales, Coniochaetales, Sordariales, as well as another group concerning Nigrospora Zimm. genus which is placed in Apiosporaceae, Sordariomycetidae families incertae sedis. The members of Dothideomycetes were grouped into the five orders of Venturiales, Pleosporales, Capnodiales, Dothideales, and Botryosphaeriales. The third class, Eurotiomyetes, involved the members of order Eurotiales.
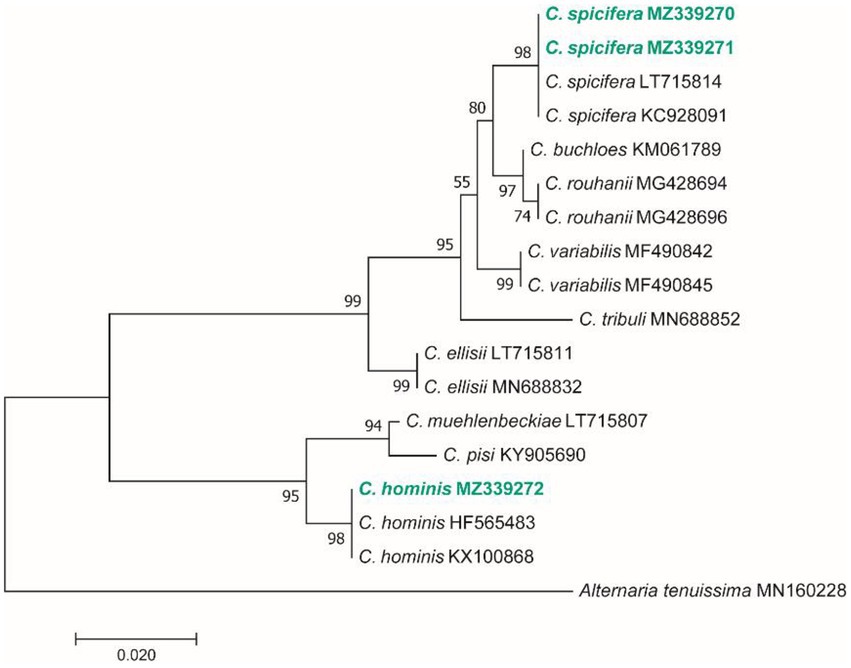
Figure 4. Maximum Likelihood (ML) tree based on aligned sequences of gapdh gene of 18 isolates generated in MEGA 7 under TN93 + G model. The tree was rooted to Alternaria tenuissima (IRAN 2428C). Bootstrap values (1,000 replicates) indicated at the nodes. The scale bar indicates nucleotide substitution in ML analysis, values ≥50% are shown above/below the branches. The surveyed isolates in the current study are highlighted in bold.
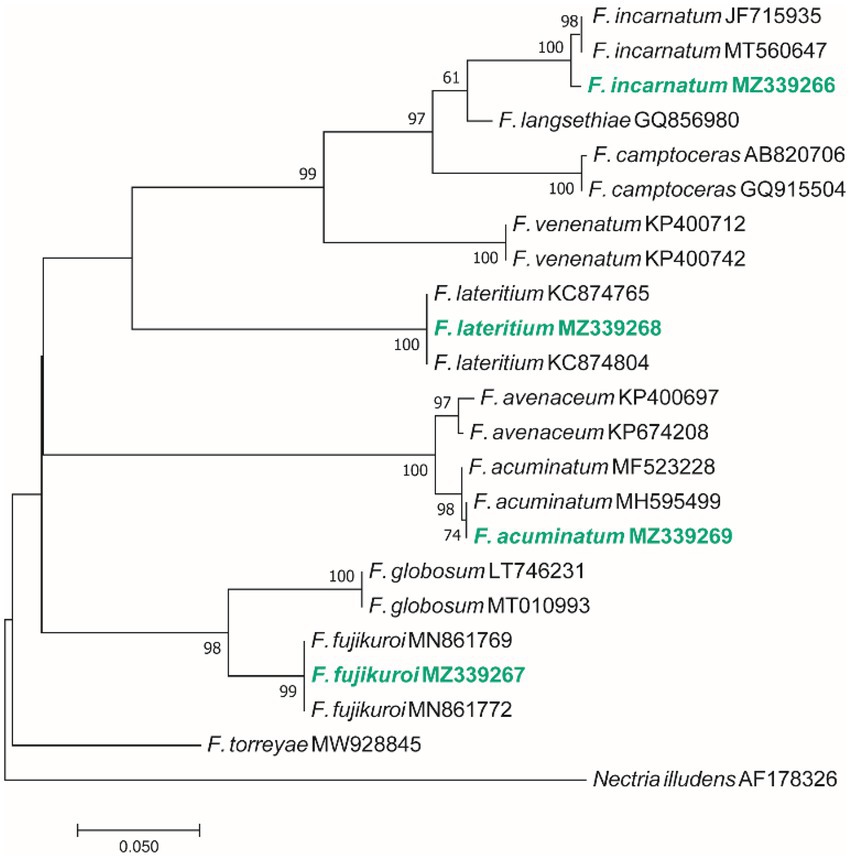
Figure 5. Maximum Likelihood (ML) tree based on aligned sequences of tef-1α gene of 23 isolates generated in MEGA 7 under K2 + I model. The tree was rooted to Nectria illudens (NRRL 22090). Bootstrap values (1,000 replicates) indicated at the nodes. The scale bar indicates nucleotide substitution in ML analysis, values ≥50% are shown above/below the branches. The surveyed isolates in the current study are highlighted in bold.
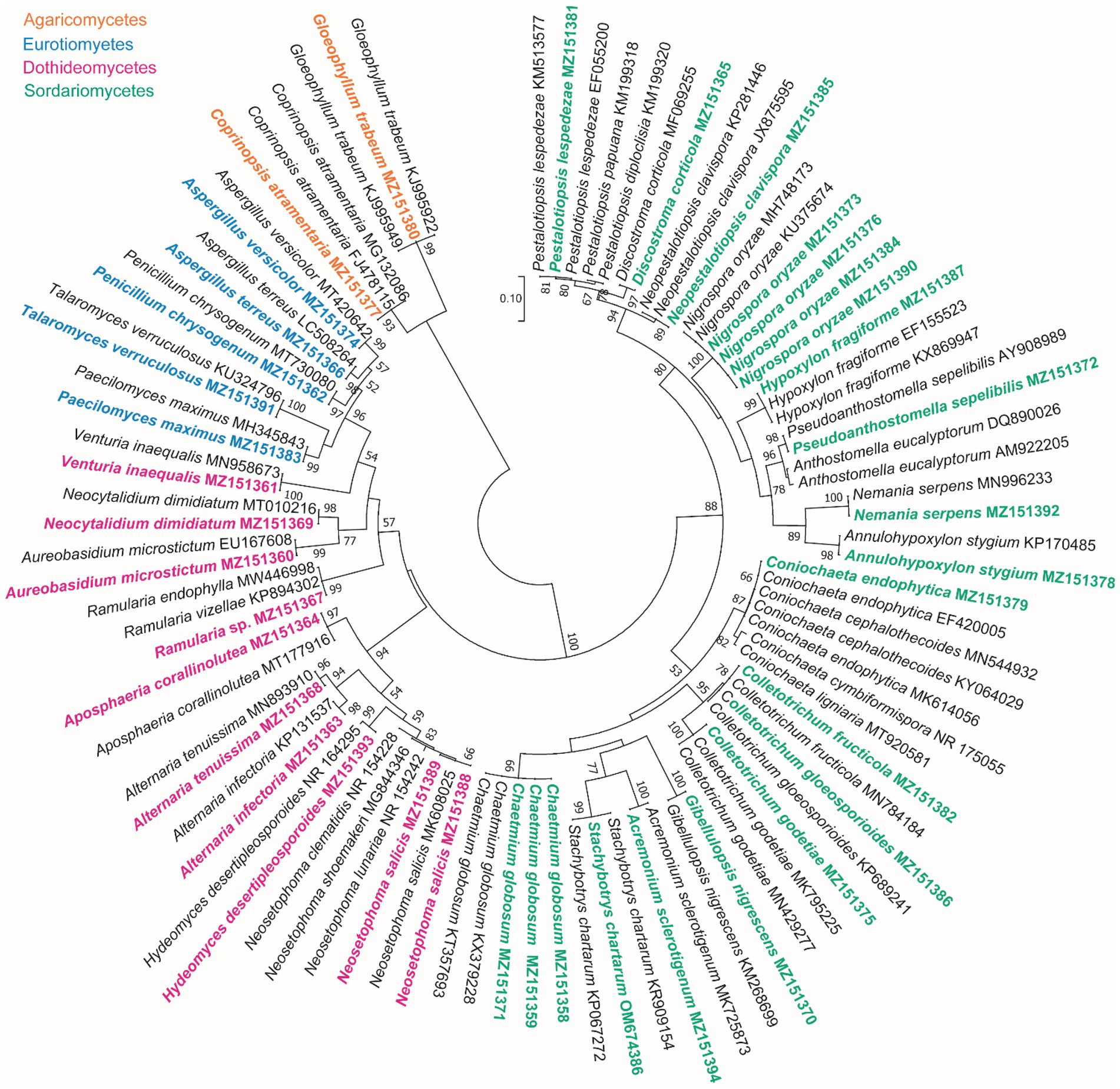
Figure 6. Maximum Likelihood (ML) tree based on aligned sequences of ITS region of 92 isolates generated in MEGA 7 under K2 + G model. Bootstrap values (1,000 replicates) indicated at the nodes. The scale bar indicates nucleotide substitution in ML analysis, values ≥50% are shown above/below the branches. The surveyed isolates in the current study are highlighted in bold.
Screening of endophytic fungi for antifungal activity
In this study, the endophytic fungal isolates were evaluated for antifungal activity through employing the cellophane membrane-based method. They were tested in terms of the ability to produce antifungal media-permeable metabolites against V. inaequalis. The other approaches such as dual culture assay are not applicable since V. inaequalis is a slow-growing fungus. Furthermore, the isolates belonged to the geneus Alternaria, Aspergillus, Colletotrichum, Neoscytalidium Crous & Slippers, Nigrospora, Paecilomyces Bainier, Pestalotiopsis Steyaert, Penicillium and Talaromyces Visagie, N. Yilmaz & K. Jacobs did not exhibit antagonistic effect. The antifungal properties against apple scab agent were observed in 29 isolates (Table 2).
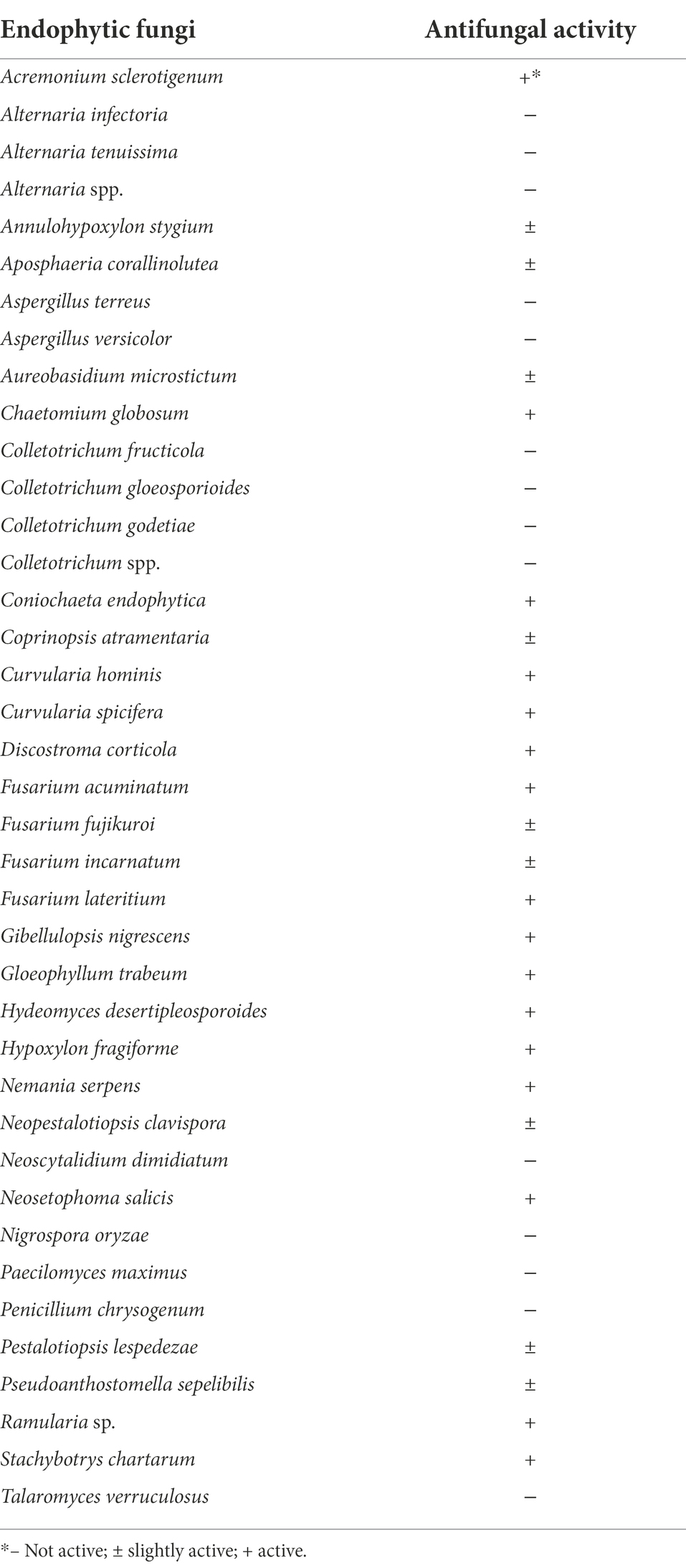
Table 2. Antifungal activity of endophytic fungi isolated from apple based on the cellophane membrane-based method.
Figures 7, 8 display the results related to the media-permeable metabolites of 29 endophytic isolates on the mycelial growth of V. inaequalis. As shown, the metabolites of Acremonium sclerotigenum (Moreau & R. Moreau ex Valenta) W. Gams GO13S1, Coniochaeta endophytica A.H. Harrington & A.E. Arnold 55S2, and Fusarium lateritium Nees 61S2 lead to the maximum growth inhibition (100%) of V. inaequalis, followed by Hydeomyces desertipleosporoides Maharachch., H.A. Ariyaw., Wanas. & Al-Sadi GO8S2 (87.7%) and Chaetomium globosum Kunze 2S1 (78.7%).
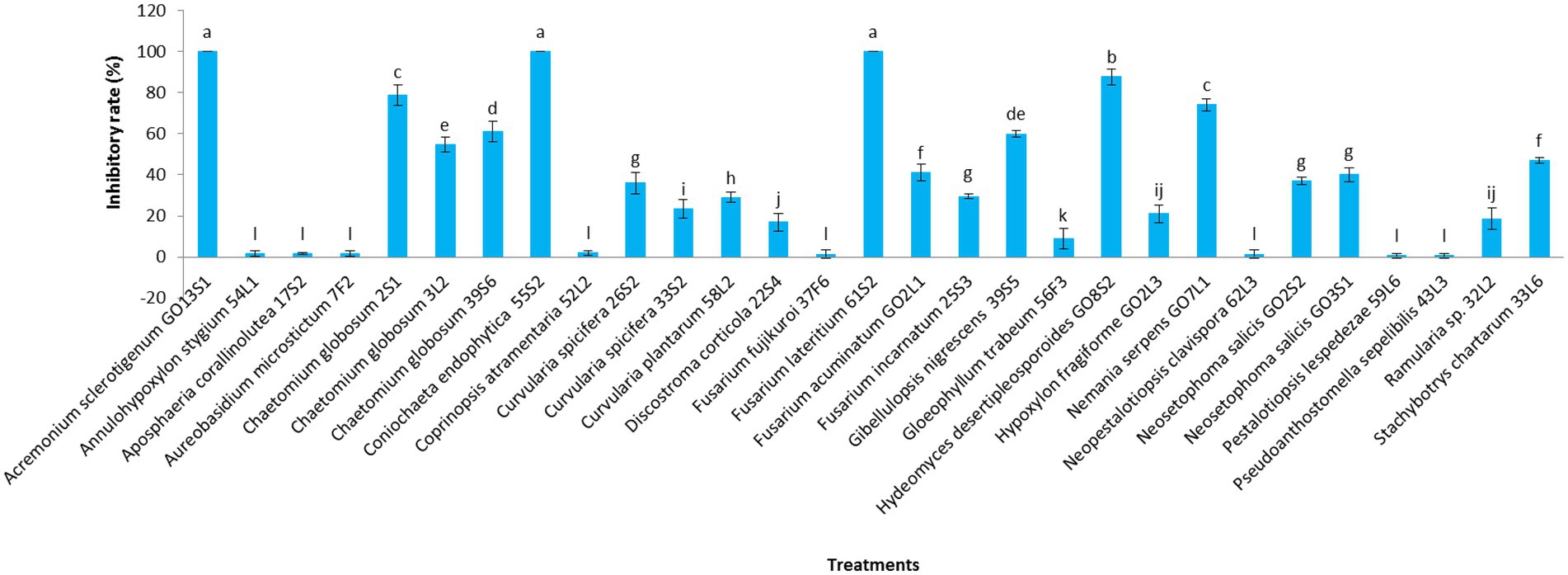
Figure 7. Effect of media-permeable metabolites of endophytic fungi isolated from apple on Venturia inaequalis mycelia growth after 1 month in vitro. Data are the means ± SE of three replicates. Values of histograms with common letters are not significantly different at p ≤ 0.01 according to Duncan’s multiple range test.
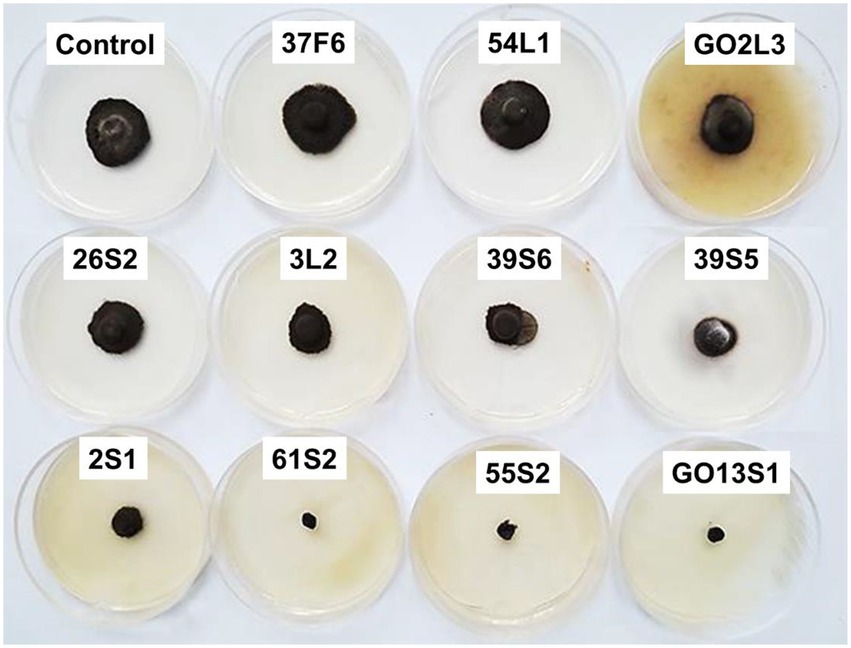
Figure 8. Effect of media-permeable metabolites of endophytic fungi isolated from apple on Venturia inaequalis mycelia growth in comparison to control after 1 month in vitro.
Additionally, the VOC production was assessed in the isolates. The VOCs produced by the isolates of Aureobasidium microstictum (Bubák) W.B. Cooke 7F2, Ch. globosum 2S1, Ch. globosum 3 L2, Coprinopsis atramentaria 52 L2, Fusarium fujikuroi Nirenberg 37F6, Fusarium acuminatum Ellis & Everh. GO2L1, and Fusarium incarnatum (Desm.) Sacc. 25S3 completely prevented the mycelia growth of V. inaequalis. However, the minimum pathogen growth inhibition was detected in the VOCs of Annulohypoxylon stygium (Lév.) Y.M. Ju, J.D. Rogers & H.M. Hsieh 54 L1 (12.4%) and Hypoxylon fragiforme (Pers.) J. Kickx f. GO2L3 (9.6%). The VOCs of other isolates except Aposphaeria corallinolutea Gruyter, Aveskamp & Verkley 17S2 and Discostroma corticola (Fuckel) Brockmann 22S4 inhibited pathogen mycelia growth by more than 70% (Figures 9, 10).
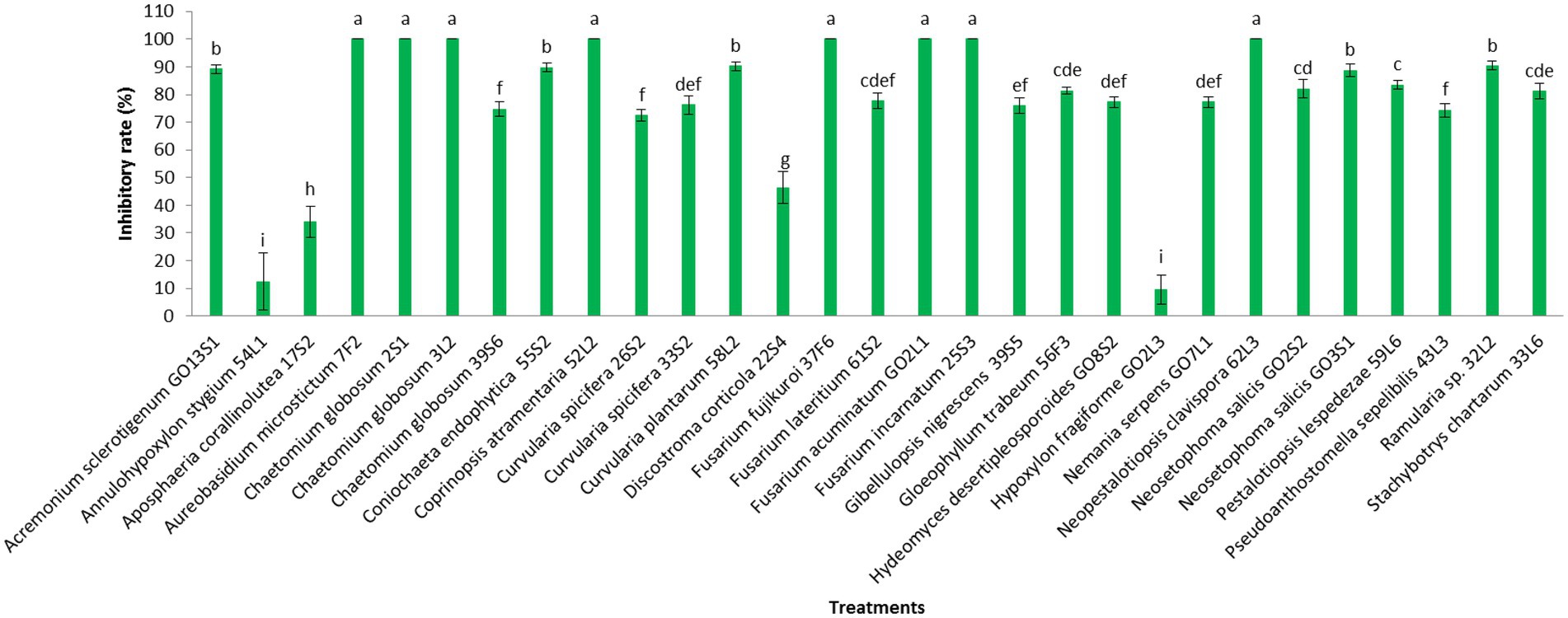
Figure 9. Effect of volatile organic compounds of endophytic fungi isolated from apple on Venturia inaequalis mycelia growth after 1 month in vitro. Data are the means ± SE of three replicates. Values of histograms with common letters are not significantly different at p ≤ 0.01 according to Duncan’s multiple range test.
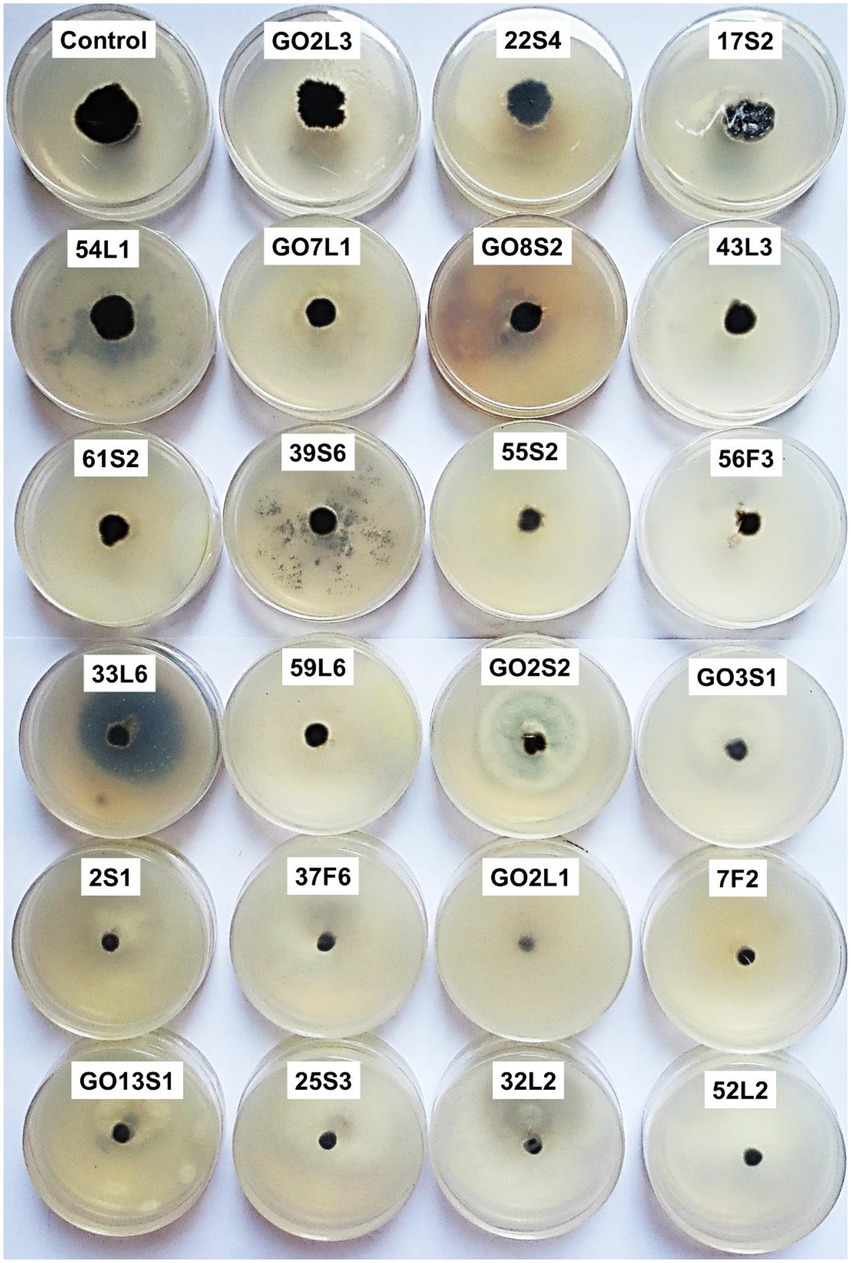
Figure 10. Effect of volatile organic compounds of endophytic fungi isolated from apple on Venturia inaequalis mycelia growth in comparison to control after 1 month in vitro.
Phosphate solubilization and enzyme activity
Based on the results of the biological control tests, six isolates were selected for further evaluation, of which Co. endophytica 55S2 and F. lateritium 61S2 could solubilize inorganic phosphate with the ratio of 1.2 and 1.07, respectively (Table 3). All isolates except Ch. globosum 3 L2 represented cellulase activity, which the highest halo zone/colony diameter ratio (2.62) was recorded for Au. microstictum 7F2 (Table 3). Further, chitinase activity was observed in the isolates of Ch. globosum 2S1, Ch. globosum 3L2, and F. lateritium 61S2 with the halo zone/colony diameter ratio of 1.2, 1.2, and 1.1, respectively (Table 3).
Biocontrol assays under greenhouse conditions
As already mentioned, six isolates were further tested in whole-plant tests under greenhouse conditions. The results demonstrated a significant reduction in the apple scab severity after 1 month when endophytes were inoculated on the leaves 48 h before pathogen (Figures 11, 12). The in vivo tests revealed the complete control of apple scab disease by Co. endophytica 55S2 and Ch. globosum 2S1. Furthermore, the decrease in the apple scab severity was similar for F. lateritium 61S2 and Ch. globosum 3 L2, which ranged between 50 (F. lateritium 61S2) and 62% (Ch. globosum 3L2). Finally, Au. microstictum 7F2 and Ac. sclerotigenum GO13S1 declined the disease severity by 37.5% (Figures 11, 12).
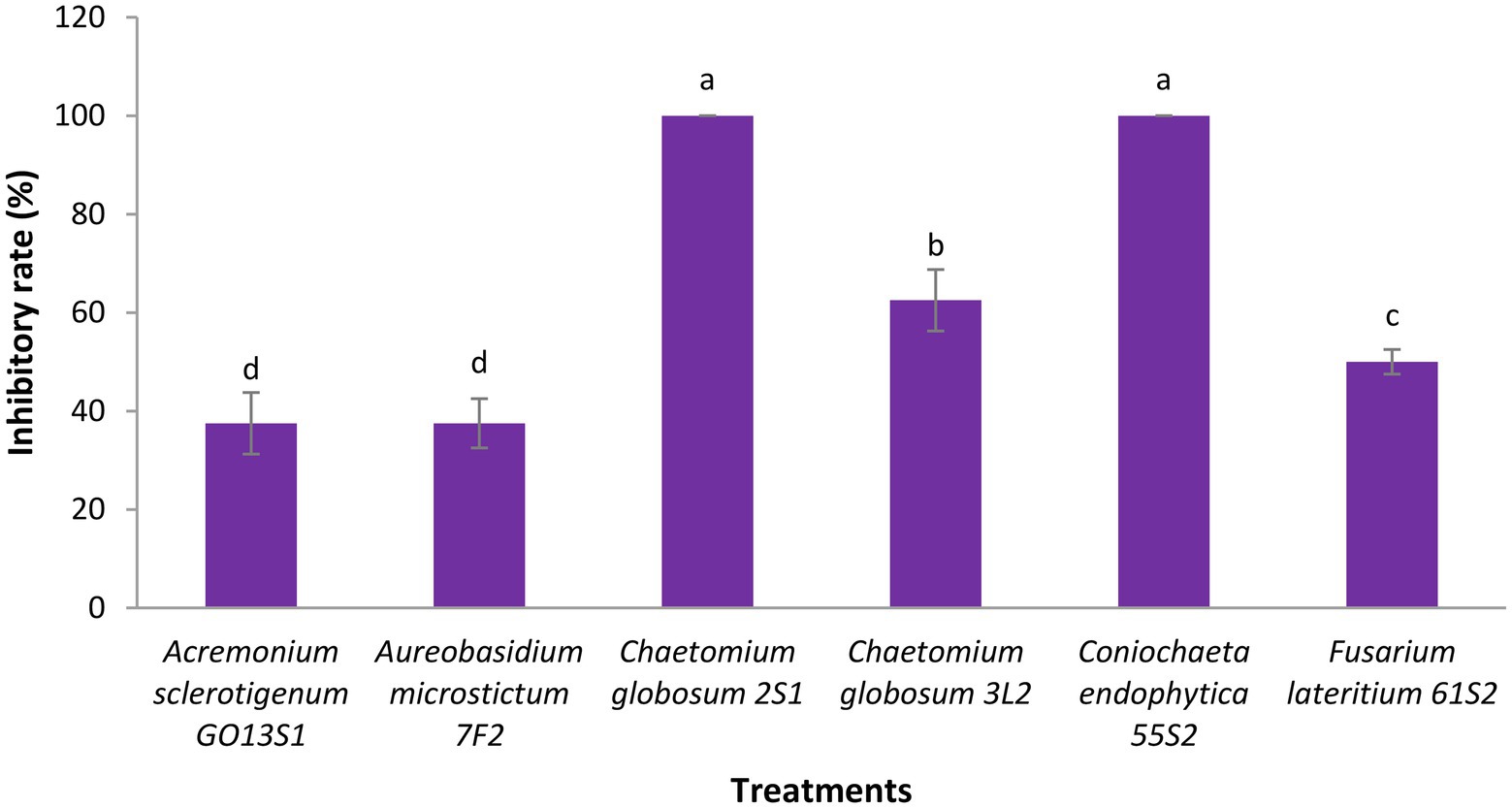
Figure 11. Antagonistic effect of endophytic fungi isolated from apple against apple scab disease on apple seedling after 1 month of pathogen inoculation under greenhouse conditions. Data are the means ± SE of three replicates. Values of histograms with common letters are not significantly different at p ≤ 0.01 according to Duncan’s multiple range test.
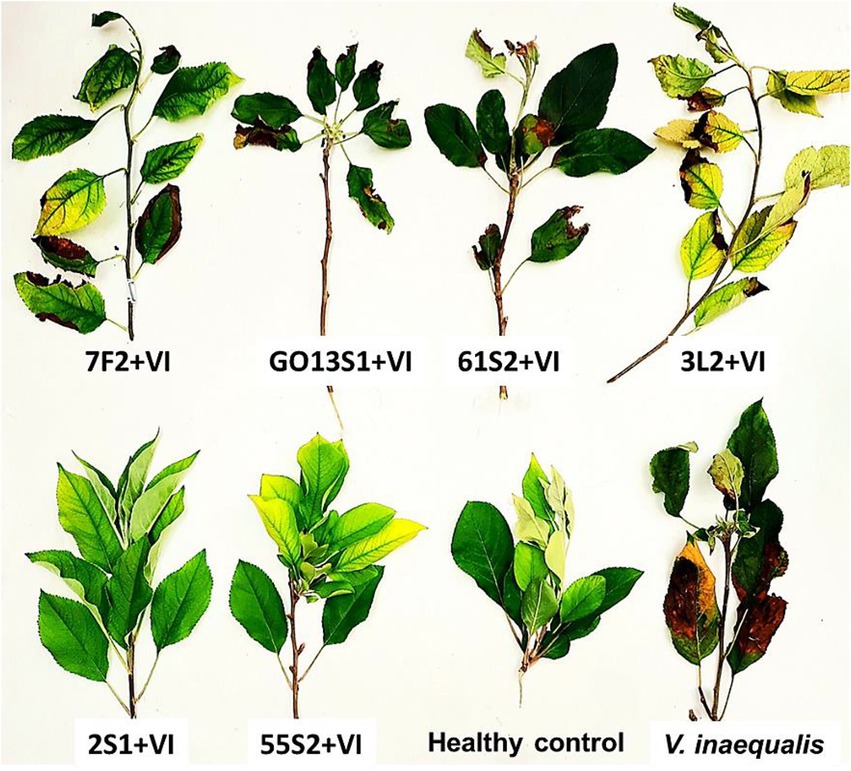
Figure 12. Effect of endophyte isolates on development of symptoms caused by Venturia inaequalis on apple leaves at 18–20°C after 1 month of pathogen inoculation in greenhouse. (Treatments: Aureobasidium microstictum 7F2, Acremonium sclerotigenum GO13S1, Fusarium lateritium 61S2, Chaetomium globosum 3 L2, Chaetomium globosum 2S1, Coniochaeta endophytica 55S2, VI: Venturia inaequalis).
Discussion
The plant-associated habitat is considered as a dynamic environment, in which many factors affect the structure and composition of the species colonizing various tissues. The endophytic communities may spatially vary in many kinds of plants (Rivera-Orduña et al., 2011). In addition, microorganism population can be different in natural forest and agroecosystem due to the use of synthetic chemicals by farmers. The present study highlighted the endophytic fungi associated with the wild and Iranian endemic apple cultivars which are mostly spread along the Caspian Sea coast in the north of Iran. The results revealed the differences in the apple branch, fruit, and leaf tissues in terms of the richness and abundance of endophytic fungi. Among the 417 endophytic isolates under study, 243, 112, and 62 were obtained from leaves, branches, and fruits, respectively. Generally, leaves carry more endophytic fungi than the stem due to the exposure of larger surface area to the outer environment and presence of numerous stomata which facilitate the entry of fungal hyphae (Gond et al., 2012). Camatti-Sartori et al. (2005) reported the greatest total number of endophytic isolates from the orchards under organic cultivation compared to the integrated and conventional cultivation systems. Alijani et al. (2016a) separated 14.37, 28.34, and 57.28% of endophytic fungal isolates from the apple leaf, annual and biennial branches, and bark in the commercial orchards of West Azerbaijan province, Iran (n: 350). These results are inconsistent with those of the present study regarding endophytic fungal diversity on the wild and endemic apple cultivars from the forests and natural regions. Alijani et al. (2016a) suggested the possible effect of fungicide application in commercial orchard on the endophytic fungal abundance of apple leaves. However, many other factors such as climate, orchard location, and host cultivar may influence the abundance and diversity of endophytic fungi. Afandhi et al. (2018) found that the abundance and diversity of endophytic fungi were maximized in mature apple leaves compared to the young and old ones. According to Arrigoni et al. (2020), bark age and orchard location strongly affected fungal and bacterial diversity. Further, scab disease management had an effect on the abundance of some taxa depending on bark age, orchard location, and sampling time (Arrigoni et al., 2020). Interestingly, Olivieri et al. (2021) mentioned the presence of significant differences between canker-resistant and susceptible apple cultivars with respect to the endophyte community.
In the present study, 417 endophytic fungi were separated from apple, which mostly belonged to Ascomycota (77.93%). The genera Alternaria, Cladosporium, Nigrospora, Colletotrichum, Fusarium, Chaetomium, and Curvularia were the most frequent fungi, the isolate number of which was 50, 49, 39, 23, 16, 9, and 8, respectively. Camatti-Sartori et al. (2005) identified genera Colletotrichum, Xylaria, and Botryosphaeria as the most common endophytic fungi of apple in Brazil. Based on the results of Liu et al. (2018), Ascomycota (47.8%), Mucoromycota (31.1%), and Basidiomycota (11.6%) were the dominant endophytic fungal phyla in all apple samples studied in the USA. In general, Zoophthora Bałazy & Manole (31%), Cladosporium (17.3%), and Aureobasidium (11%) constituted more than 59% of the detected fungi, and the next genera were Alternaria (5.6%) and Aspergillus (1.6%), respectively (Liu et al., 2018). Alijani et al. (2016a,b) reported the presence of 12 genera of endophytic fungi, Alternaria, Arthrinium, Aspergillus, Chaetomium, Cytospora Ehrenb., Dicyma, Doratomyces, Paraconiothyrium, Periconia Tode, Stemphylium, Trichoderma, and Trichothecium, on the apple cultivated in the West Azerbaijan province, Iran. Muresan (2017) gathered a collection of 60 fungal isolates, mostly obtained from apple tree roots (78%) in Canada, 60% of which were Penicillium or Trichoderma species. Liu et al. (2017) referred to Epicoccum, Chaetomium, Biscogniauxia, Neoseptophoma, and Penicillium as the most species identified in the culturable endophytes from apple shoots in New Zealand. According to Afandhi et al. (2018), Aspergillus was dominant in the endophytic fungal isolates of apple.
The biological control of plant pathogens instead of synthetic fungicides adequately protects plants, humans and other animals, and natural environment. The endophytic fungi play a key role in plant protection against biotic and abiotic stresses (Lugtenberg et al., 2016) through the various modes of action such as the mycoparasitism, antibiosis, competition, and plant resistance induction (Latz et al., 2018). The mechanisms may act coordinately, and their importance in the biological control process depends on the antagonist strain, pathogenic fungus, host plant, and environmental conditions (Golafrouz et al., 2020).
Many active metabolites are produced by antagonistic fungi, some of which possess significant biological activities like cytotoxicity, enzyme inhibition, and antibiosis (Yang S. X. et al., 2011; Yang S. Z. et al., 2011). Furthermore, some antagonistic fungi secrete various hydrolytic enzymes such as chitinase, glucanase, and protease to effectively digest the cell walls of their competitors, inhibit their ss growth, or surpass their capacity for metabolizing available resources leading to competitive exclusion in substrate (Wilkins et al., 2003). The endophytic fungi colonize plant tissues, as well as obtaining nutrients and highly-active cellulases produced to help the host plant defend themselves against invading pathogens (Marques et al., 2018). Therefore, some enzyme activities of endophytes such as cellulase are important in biological control. Additionally, phosphorus is known as a major plant nutrient, the insoluble calcium phosphate salts of which are mostly formed in the presence of a high concentration of calcium ions (Chen and Barak, 1982). Thus, the ability of endophytes to solubilize inorganic phosphate can help plant by improving their growth. In the present study, the six isolates of Ac. sclerotigenum GO13S1, Co. endophytica 55S2, F. lateritium 61S2, Au. microstictum 7F2, Ch. globosum 2S1, and Ch. globosum 3 L2 were selected for greenhouse tests based on the results of media-permeable metabolites and VOC tests. Among the intended isolates, Co. endophytica 55S2 and F. lateritium 61S2 had the ability to solubilize inorganic phosphate, which the ability should be investigated in soil environment to affects plant growth and disease biocontrol. The cellulase activity was observed in all isolates except Ch. globosum 3 L2, while Ch. globosum 2S1, Ch. globosum 3 L2, and F. lateritium 61S2 exhibited chitinase activity. The results demonstrated the successful biological control of apple scab disease following the use of endophytic fungi in greenhouse so that Co. endophytica 55S2 and Ch. globosum 2S1 completely controlled the disease on apple seedling leaves.
The various studies around the world have revealed the biological control potential of different apple endophytic fungi against apple fungal pathogens. For example, Alijani et al. (2016b) evaluated the antifungal potential of 15 endophytic species of apple against D. bulgarica in vitro. They found that pathogen mycelia growth significantly reduced by Trichoderma harzianum Rifai and Trichoderma longibrachiatum Rifai. According to Muresan (2017), 55 endophytic isolates of apple among 60 ones significantly prevented V. inaequalis growth in vitro and F. oxysporum FRS09 was determined as the most effective isolate with 83% inhibition. Doolotkeldieva and Bobusheva (2017) examined the two biological control agents of Trichoderma viride Pers. and Streptomyces sр. against apple scab disease in vitro and field conditions. The application of T. viride within 35 days completely stopped the disease in seedling leaves, while Streptomyces sp. isolates were less effective than the T. viride. Further, there was a diverse range of potential biological control agents for organic apple production. Liu et al. (2017) reported the potential of the endophytes isolated from apple tissues (i.e., Epicoccum, Chaetomium, Biscogniauxia, Neoseptophoma, and Penicillium) for using in the sustainable control of N. ditissima. Chaetomium globosum is one of the most common species utilized in biological control against various phytopathogens such as V. inaequalis (Soytong and Ratanacherdchai, 2005; Zhang et al., 2010). The results of thin-layer chromatography studies reflected the production of different secondary metabolites such as chetomin, BHT, mollicelin G along with chaetoglobosin by this species (Biswas et al., 2012). Several Chaetomium spp. such as Ch. cupreum L.M. Ames and Ch. globosum produce resistance-inducing substances which prevent many plant diseases like Pythium aphanidermatum (Edson) Fitzp. in sugarcane, wilt symptoms in grain seedlings, and apple scab incited by Venturia spp., as well as decreasing tomato Fusarium wilt, and inhibiting the growth of pathogenic Rhizoctonia solani J.G. Kühn and Botrytis spp. (Soytong and Ratanacherdchai, 2005).
Conclusion
In this study, we have identified Co. endophytica 55S2, and Ch. globosum 2S1 as the most potent endophytic fungal isolates for controlling apple scab disease caused by V. inaequalis under greenhouse conditions. These isolates could therefore be considered the best candidates for development of endophytic-based biofungicide and could be integrated as a component in a sustainable integrated apple management strategy for scab. However, further studies are warranted to clearly understand the underlying mechanisms by which the presence of endophytic fungi affect V. inaequalis as well as validate the findings under field conditions on different cultivars of apple.
Data availability statement
The data presented in the study are deposited in the GenBank repository, accession numbers presented in Table 1.
Author contributions
LE designed and directed the research and wrote the manuscript. HE gave advice during experiments. LE and SHR carried out experiments. All authors contributed to the interpretation of the results. All authors contributed to the article and approved the submitted version.
Funding
This work was supported by the Iran National Science Foundation (INSF) (Grant No. 97021003), and University of Tehran, Iran.
Acknowledgments
We gratefully acknowledge the Iran National Science Foundation (INSF), and University of Tehran, Iran, for financial support.
Conflict of interest
The authors declare that the research was conducted in the absence of any commercial or financial relationships that could be construed as a potential conflict of interest.
Publisher’s note
All claims expressed in this article are solely those of the authors and do not necessarily represent those of their affiliated organizations, or those of the publisher, the editors and the reviewers. Any product that may be evaluated in this article, or claim that may be made by its manufacturer, is not guaranteed or endorsed by the publisher.
Supplementary material
The Supplementary material for this article can be found online at: https://www.frontiersin.org/articles/10.3389/fmicb.2022.1024001/full#supplementary-material
References
Adhikari, P., and Pandey, A. (2019). Phosphate solubilization potential of endophytic fungi isolated from Taxus wallichiana Zucc. Roots Rhizosphere 9, 2–9. doi: 10.1016/j.rhisph.2018.11.002
Afandhi, A., Choliq, F. A., Anggrilika, W. S. H., and Tarno, H. (2018). Distribution of the endophytic fungi in apple leaves. Agri 40, 91–100. doi: 10.17503/agrivita.v40i1.1563
Alijani, N., Ghosta, Y., and Rezaiie-Danesh, Y. (2016a). Biodiversity of endophytic fungi from apple trees in West Azerbaijan province. Proceeding of 22nd Iranian Plant Protection Congress 27–30 August. College of Agriculture and Natural Resources, University of Tehran, Karaj, Iran 155.
Alijani, N., Manafi Shabestari, M., and Ghosta, Y. (2016b). Biocontrol effects of endophytic fungi isolated from apple trees against Diplodia bulgarica, the causal agent of apple canker disease. Proceeding of 22nd Iranian Plant Protection Congress, August. College of Agriculture and Natural Resources, University of Tehran, Karaj, Iran.
Arrigoni, E., Antonielli, L., Longa, C. M. O., Angeli, D., Donati, C., Ioriatti, C., et al. (2020). Tissue age, orchard location and disease management influence the composition of fungal and bacterial communities present on the bark of apple trees. Environ. Microbiol. 22, 2080–2093. doi: 10.1111/1462-2920.14963
Berbee, M. L., Pirseyedi, M., and Hubbard, S. (1999). Cochliobolus phylogenetics and the origin of known, highly virulent pathogens, inferred from ITS and glyceraldehyde-3-phosphate dehydrogenase gene sequences. Mycologia 91, 964–977. doi: 10.1080/00275514.1999.12061106
Berger, L. R., and Reynolds, D. M. (1958). The chitinase system of a strain of Streptomyces griseus. Biochim. Biophys. Acta 29, 522–534. doi: 10.1016/0006-3002(58)90008-8
Biswas, S. K., Rashmi, A., Srivastava, K. D., Sangeeta, G., and Prem, D. (2012). Characterization of antifungal metabolites of Chaetomium globosum Kunze and their antagonism against fungal plant pathogens. J. Biol. Control. 26, 70–74. doi: 10.18641/jbc/26/1/45659
Camatti-Sartori, V., Da Silva-Ribeiro, R. T., Valdebenito-Sanhueza, R. M., Pagnocca, F. C., Echeverrigaray, S., and Azevedo, J. L. (2005). Endophytic yeasts and filamentous fungi associated with southern Brazilian apple (Malus domestica) orchards subjected to conventional, integrated or organic cultivation. J. Basic Microbiol. 45, 397–402. doi: 10.1002/jobm.200410547
Cenis, J. L. (1992). Rapid extraction of fungal DNA for PCR amplification. Nucleic Acids Res. 20:2380. doi: 10.1093/nar/20.9.2380
Chen, Y., and Barak, P. (1982). Iron nutrition of plants in calcareous soils. Adv. Agron. 35, 217–240. doi: 10.1016/S0065-2113(08)60326-0
Chen, X., Wang, Y., Gao, Y., Gao, T., and Zhang, D. (2019). Inhibitory abilities of bacillus isolates and their culture filtrates against the gray mold caused by Botrytis cinerea on post-harvest fruit. Plant Pathol. J. 35, 425–436. doi: 10.5423/PPJ.OA.03.2019.0064
Dennis, C., and Webster, J. (1971). Antagonistic propertics of specific group of Trichoderma: production of non-volatile antibiotics. Trans. Br. Mycol. Soc. 57, 25–IN3. doi: 10.1016/S0007-1536(71)80078-5
Doolotkeldieva, T., and Bobusheva, S. (2017). Scab disease caused by Venturia inaequalis on apple trees in Kyrgyzstan and biological agents to control this disease. Adv. Appl. Microbiol. 07, 450–466. doi: 10.4236/aim.2017.76035
Ebrahimi, L., and Fotouhifar, K.-B. (2016). First report of Cyphellophora fusarioides (Chaetothyriales) on a plant host. Sydowia 68, 131–137. doi: 10.12905/0380.sydowia68-2016-0131
Ebrahimi, L., Fotuhifar, K. B., Javan Nikkhah, M., Naghavi, M. R., and Baisakh, N. (2016). Population genetic structure of apple scab (Venturia inaequalis (coo.Ke) G. winter) in Iran. PLoS One 11:e0160737. doi: 10.1371/journal.pone.0160737
Ebrahimi, L., Hatami Rad, S., Ayenekar, T., Agh-Atabay, M. E., Moghimi, H., and Etebarian, H. R. (2021). New records of apple endophytic fungi for the Funga of Iran. Mycologia Iranica 8, 31–39. doi: 10.22043/MI.2022.358420.1214
Ellis, M. B. (1976). More dematiaceous Hyphomycetes. Commonweath Mycological Institute, Kew, England.
Etebarian, H. R., Sholberg, P. L., Eastwell, K. C., and Sayler, R. J. (2005). Biological control of apple blue mold with Pseudomonas fluorescens. Can. J. Microbiol. 51, 591–598. doi: 10.1139/w05-039
Felsenstein, J. (1981). Evolutionary trees from DNA sequences: a maximum likelihood approach. J. Mol. Evol. 17, 368–376. doi: 10.1007/BF01734359
Felsenstein, J. (1985). Confidence intervals on phylogenies: an approach using bootstrap. Evolution 39, 783–791. doi: 10.1111/j.1558-5646.1985.tb00420.x
Gimenez, C., Cabrera, R., Reina, M., and Gonzalez-Coloma, A. (2007). Fungal endophytes and their role in plant protection. Curr. Org. Chem. 11, 707–720. doi: 10.2174/138527207780598765
Golafrouz, H., Safaie, N., and Khelghatibana, F. (2020). The reaction of some apple rootstocks to biocontrol of white root rot Rosellinia necatrix by Trichoderma harzianum in greenhouse. J. Crop Prot. 9, 577–589.
Gond, S. K., Mishra, A., Sharma, V. K., Verma, S. K., Kumar, J., Ravindra, N., et al. (2012). Diversity and antimicrobial activity of endophytic fungi isolated from Nyctanthes arbor-tristis, a well-known medicinal plant of India. Mycoscience 53, 113–121. doi: 10.1007/S10267-011-0146-Z
Hartl, L., Zach, S., and Seidl-Seiboth, V. (2012). Fungal Chitinases: diversity, mechanistic properties, and biotechnological applications. Appl. Microbiol. Biotechnol. 93, 533–543. doi: 10.1007/s00253-011-3723-3
Hsu, S. C., and Lockwood, J. L. (1975). Powdered chitin agar as a selective medium for enumeration of Actinomycetes in water and soil. Appl. Microbiol. 29, 422–426. doi: 10.1128/am.29.3.422-426.1975
Klich, M. A. (2002). Identification of Common Aspergillus Species. Central voor Schimmeltures, Uterch, The Netherlands.
Klich, M. A., and Pitt, J. I. (1988). A Laboratory Guide to the Common aspergillus Species and their Teleomorphs. Commonwealth Scientific and Industrial Research Organization, Division of Food Processing, Australia, Canberra
Kumar, S., Stecher, G., and Tamura, K. (2016). MEGA 7: molecular evolutionary genetics analysis version 7.0 for bigger dataset. Mol. Biol. Evol. 33, 1870–1874. doi: 10.1093/molbev/msw054
Latz, M. A. C., Jensen, B., Collinge, D. B., and Jørgensen, H. J. L. (2018). Endophytic fungi as biocontrol agents: elucidating mechanisms in disease suppression. Plant Ecol. Divers. 11, 555–567. doi: 10.1080/17550874.2018.1534146
Leslie, J. F., and Summerell, B. A. (2006). The Fusarium Laboratory Manual. Blackwell Publishing. Hoboken
Li, H., Dou, M., Wang, X., Guo, N., Kou, P., Jiao, J., et al. (2021). Optimization of cellulase production by a novel endophytic fungus Penicillium oxalicum R4 isolated from Taxus cuspidata. Sustainability 13:6006. doi: 10.3390/su13116006
Li, X., Zhou, J., Xu, R. S., Meng, M., Yu, X., and Dai, C. C. (2018). Auxin, cytokinin, and ethylene involved in rice N availability improvement caused by endophyte Phomopsis liquidambari. J. Plant Growth Regul. 37, 128–143. doi: 10.1007/s00344-017-9712-8
Lillbro, M. (2005). Biocontrol of Penicillium roqueforti on grain-acomparison of mode of action of several yeast species Master thesis of the Agriculture Program, Animal Science, performed at the Department of Microbiology. Swedish University of Agricultural Sciences, Uppsala.
Liu, J., Abdelfattah, A., Norelli, J., Burchard, E., Schena, L., Droby, S., et al. (2018). Apple endophytic microbiota of different rootstock/scion combinations suggests a genotype-specific influence. Microbiome 6:18. doi: 10.1186/s40168-018-0403-x
Liu, J., Ridgway, H. J., and Jones, E. E. (2017). Identification of culturable endophytes isolated from apple tissues with antagonism towards Neonectria ditissima. Science protecting plant health. September 23–29. New Zealand.
Lugtenberg, B. J. J., Caradus, J. R., and Johnson, L. J. (2016). Fungal endophytes for sustainable crop production. FEMS Microbiol. Ecol. 92, 1–17. doi: 10.1093/femsec/fiw194
MacHardy, W. E. (1996). Apple Scab: Biology, Epidemiology, and Management. APS Press: St. Paul, Minnesota
Majidi, S., Roayaei, M., and Ghezelbash, G. (2011). Carboxymethyl-cellulase and filter-paperase activity of new strains isolated from Persian gulf. Microbiol. J. 1, 8–16. doi: 10.3923/mj.2011.8.16
Marques, N. P., de Cassia Pereira, J., Gomes, E., da Silva, R., Araújo, A. R., Ferreira, H., et al. (2018). Cellulases and xylanases production by endophytic fungi by solid state fermentation using lignocellulosic substrates and enzymatic saccharification of pretreated sugarcane bagasse. Ind. Crop. Prod. 122, 66–75. doi: 10.1016/j.indcrop.2018.05.022
Meenavalli, B., Rajulu, G., Thirunavukkarasu, N., Suryanarayanan, T. S., Ravishankar, J. P., Gueddari, N. E. E., et al. (2011). Chitinolytic enzymes from Endophytic fungi. Fungal Divers. 47, 43–53. doi: 10.1007/s13225-010-0071-z
Muresan, L. E. (2017). Cultivable bacterial and fungal endophytes from apple tissues and their potential for biological control of Venturia inaequalis Master thesis Environmental Sciences, University of Guelph. Canada
O'Donnell, K., Kistler, H. C., Cigelink, R., and Ploetz, R. C. (1998). Multiple evolutionary origins of the fungus causing Panama disease of banana: concordant evidence from nuclear and mitochondrial gene genealogies. Proc. Nat. Acad. Sci. U. S. A. 95, 2044–2049. doi: 10.1073/pnas.95.5.2044
Olivieri, L., Saville, R. J., Gange, A. C., and Xu, X. (2021). Apple endophyte community in relation to location, scion and rootstock genotypes and susceptibility to European canker. FEMS Microbiol. Ecol. 97, 1–18. doi: 10.1093/femsec/fiab131
Petrini, O. (1991). “Fungal endophyte of tree leaves” in Microbial Ecology of Leaves. eds. J. Andrews and S. S. Hirano (New York: Spring-Verlag)
Rai, M., Rathod, D., Agarkar, G., Dar, M., Brestic, M., Pastore, G. M., et al. (2014). Fungal growth promotor endophytes: a pragmatic approach towards sustainable food and agriculture. Symbiosis 62, 63–79. doi: 10.1007/s13199-014-0273-3
Rinu, K., Pandey, A., and Palni, L. M. S. (2012). “Utilization of psychrotolerant phosphate solubilizing fungi under low temperature conditions of the mountain ecosystem” in Microorganisms in Sustainable Agriculture and Biotechnology. eds. T. Satyanarayana and B. Johri (Dordrecht: Springer)
Rivera-Orduña, F. N., Suarez-Sanchez, R. A., Flores-Bustamante, Z. R., Gracida-Rodriguez, J. N., and Flores-Cotera, L. B. (2011). Diversity of endophytic fungi of Taxus globosa (Mexican yew). Fungal Divers. 47, 65–74. doi: 10.1007/s13225-010-0045-1
Rozpądek, P., Wężowicz, K., Nosek, M., Ważny, R., Tokarz, K., Lembicz, M., et al. (2015). The fungal endophyte Epichloë typhina improves photosynthesis efficiency of its host orchard grass (Dactylis glomerata). Planta 242, 1025–1035. doi: 10.1007/s00425-015-2337-x
Saikkonen, K., Faeth, S. H., Helander, M., and Sullivan, T. J. (1998). Fungal endophytes: a continuum of interactions with host plants. Annu. Rev. Ecol. Syst. 29, 319–343. doi: 10.1146/annurev.ecolsys.29.1.319
Santoyo, G., Moreno-Hagelsieb, G., Orozco-Mosqueda, M. C., and Glick, B. R. (2016). Plant growth-promoting bacterial endophytes. Microbiol. Res. 183, 92–99. doi: 10.1016/j.micres.2015.11.008
Silva, N. I. D. E., Brooks, S., Lumyong, S., and Hyde, K. D. (2018). Use of endophytes as biocontrol agents. Rev. Fungal. Biol. 33, 133–148. doi: 10.1016/j.fbr.2018.10.001
Simmons, E. G. (2007). Alternaria an identification manual. CBS biodiversity series, no. 6. The Netherlands. 775
Singh, B., and Satyanarayana, T. (2011). Microbial phytases in phosphorus acquisition and plant growth promotion. Physiol. Mol. Biol. Plants 17, 93–103. doi: 10.1007/s12298-011-0062-x
Sivanesan, A. (1987). Graminicolous species of Bipolaris, Curvularia, Drechslera, Exserohilum and their teleomorphs. Mycol. Pap. 81:170. doi: 10.2307/3759472
Song, J., Liang, J. F., Mehrabi-Koushki, M., Krisai Reilhuber, I., Ali, B., Kumar Bhatt, V., et al. (2019). Fungal systematics and evolution: FUSE 5. Sydowia 71, 141–245. doi: 10.12905/0380.sydowia71-2019-0141
Soytong, K., and Ratanacherdchai, K. (2005). Application of mycofungicide to control late blight of potato. J. Agric. Technol. 1, 19–32.
Sperber, J. I. (1958). The incidence of apatite-solubilizing organisms in the rhizosphere and soil. Crop Pasture Sci. 9, 778–781. doi: 10.1071/AR9580778
Steel, R. G. D., and Torrie, J. H. (1980). Principles and Procedures of Statistics. McGraw Hill book Co Inc, New York, USA.
Strobel, G. (2018). The emergence of Endophytic microbes and their biological promise. J. Fungi 4:57. doi: 10.3390/jof4020057
Strobel, G., and Daisy, B. (2003). Bioprospecting for microbial endophytes and their natural products. Microbiol. Mol. Biol. Rev. 67, 491–502. doi: 10.1128/MMBR.67.4.491-502.2003
Sutton, B. C. (1980). The Coelomycetes. Fungi Imperfecti with Pycnidia, Acervuli and Stromata. Common Wealth Mycologial Institute, Kew, Surrey, England
Tenzer, I., and Gessler, C. (1997). Subdivision and genetic structure of four populations of Venturia inaequalis in Switzerland. Eur. J. Plant Pathol. 103, 565–571. doi: 10.1023/A:1008636913211
Terhonen, E., Blumenstein, K., Kovalchuk, A., and Asiegbu, F. O. (2019). Forest tree microbiomes and associated fungal endophytes: functional roles and impact on forest health. Forests 10:42. doi: 10.3390/f10010042
Thompson, J. D., Higgins, D. G., and Gibson, T. J. (1994). CLUSTAL W: improving the sensitivity of progressive multiple sequence alignment through sequence weighting, position specific gap penalties and weight matrix choice. Nucleic Acids Res. 22, 4673–4680. doi: 10.1093/nar/22.22.4673
Toghueo, R. M. K., Zabalgogeazcoa, I., Vazquez de Aldana, B. R., and Boyom, F. F. (2017). Enzymatic activity of Endophytic fungi from the medicinal plants Terminalia catappa, Terminalia mantaly and Cananga odorata. South Afr. J. Bot. 109, 146–153. doi: 10.1016/j.sajb.2016.12.021
Vergara, C., Araujo, K. E. C., Urquiaga, S., Schultz, N., de Carvalho Balieiro, F., Medeiros, P. S., et al. (2017). Dark septate endophytic fungi help tomato to acquire nutrients from ground plant material. Front. Microbiol. 8:2437. doi: 10.3389/fmicb.2017.02437
Wenneker, M., and Thomma, B. P. (2020). Latent postharvest pathogens of pome fruit and their management: from single measures to a systems intervention approach. Eur. J. Plant Pathol. 156, 663–681. doi: 10.1007/s10658-020-01935-9
White, T. J., Bruns, T., Lee, S., and Taylor, J. (1990). “Amplification and direct sequencing of fungal ribosomal RNA genes for phylogenetics” in PCR Protocols: A Guide to Methods and Applications. eds. M. A. Innes, D. H. Gelfand, J. J. Sninsky, and T. J. White (New York: Academic Press)
Wilkins, K., Nielsen, K. F., and Din, S. U. (2003). Patterns of volatile metabolites and nonvolatile Trichothecenes produced by isolates of Stachybotrys, Fusarium, Trichoderma, Trichothecium, and Memnoniella. Environ. Sci. Pollut. Res. 10, 162–166. doi: 10.1065/espr2002.05.118
Yang, S. X., Gao, J. M., Zhang, Q., and Laatsch, H. (2011). Toxic polyketides produced by Fusarium sp., an endophytic fungus isolated from Melia azedarach. Bioorg. Med. Chem. Lett. 21, 1887–1889. doi: 10.1016/j.bmcl.2010.12.043
Yang, S. Z., Peng, L. T., Su, X. J., Chen, F., Cheng, Y. J., Fan, G., et al. (2011). Bioassay guided isolation and identification of antifungal components from propolis against Penicillium italicum. Food Chem. 127, 210–215. doi: 10.1016/j.foodchem.2010.12.011
Zhang, J., Ge, H. M., Jiao, R. H., Li, J., Peng, H., Wang, Y. R., et al. (2010). Cytotoxic chaetoglobosins from the endophyte Chaetomium globosum. Planta Med. 76, 1910–1914. doi: 10.1055/s-0030-1249936
Zhang, H. W., Song, Y. C., and Tan, R. X. (2006). Biology and chemistry of endophytes. Nat. Prod. Rep. 23, 753–771. doi: 10.1039/b609472b
Keywords: biocontrol, chitinase activity, cellulase activity, endophyte, metabolites, volatile organic compounds
Citation: Ebrahimi L, Hatami Rad S and Etebarian HR (2022) Apple Endophytic fungi and their antagonism against apple scab disease. Front. Microbiol. 13:1024001. doi: 10.3389/fmicb.2022.1024001
Edited by:
Naser Safaie, Tarbiat Modares University, IranReviewed by:
Jadson Diogo Pereira Bezerra, Universidade Federal de Goiás, BrazilWei Yan, Nanjing Agricultural University, China
Copyright © 2022 Ebrahimi, Hatami Rad and Etebarian. This is an open-access article distributed under the terms of the Creative Commons Attribution License (CC BY). The use, distribution or reproduction in other forums is permitted, provided the original author(s) and the copyright owner(s) are credited and that the original publication in this journal is cited, in accordance with accepted academic practice. No use, distribution or reproduction is permitted which does not comply with these terms.
*Correspondence: Leila Ebrahimi, Le_ebrahimi@ut.ac.ir