- 1College of Life Sciences, Shandong Normal University, Jinan, China
- 2Vector-Borne Virus Research Center, Fujian Province Key Laboratory of Plant Virology, Fujian Agriculture and Forestry University, Fuzhou, China
- 3College of Plant Protection, Fujian Agriculture and Forestry University, Fuzhou, China
- 4School of Forests, Fisheries and Geomatics Sciences, University of Florida, Gainesville, FL, United States
- 5Institute of Microbiology, Czech Academy of Sciences, Prague, Czechia
Fungi of the genus Geosmithia are frequently associated with bark beetles that feed on phloem on various woody hosts. Most studies on Geosmithia were carried out in North and South America and Europe, with only two species being reported from Taiwan, China. This study aimed to investigate the diversity of Geosmithia species in China. Field surveys in Fujian, Guangdong, Guangxi, Hunan, Jiangsu, Jiangxi, Shandong, Shanghai, and Yunnan yielded a total of 178 Geosmithia isolates from 12 beetle species. The isolates were grouped based on morphology. The internal transcribed spacer, β-tubulin, and elongation factor 1-α gene regions of the representatives of each group were sequenced. Phylogenetic trees were constructed based on those sequences. In total, 12 species were identified, with three previously described species (Geosmithia xerotolerans, G. putterillii, and G. pallida) and nine new species which are described in this paper as G. luteobrunnea, G. radiata, G. brevistipitata, G. bombycina, G. granulata (Geosmithia sp. 20), G. subfulva, G. pulverea (G. sp. 3 and Geosmithia sp. 23), G. fusca, and G. pumila sp. nov. The dominant species obtained in this study were G. luteobrunnea and G. pulverea. This study systematically studied the Geosmithia species in China and made an important contribution to filling in the gaps in our understanding of global Geosmithia species diversity.
Introduction
Members of Geosmithia are widely distributed fungal associates of phloem- and xylem-feeding beetles (Pitt, 1979; Kolařík et al., 2007, 2017; Lin et al., 2016), such as species in Bostrichidae and Curculionidae-Scolytinae (Coleoptera) (Juzwik et al., 2015; Kolařík et al., 2017). Geosmithia species are predominantly isolated from phloem-feeding bark beetles on broadleaved and conifer trees although they have been documented from many other substrates including soil (Kolařík et al., 2004), seed-feeding beetles (Huang et al., 2017), animal skin (Crous et al., 2018), indoor environment (Crous et al., 2018), insect-free plant tissues (McPherson et al., 2013), and food materials (Pitt and Hocking, 2009). To date, almost 60 phylogenetic and 21 formally described Geosmithia species have been recognized (Strzałka et al., 2021).
Geosmithia is similar to Penicillium and Paecilomyces in morphology, but it can be distinguished by the combination of stipe with or without a curved basal cell, verrucose conidiophores (including phialide), cylindrical phialide shape with a very short and cylindrical neck (collula), and ellipsoidal or cylindrical conidia (except globose conidia in Geosmithia eupagioceri and G. microcorthyli). The colony color could be in shades of white, yellow, brown, or red but never bluish-green or green (Kolařík et al., 2004; Kolařík and Kirkendall, 2010).
The spores of Geosmithia may be transmitted by attaching to the surface of the beetle vector, but the ecological role of most Geosmithia species in symbiosis with bark beetles is still unclear. Some species serve as the main food source or supplementary nutrition for the beetles (Kolařík and Kirkendall, 2010; Machingambi et al., 2014), but most are probably commensals with minimal or no benefit to the beetle (Veselská et al., 2019) because the vector beetles show neither any apparent morphological adaptation nor nutrient dependence (Huang et al., 2017, 2019). Some Geosmithia species exhibit extracellular antimicrobial and antifungal metabolites, but their ecological implications are unknown (Stodůlková et al., 2009; Veselská et al., 2019).
Some Geosmithia species can cause serious tree diseases. One example is the thousand cankers disease (TCD) of walnuts caused by G. morbida (Kolařík et al., 2011). Following high-density colonization by its beetle vector, the walnut twig beetle (Pityophthorus juglandis), in the phloem of walnut (Juglans spp.) or wingnut (Pterocarya spp.) trees, G. morbida causes numerous small lesions which eventually girdle the vascular tissue (Tisserat et al., 2009; Kolařík et al., 2011; Utley et al., 2012; Seybold et al., 2013; Hishinuma et al., 2015). TCD has affected many walnut trees in North America, especially in the western United States (Tisserat et al., 2009, 2011), and has recently been detected in Europe (Montecchio et al., 2014). Another mildly pathogenic species Geosmithia sp. 41 causes mild pathogenicity in Quercus argifolia (Kolařík et al., 2017), originally reported as G. pallida (Lynch et al., 2014).
After the discovery of the Geosmithia–beetle association (Kirschner, 2001), there has been an accumulation of reports describing Geosmithia fungi from phloem-feeding bark beetles around the world (Kolařík et al., 2004, 2007, 2008; Kubátová et al., 2004; Kolarik et al., 2005; Kolařík and Jankowiak, 2013; McPherson et al., 2013; Jankowiak et al., 2014; Machingambi et al., 2014; Pepori et al., 2015; Huang et al., 2019; Strzałka et al., 2021). Fungal communities associated with phloem-infected bark beetles are formed by a variety of biological and abiotic factors. The tree host is one of the most important selection factors (Skelton et al., 2018). Like other beetle-vectored fungi such as the ophiostomatoid fungi (Seifert et al., 2013), Geosmithia species display variable degrees of specificity to their beetle vectors and tree hosts, ranging from generalists to single-species specialists (Kolařík et al., 2007, 2008; Kolařík and Jankowiak, 2013; Jankowiak et al., 2014; Veselská et al., 2019). Other factors affecting the fungal community structure include beetle ecology, surrounding host tree community, and climatic factors (Six and Bentz, 2007; Jankowiak et al., 2014). These factors also influence the communities of Geosmithia, most notably by the fact that different beetles infesting the same host tree have similar Geosmithia assemblages (Kolařík et al., 2008; Machingambi et al., 2014).
At present, most of the studies of Geosmithia were conducted in North and South America and Europe, but the mycoflora of Asian bark beetles remain understudied. This study investigated the Geosmithia species from China using phylogenetic analyses and morphological and physiological features, and nine Geosmithia new species are described to fill the gap in our understanding of the global Geosmithia diversity.
Materials and Methods
Sampling, Isolating, and Preserving Fungal Isolates
The beetle gallery samples were collected in Fujian, Guangdong, Guangxi, Hunan, Jiangsu, Jiangxi, Shandong, Shanghai, and Yunnan Province from plant hosts of Altingia gracilipes (Altingiaceae), Gnetum luofuense (Gnetaceae), Lauraceae sp., Liquidambar formosana (Altingiaceae), L. styraciflua (Altingiaceae), Eriobotrya japonica (Rosaceae), Acacia pennata (Mimosaceae), Rhus chinensis (Anacardiaceae), Cupressus funebris (Cupressaceae), and Ulmus spp. (Ulmaceae) and kept individually in sealable bags (Table 1). The adult beetles were individually placed in Eppendorf tubes. Both galleries and adult beetles were kept at 4°C for further isolation. The beetle vectors included three groups: (1) Curculionidae-Scolytinae: Acanthotomicus suncei, Scolytus jiulianshanensis (Curculionidae-Scolytinae), S. semenovi, Microperus sp. L589, Cryphalus eriobotryae, C. kyotoensis, and Phloeosinus sp. and P. cf. hopehi, (2) Curculionidae-Platypodinae: Crossotarsus emancipates, and (3) Bostrichidae: Dinoderus sp. L489, Sinoxylon cf. cucumellae and Xylocis tortilicornis (Table 1). The fungal isolates were obtained by scraping wood tissue from the beetle galleries and crushing the beetle coating. The isolates were inoculated on 2% malt extract agar [MEA: 20 g agar (Solarbio, China), 20 g malt extract (Hopebio, China), and 1 L deionized water] amended with 0.05% streptomycin. The cultures were purified by hyphal-tip subculturing and incubated at 25°C. All the cultures obtained in this study were deposited in the culture collection (SNM) of Shandong Normal University, Jinan, Shandong Province, China. Isolates linked to type specimens of the fungal species were deposited in the China General Microbiological Culture Collection Center (CGMCC), Beijing, China. The holotype specimens (dry cultures) were deposited in the Herbarium Mycologicum, Academiae Sinicae (HMAS), Beijing, China (Table 2).
DNA Extraction, Amplification, and Sequencing
DNA was extracted by scraping fresh fungal tissue from pure cultures and adding to 50 μl extraction solution of the PrepMan Ultra Sample Preparation Reagent (Applied Biosystems, Foster City, CA, United States). The samples were vortexed for 1 min, incubated at 100°C for 10 min, and then centrifuged at 5,000 rpm (MiniSpin Plus Centrifuge, Eppendorf 5453, Germany) for 5 min. The supernatant was transferred to a new Eppendorf tube and used as the template for polymerase chain reaction (PCR) amplification.
The rDNA region of ITS1-5.8S-ITS2, internal transcribed spacer (ITS), was amplified using the primer pair of ITS1-F (Gardes and Bruns, 1993) and ITS4 (White et al., 1990). The translation elongation factor 1-α gene (TEF1-α) was amplified using the primer pair of EF1-983F and EF1-2218R (Rehner and Buckley, 2005). β-Tubulin (TUB2) was amplified by using T10 and Bt2b (Glass and Donaldson, 1995; O’Donnell and Cigelnik, 1997). The second-largest subunit of the RNA polymerase II gene (RPB2) was amplified using the primer pair of fRPB2-5F/fRPB2-7cR (Liu et al., 1999). The PCR amplifications were carried out in a final 25-μl PCR reaction mixture consisting of 50–100 ng template DNA, 1.25 U Taq polymerase (Vazyme Biotech Co., Ltd., Nanjing, China), 200 μM dNTP, 0.5 μM of each primer, and 5% (v/v) dimethyl sulfoxide. The PCR conditions were as follows: 95°C for 3 min, followed by 30 cycles of 95°C for 1 min, 55°C for 1 min, and 72°C for 1 min. The final extension step was 72°C for 10 min. The amplified products were sequenced in Sangon Biotech, Qingdao, Shandong Province, China.
DNA Sequence Analyses
The sequences obtained using the forward and reverse primers were aligned in Geneious version 10.2.2 (Biomatters, Auckland, New Zealand). The reference sequences of Geosmithia species were retrieved from GenBank (Table 2). Emericellopsis pallida CBS 490.71 was chosen as the phylogenetic outgroup. The sequences were aligned by using the online version of MAFFT v. 7 (Katoh and Standley, 2013) with the default settings. The best nucleotide substitution model for each partition was determined in jModelTest v. 2.1.1 (Darriba et al., 2012). Maximum parsimony (MP) analyses were performed using MEGA v.10.2.0 with 1,000 bootstrap replicates; gaps were treated as a fifth-state character. Maximum likelihood (ML) phylogenetic analyses were conducted in the CIPRES Science Gateway (Miller et al., 2010) using RAxML v. 8.2.2 (Stamatakis, 2014) with the recommended partition parameters to assess the tree topology and bootstrap values from 1,000 replicate searches. Bayesian inference (BI) was estimated in the CIPRES Science Gateway (Miller et al., 2010) using MrBayes 3.2.7a (Ronquist et al., 2012). The MCMC runs of four chains were executed simultaneously from a random starting tree for 20 million generations, and every 100 generations were sampled, resulting in 200,000 trees. Chain convergence was determined with Tracer 1.71, and the effective sample size values over 200 are considered adequate. A total of 50,000 trees were discarded during burn-in. Posterior probabilities were estimated from the retained 150,000 trees. Phylogenetic trees were visualized and edited in FigTree v. 1.4.3. The final alignments used in this study have been submitted to TreeBase2 (nos.: 28242).
Morphological Study
Morphological characters were observed and recorded using Olympus BX61 microscope (Olympus Corporation, Tokyo, Japan). The images were analyzed using ImageJ3. At least 50 measurements for each of the structures were measured. The results of the calculation are expressed as (minimum -) mean minus standard deviation -- mean plus standard deviation -- (- maximum). One-way ANOVA in SPSS version 26.04 was used to evaluate the morphological differences of the different species, with a significance level of 0.05 (Supplementary Figure S5).
Growth Study
Three independently isolated strains of each novel taxon were randomly selected for the growth experiments. The actively growing edge mycelia were inoculated at the center of 90-mm Petri dishes containing 2% MEA and incubated in darkness at temperatures ranging from 5 to 35°C at 5°C intervals and 37°C for 8 days; each temperature had three duplicates. The colony diameters were measured every 2 days, and then the optimum temperature of growth for each species and the high- and low-temperature conditions of growth were calculated.
Results
In total, 125 samples (N) were collected (Table 1). A total of 178 strains in the genus Geosmithia were isolated from 12 beetle species and their galleries. One hundred fifty-eight strains were from the galleries and 20 strains were from the beetles. There were 63 strains from Jiangxi, 47 from Guangdong, 23 from Shandong, 20 from Yunnan, 9 from Shanghai, 8 from Jiangsu, 5 from Fujian, 2 from Guangxi, and 1 from Hunan (Table 1).
Phylogenetic Analysis
The preliminary classification was carried out by BLAST on NCBI GenBank using the ITS marker (Supplementary Table S1). Subsequently, 32 representative strains were selected for multi-gene phylogenetic analysis, and 24 strains were screened for morphological studies (Table 2). The aligned sequences, including gaps, yielded 555 characters for ITS where 124 were parsimony informative, 899 characters for TEF1-α where 209 were parsimony informative, 1,066 characters for RPB2 where 380 were parsimony informative, and 653 characters for TUB2 where 321 were parsimony informative. The concatenated dataset comprised 162 sequences covering 3,173 characters where 1,028 were parsimony informative. The final average standard deviation of split frequency of MCMC analysis was 0.009591 for the concatenated dataset, 0.004862 for ITS, 0.006573 for TEF1-α, 0.008026 for RPB2, and 0.007595 for TUB2. The best substitution model for ITS, TEF1-α, RPB2, TUB2, and combined alignment was GTR + I + G. For all datasets (ITS, TUB2, TEF1-α, and RPB2), ML, MP, and Bayesian inference produced nearly identical topologies, with slight variations in the statistical support for each of the individual sequence datasets (Figure 1 and Supplementary Figures S1–S4). Phylograms obtained by ML are presented for all the individual datasets.
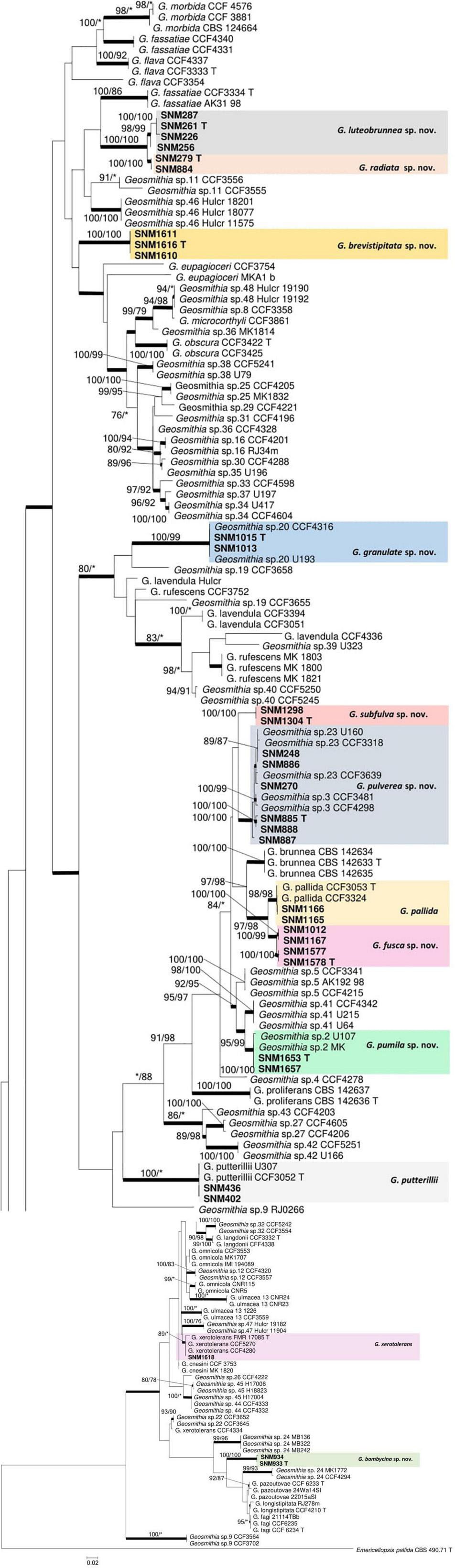
Figure 1. Maximum likelihood (ML) tree of Geosmithia generated from the combined ITS, TEF1-α, TUB2, and RPB2 sequence data. The sequences generated from this study are printed in bold. The bold branches indicate posterior probability values ≥0.9. Bootstrap values of ML/maximum parsimony ≥75% are recorded at the nodes. T, ex-type isolates.
Morphological Statistical Analysis
The results of the morphological comparison of the different species are presented in Supplementary Figure S5. The values are mean of 50 measurements (±) SD, and significant differences according to Dunnett-t3′ multiple-range tests at p < 0.05 levels were indicated and followed by different letters.
Taxonomy
Among the 178 strains obtained in this study, 12 species were identified. Nine of these species are new to science and are described as follows:
Geosmithia luteobrunnea R. Chang and X. Zhang, sp. nov.
MycoBank MB839256
Etymology: luteobrunnea, referring to the yellowish-brown appearance of the colony on MEA.
Diagnosis: The stipe of G. luteobrunnea is slightly thicker and shorter than that in other species. Geosmithia luteobrunnea can grow at 5 and 35°C, even grows slowly at 37°C (Figure 2).
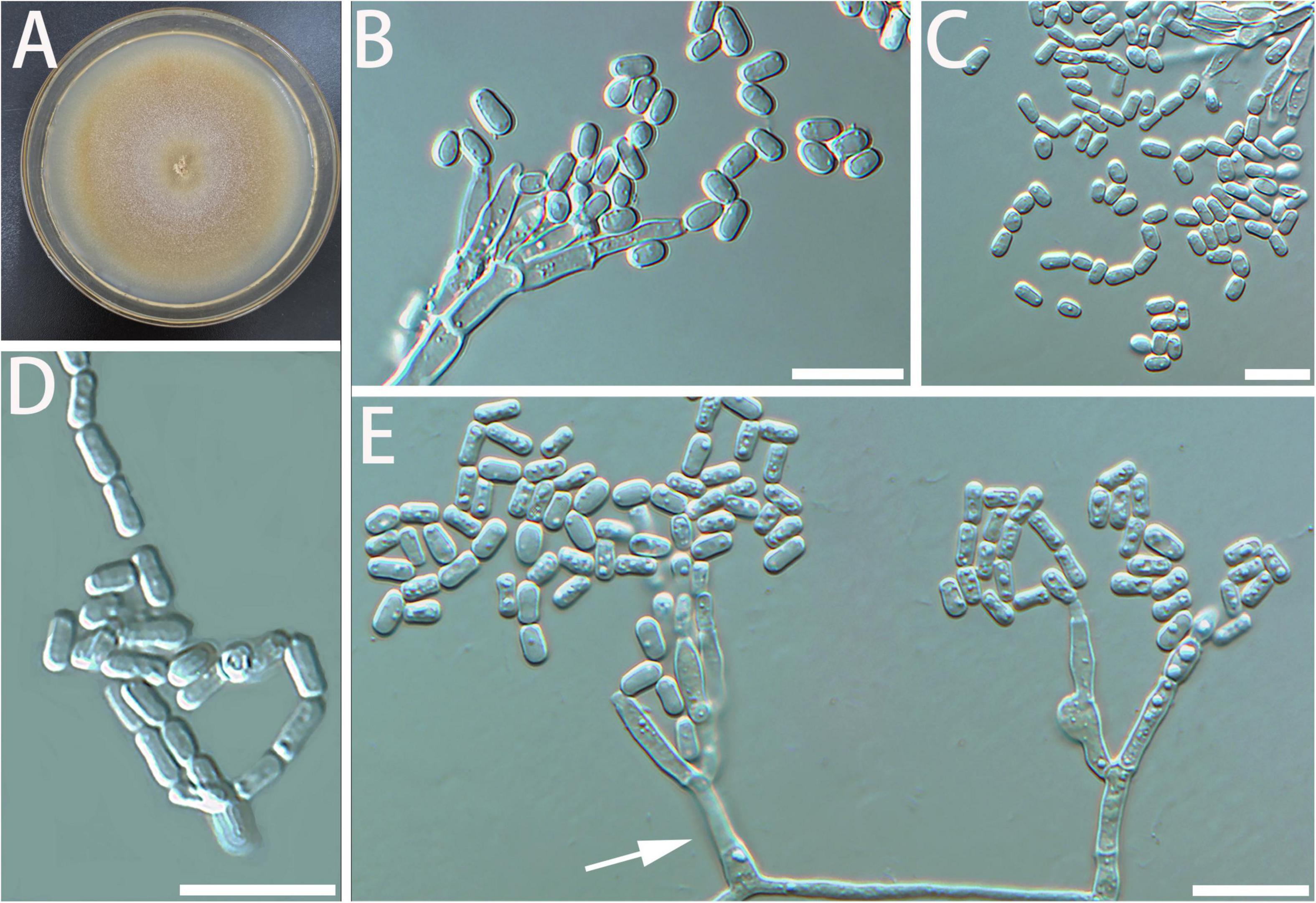
Figure 2. Morphological characteristics of Geosmithia luteobrunnea sp. nov. (SNM261 = CGMCC3.20252, SNM226, SNM287). (A) Eight-day-old culture on 2% malt extract agar. (B–E) Conidiophores and conidia. The stipe (indicated with arrows) is thick and short. Scale bars: 10 μm (B–E).
Type: China, Jiangxi Province, Ganzhou City, Longnan County, Jiulianshan National Nature Reserve (24°34′1′′ N, 114°30′ E), from the gallery of Scolytus jiulianshanensis on Ulmus sp., 5 May 2020, S. Lai, Y. Xu, S. Liao, Y. Wen and T. Li (HMAS 249919 – holotype, SNM261 = CGMCC3.20252 – ex-holotype culture).
Description: Sexual state not observed. Asexual state penicillium-like and (19.0–) 29.6–61.5 (–85.0)-μm long. Conidiophores borne mostly from aerial fungal hyphae, erect, determinate, solitary, sometimes funiculose, with all parts verrucose; base often consisting of a curved and atypically branched cell, stipe (6.4–) 11.3–40.1 (–78.4)-μm long, (1.5–) 1.7–3.2 (–6.0)-μm wide; penicillus, monoverticillate to terverticillate (penicilli of conidiophores on aerial funiculose mycelia are monoverticillate or biverticillate), symmetric or asymmetric, often irregularly branched, rami (1st branch) in whorls of 1–3, (4.1–) 5.2–7.0 (–8.7) × (1.2–) 1.7–2.5 (–3.2) μm, metulae (last branch) in whorls of 1 to 2, (4.0–) 4.9–6.5 (–7.6) × (1.4–) 1.8–2.3 (–2.6) μm; phialides in whorls of 1–3, cylindrical, without or with short cylindrical neck and smooth to verrucose walls, (4.2–) 5.1–7.5 (–10.2) × (1.1–) 1.5–2.3 (–2.7) μm. Conidia hyaline to subhyaline, smooth, narrowly cylindrical to ellipsoidal, (2.3–) 2.9–4.0 (–4.7) × (0.9–) 1.2–1.7 (–2.2) μm, produced in non-persistent conidial chains. Substrate conidia absent.
MEA, 8 days: Colony diameter 50–64 mm at 20°C, 58–78 mm at 25°C, and 44–70 mm at 30°C. The hyphae grow slowly at 5 and 35°C. After 8 days of culture, the colony diameter was 1.5–4 mm and 11–14 mm, respectively. The optimal temperature for growth was 25°C. Colonies at 25°C, 8 days, were oppressed, velutinous, or floccose with raised mycelial cords; colony margin smooth, filamentous, diffuse; aerial mycelium sparse; substrate mycelium sparse; conidiogenesis moderate; milky white to light yellow; reverse lighter brown; absence of exudate; no soluble pigment. When incubated at 35°C, colonies were raised, slightly depressed at the center, rugose, or irregularly furrowed; margin undulate somewhat erose; aerial mycelia sparse to moderate; substratum mycelia dense, forming a tough basal felt; the colony was darker and yellowish-brown; reverse brown; soluble pigment was brown. MEA, 37°C, 8 days, germinating only.
Host: Liquidambar formosana, Liquidambar styraciflua, Ulmus sp.
Beetle vectors: Acanthotomicus suncei, Scolytus jiulianshanensis.
Distribution: Currently only known from Jiangxi and Shanghai.
Notes: Geosmithia luteobrunnea and G. radiata are phylogenetically close to each other on ITS, TUB2, RPB2, TEF1-α trees, and combined alignment tree (Figure 1 and Supplementary Figures S1–S4). The colony morphology of G. luteobrunnea and G. radiata are also similar, but there are many differences among those two species. First of all, their sequences are different (Table 3). Then, under the microscope, the morphological differences between them are more obvious (Supplementary Figure S5). The spore of G. radiata is shorter than the other specie. The stipe of G. radiata is thicker than the other specie, and the stipe of G. luteobrunnea is slightly shorter than the other two species (Supplementary Figure S5). Moreover, their growths at different temperatures are also different (Table 4). G. luteobrunnea can grow at both temperatures, especially at 35°C, even grows slowly at 37°C. Geosmithia radiata only grows a little at 5°C and grows slowly at 35°C. The growth speed of G. luteobrunnea is faster than that of G. radiata (Table 4). Geosmithia luteobrunnea and G. radiata form a species group outstanding by cream to yellow or brown color of sporulation accompanied by the darker (brownish to rusty) shades of the substrate mycelium and colony reverse. This feature is shared also by the phylogenetically related Geosmithia sp. 11 (Kolařík et al., 2007) which is known from Europe and the Mediterranean (Kolařík et al., 2007, 2008) and seems to be diagnostic for the whole species group.
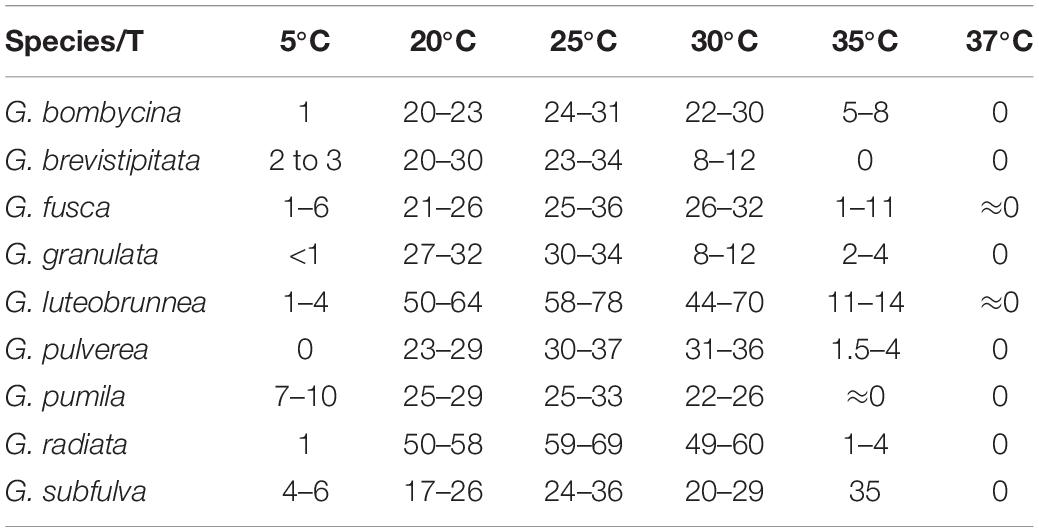
Table 4. The colony diameter of G. subfulva, G. bombycine, G. luteobrunnea, G. radiata, G. granulate, and G. pallida species complex, G. brevistipitata and G. pumila, at different temperatures after 8 days on malt extract agar medium (unit: millimeter).
Additional cultures examined: China, Shanghai, from the gallery of Acanthotomicus suncei on Liquidambar styraciflua, April 2019, L. Gao (SNM226, SNM287).
Geosmithia radiata R. Chang and X. Zhang, sp. nov.
MycoBank MB839257
Etymology: radiata, referring to the radial appearance of the colony on MEA.
Diagnosis: The spore and the stipe of G. radiata are thicker than closely related species. Geosmithia radiata only grows a little at 5 and 35°C (Figure 3).
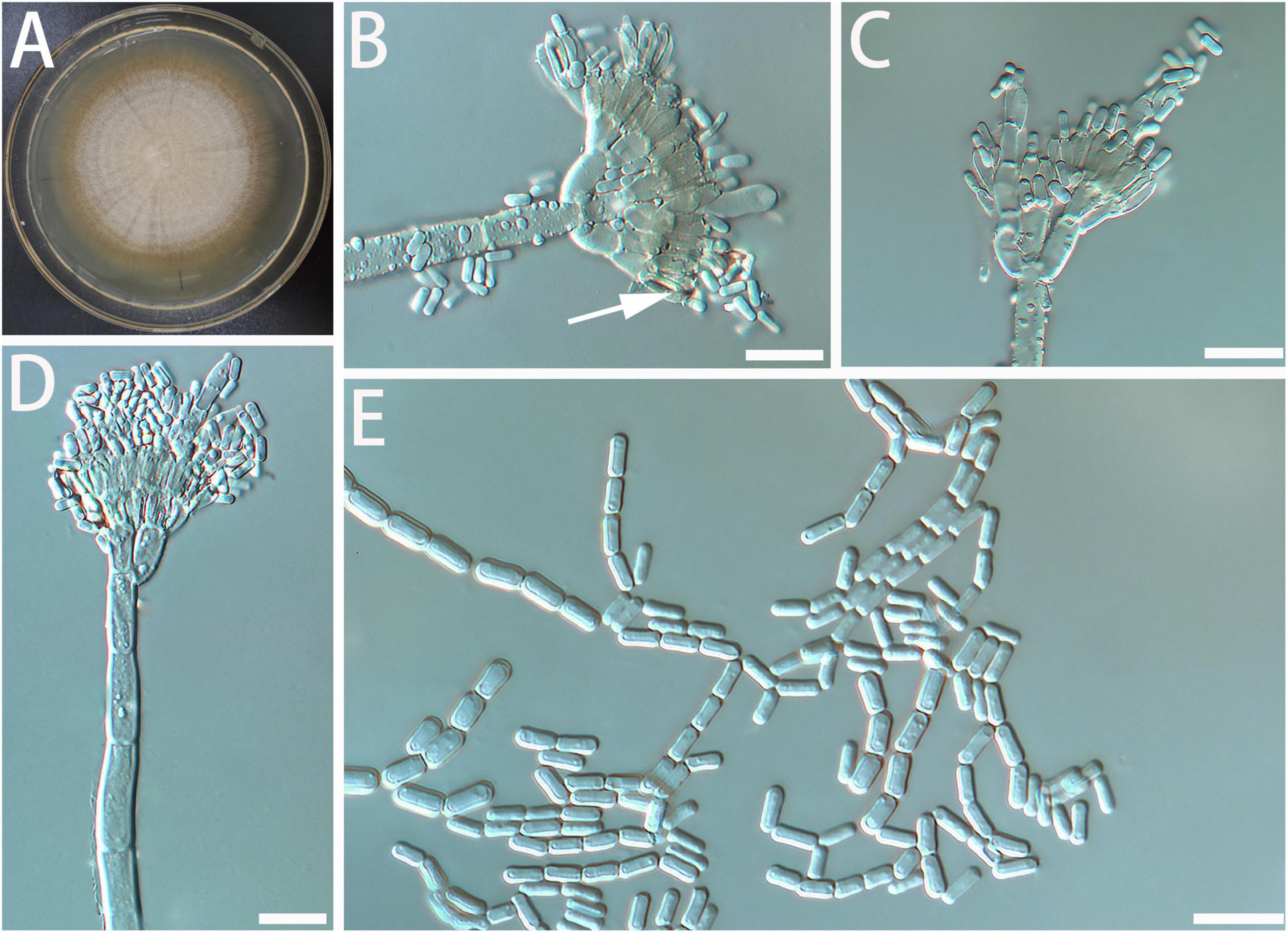
Figure 3. Morphological characteristics of Geosmithia radiata sp. nov. (SNM279 = CGMCC3.20253, SNM884). (A) Eight-day-old culture on 2% malt extract agar. (B–E) Conidiophores and conidia. The sporulation structure is coarse, and the phialides (indicated with arrows) are abundant and compact. Scale bars: 10 μm (B–D) and 20 μm (E).
Type: China, Jiangxi Province, Ganzhou City, Longnan County (24°5′2.4′′ N, 114°47′2.4′′ E), from the gallery of Acanthotomicus suncei on Liquidambar formosana, 5 May 2020, S. Lai (HMAS 249920 – holotype, SNM279 = CGMCC3.20253 – ex-holotype culture).
Description: Sexual state not observed. Asexual state penicillium-like and (22.6–) 35.6–85.7 (–119.3)-μm long. Conidiophores borne from the substrate or aerial hyphae, sometimes arising laterally from another conidiophore, erect, determinate, solitary, with all parts verrucose; stipe commonly (7.3–) 18.4–63.6 (–115.8)-μm long, (1.6–) 2.1–3.8 (–5.9)-μm wide, penicillus, with walls thick, septate; penicillus terminal, monoverticillate, biverticillate, or terverticillate, mostly symmetrical, rami (1st branch) in whorls of 2 to 3, (4.2–) 5.2–7.8 (–10.6) × (1.3–) 2.1–3.5 (–4.8) μm; metulae (last branch) in whorls of 1 to 2, (2.6–) 3.9–5.8 (–7.3) × (1.3–) 1.7–2.6 (–3.3) μm. Phialides in whorls of 1–5, (3.9–) 4.6–6.2 (–7.7) × (1.5–) 1.9–2.8 (–3.9) μm, cylindrical, without or with short cylindrical neck and smooth to verrucose walls. Conidia cylindrical to ellipsoidal, smooth, hyaline to subhyaline, (2.2–) 2.5–3.2 (–4.0) × (0.9–) 1.1–1.5 (–1.8) μm, formed in non-persistent conidial chains. Substrate conidia absent.
MEA, 8 days: Colony diameter 50–58 mm at 20°C, 59–69 mm at 25°C, and 49–60 mm at 30°C. The hyphae grow slowly at 5 and 35°C. After 8 days of culture, the colony diameter was only 1 and 1–4 mm, respectively. The optimal temperature for growth is 25°C. Colonies at 25°C, 8 days, plane, slightly raised centrally, velutinous, with a slight overgrowth of aerial mycelium, with floccose and funiculose areas; substrate mycelium darker, aerial mycelium hyaline; sporulation moderate to heavy, pale cream; vegetative mycelium hyaline; reverse lighter yellow; soluble pigment and exudate absent. When incubated at 35°C, colonies were rising, slightly sunken in the center, furrowed, or irregularly fringed; the substratum hyphae were dense and formed a tough basal felt. The colony is dark and yellowish-brown. MEA, 37°C, 8 days: no growth.
Host: Liquidambar formosana, Ulmus sp.
Beetle vectors: Acanthotomicus suncei, Scolytus jiulianshanensis.
Distribution: Jiangxi.
Notes: See comparisons between Geosmithia luteobrunnea, G. radiata below the description of G. luteobrunnea.
Additional cultures examined: China, Jiangxi Province, Ganzhou City, Xunwu County (24°57′ N, 115°38′2′′ E), from the gallery of Acanthotomicus suncei on Liquidambar formosana, 5 May 2020 (SNM884).
Geosmithia brevistipitata R. Chang and X. Zhang, sp. nov.
MycoBank MB841503
Etymology: brevistipitata, referring to the short conidiophore stipe, a character distinguishing it from other members of the species complex.
Diagnosis: Isolates of G. brevistipitata formed a monophyletic clade on all the phylogenetic trees (Figure 4).
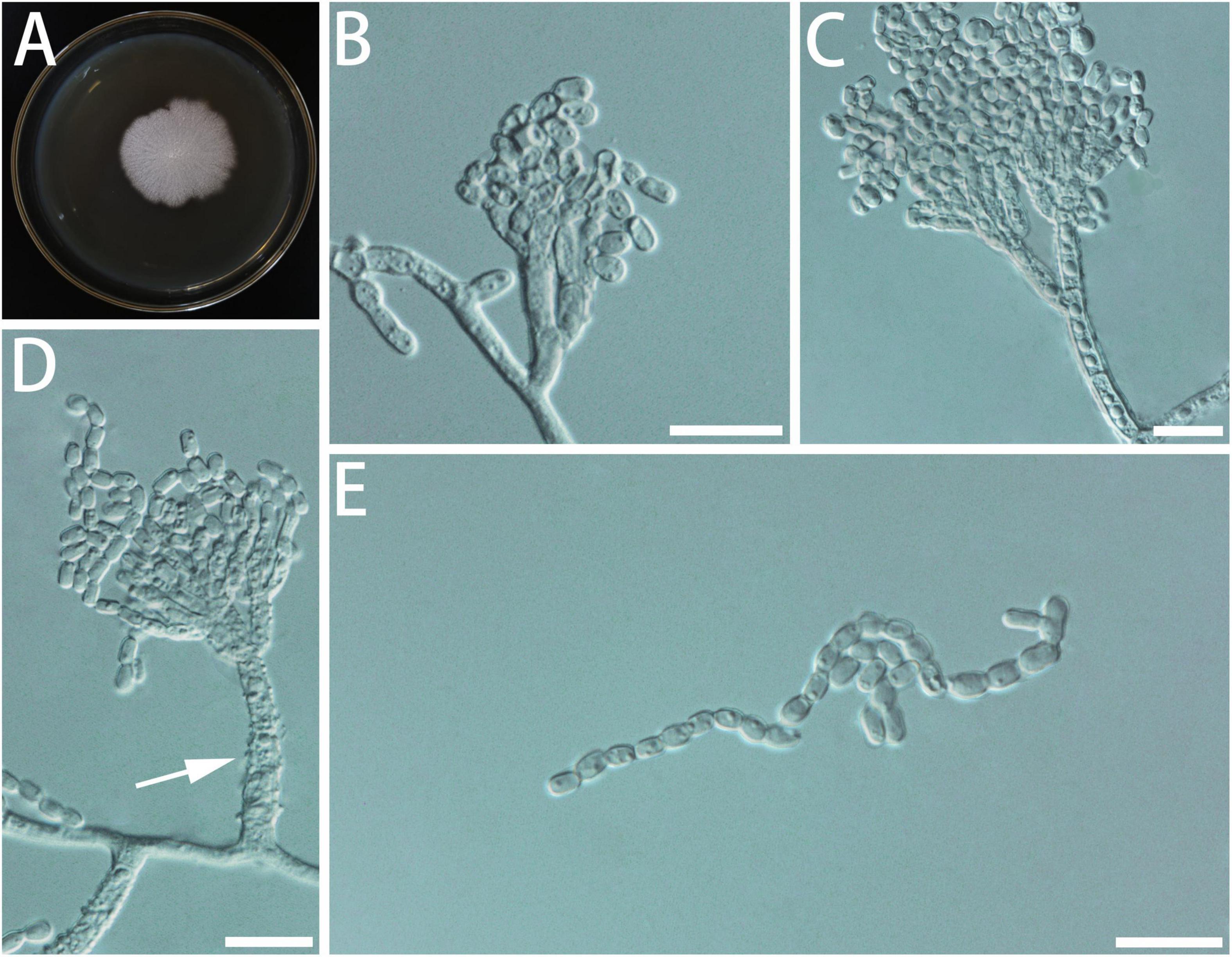
Figure 4. Morphological characteristics of Geosmithia brevistipitata sp. nov. (SNM1616 = CGMCC3.20627, SNM1610, SNM1611). (A) Eight-day-old culture on 2% malt extract agar. (B–E) Conidiophores and conidia. The stipe (indicated with arrows) is short and sometimes not smooth. Scale bars: 10 μm (B–E).
Type: China, Shandong Province, Linyi City, Tianfo scenic spot (35°5′ N, 118°2′ E), from the gallery of Phloeosinus cf. hopehi on Cupressus funebris, 8 August 2021, Y. Cao (HMAS 351566 - holotype, SNM1616 = CGMCC3.20627 – ex-holotype culture).
Description: Sexual state not observed. Asexual state penicillium-like and (9.5–) 15.5–42.3 (–77.9)-μm long. Conidiophores borne from the substrate or aerial hyphae, sometimes arising laterally from another conidiophore, erect, determinate, solitary, with all parts verrucose; stipe commonly (2.9–) 7.5–30.0 (–56.0) × (1.3–) 1.9–3.0 (–4.1) μm, penicillus, with walls thick, septate; penicillus terminal, monoverticillate or biverticillate, mostly symmetrical, metulae in whorls of 2–3, (4.6–) 6.3–9.1 (–11.2) × (1.8–) 2.0–2.7 (–3.2) μm. Phialides in whorls of 2–5, (3.2–) 5.0–8.7 (–11.4) × (1.3–) 1.7–2.4 (–2.8) μm, cylindrical, without or with short cylindrical neck and smooth to verrucose walls. Conidia cylindrical to ellipsoidal, smooth, hyaline to subhyaline, (2.2–) 2.4–3.1 (–3.8) × (1.2–) 1.5–1.9 (–2.2) μm, formed in non-persistent conidial chains. Substrate conidia absent.
MEA, 8 days: Colony diameter 24–30 mm at 20°C, 23–34 mm at 25°C, and 8–12 mm at 30°C. The hyphae grow slowly at 5°C. After 8 days of culture, the colony diameter was only 2–3 mm. No growth at 35°C. The optimal temperature for growth is 20–25°C. Colonies at 25°C, 8 days, plane, granular, with a slight growth of aerial mycelium; substrate mycelium white; reverse white; sporulation moderate white; soluble pigment and exudate absent. MEA, 37°C, 8 days: no growth.
Host: Cupressus funebris.
Beetle vectors: Phloeosinus cf. hopehi.
Distribution: Shandong.
Notes: Isolates of G. brevistipitata formed a monophyletic clade on both ITS, TUB2, TEF1-α, and RPB2 trees. Its closely related species differ on various trees, such as according to ITS tree, isolates of G. brevistipitata were closely related to G. cnesini, G. xerotolerans, G. omnicola, G. ulmacea, and Geosmithia sp. 12 (Supplementary Figure S5), but according to the TUB2 tree, isolates of G. brevistipitata were connected to other species, e.g., G. microcorthyli and G. obscura (Supplementary Figure S2). Among the other species described, it is outstanding by the combination of slow growth and white colony color and short stipe.
Additional cultures examined: China, Shandong Province, Linyi City, Tianfo scenic spot (118°2′ N, 35°5′ E), from the gallery of Phloeosinus cf. hopehi on Cupressus funebris, 8 August 2021, Y. Cao (SNM1610).
Geosmithia granulata R. Chang and X. Zhang, sp. nov.
MycoBank MB 840646
Etymology: granulata, referring to the granular appearance of the colony on MEA.
Diagnosis: The conidia of G. granulata are shorter than the closely related species (Figure 5).
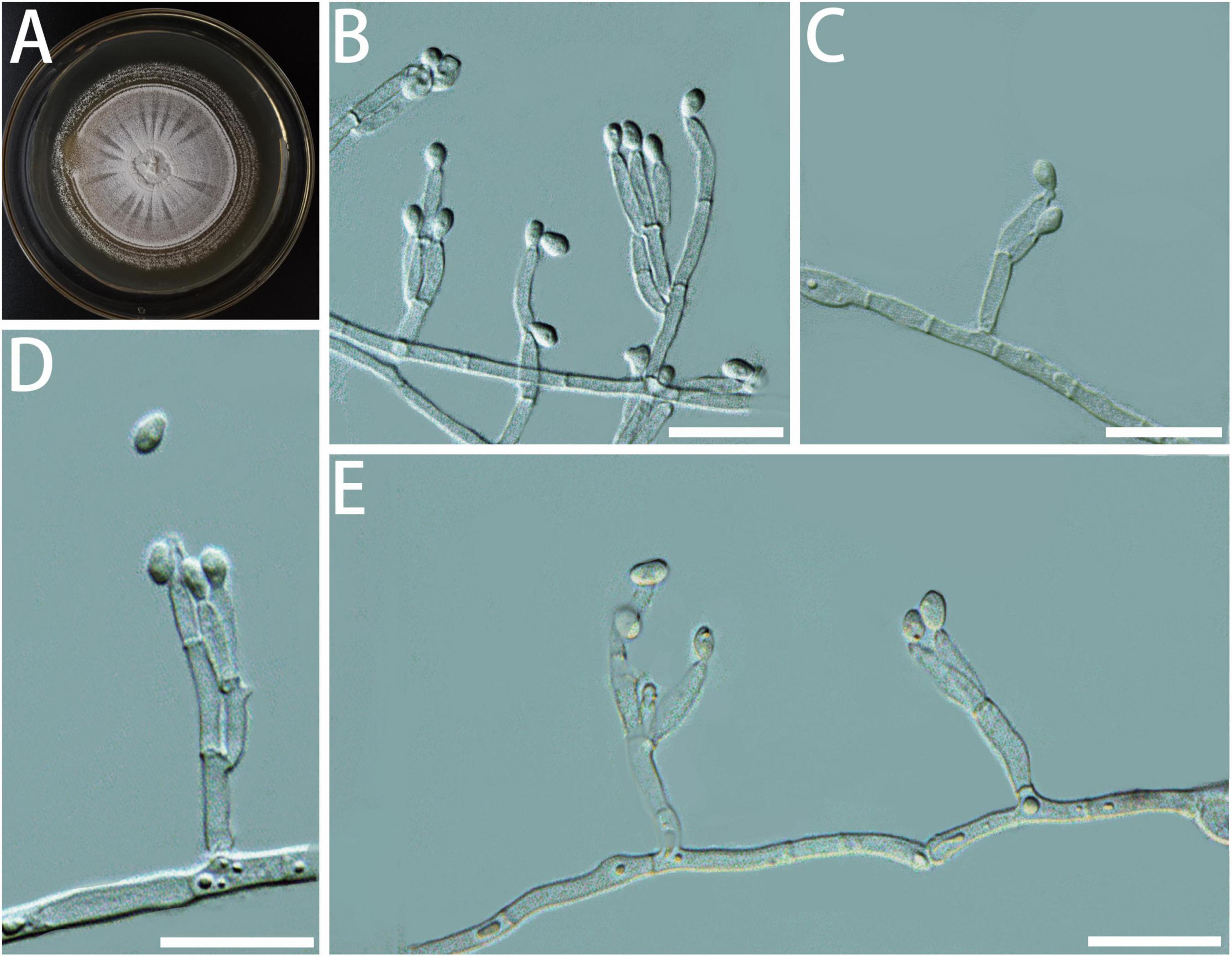
Figure 5. Morphological characteristics of Geosmithia granulata sp. nov. (SNM1015 = CGMCC3.20450, SNM1013). (A) Eight-day-old culture on 2% malt extract agar. (B–E) Conidiophores and conidia. Conidia hyaline, smooth, wide oval shape, like an egg. Scale bars: 10 μm (B–E).
Type: China, Yunnan Province, Xishuangbanna City, Xishuangbanna Botanical Garden (21°55′1′′ N, 101°16′1′′ E), from the gallery of Sinoxylon cf. cucumella on Acacia pennata, 1 May 2021, Y. Dong and Y. Li (HMAS 351568 - holotype, SNM1015 = CGMCC3.20450 – ex-holotype culture).
Description: Sexual state not observed. Asexual state penicillium-like, (9.6–) 11.6–26.0 (–50.6) μm in length. Conidiophores emerging from hyphae, smooth, septate; stipe (4.0–) 4.8–8.3 (–14.3) × (1.1–) 1.4–2.1 (–2.6) μm; penicilli typically longer than the stipe, terminal, monoverticillate, biverticillate, or terverticillate, symmetric or asymmetric, often irregularly branched, rarely more; metulae in whorls of 1–2, (5.2–) 5.7–8.1 (–11.3) × (1.0–) 1.3–1.7 (–2.0) μm; phialides in whorls of 1–4, smooth, (3.3–) 4.9–7.1 (–8.8) × (1.0–) 1.3–1.8 (–2.2) μm. Conidia hyaline, smooth, wide oval shape, like an egg, (1.5–) 1.8–2.2 (–2.5) × (0.8–) 1.0–1.4 (–1.8) μm. Conidia formed in long, non-persistent conidial chains. Substrate conidia absent.
MEA, 8 days: Colony diameter 27–32 mm at 20°C, 30–34 mm at 25°C, and 8–12 mm at 30°C. At 5°C: germinating only. At 35°C, the mycelia grew slowly. After 8 days of culture, the diameter of the colony was 2–4 mm. The optimal growth temperature is 20–25°C. At 25°C, 8 days: Colonies were flat and radiated, surface texture powdery; central hyphae slightly raised and wrinkled, conidiogenesis heavy; marginal colonies were similar to annual rings, slightly flocculent, hyphae were sparse, milky white, reverse creamyrice white; without exudate and insoluble pigment. MEA, 37°C, 8 days, no growth.
Host: Acacia pennata, Hibiscus tiliaceus, Ulmus sp.
Beetle vectors: Sinoxylon cf. cucumella, Ernoporus japonicus, Scolytus semenovi.
Distribution: Guangdong, Jiangsu, Yunnan.
Notes: According to the tree made by ITS and TEF1-α sequence, SNM1015 and SNM1013 were clustered with Geosmithia sp. 20 (Supplementary Figures S1, S3). TUB2 and RPB2 sequences of Geosmithia sp. 20 were not available on GenBank; therefore, Geosmithia sp. 20 was not included in TUB2 and RPB2 trees. These results suggested that our isolates and Geosmithia sp. 20 belonged to the same species, described as G. granulata sp. nov. This extends the geographical distribution of this species to the Mediterranean Basin (Kolařík et al., 2007) and western part of the United States (Kolařík et al., 2017) where it was found in association with many bark beetle species feeding on plants from the families Asteraceae, Fabaceae, Moraceae, Oleaceae, Ulmaceae (Mediterranean Basin), or Cupressaceae, Ulmaceae (Western United States).
Additional cultures examined: China, Yunnan Province, Xishuangbanna City, Xishuangbanna Botanical Garden (21°55′1′′ N, 101°16′1′′ E), from the gallery of Sinoxylon cf. cucumella on Acacia pennata, 1 May 2021, Y. Dong and Y. Li (SNM1013).
Geosmithia subfulva R. Chang and X. Zhang, sp. nov.
MycoBank MB 841505
Etymology: subfulva, referring to the beige appearance of the colony on MEA.
Diagnosis: Isolates of G. subfulva formed a monophyletic clade on all the phylogenetic trees (Figure 6).
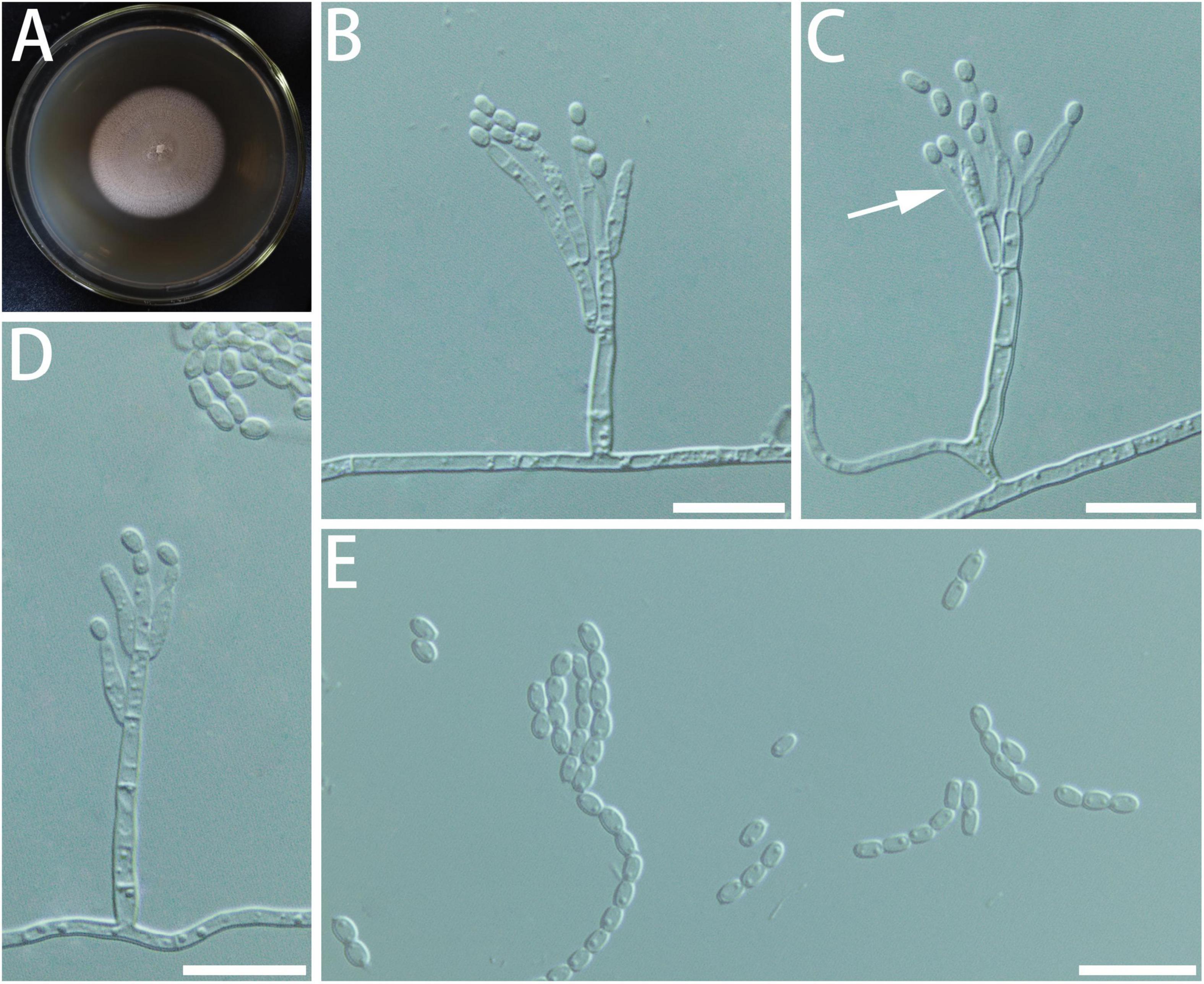
Figure 6. Morphological characteristics of Geosmithia subfulva sp. nov. (SNM1304 = CGMCC3.20579, SNM1298). (A) Eight-day-old culture on 2% malt extract agar. (B–E) Conidiophores and conidia. The metulae (indicated with arrows) branches are few and sparse. Scale bars: 10 μm (B–E).
Type: China, Guangdong Province, Zhuhai City (22°16′48′′ N, 113°30′28′′ E), from the gallery of Ernoporus japonicus in the twig of Hibiscus tiliaceus, 21 June 2021, W. Lin (HMAS 351569 - holotype, SNM1304 = CGMCC3.20579 – ex-holotype culture).
Description: Sexual state not observed. Asexual state penicillium-like and (13.3–) 21.0–43.5 (–62.5)-μm long. Conidiophores arising from substrate or aerial mycelium with all parts verrucose; stipe (5.3–) 9.3–26.4 (–36.6) × (0.9–) 1.5–2.2 (–3.1) μm; penicillus, biverticillate to quaterverticillate, symmetric or asymmetric, often irregularly branched, rarely more, rami (1st branch) in whorls of 1–2, (4.8–) 5.6–7.4 (–8.4) × (1.0–) 1.3–1.8 (–2.0) μm, metulae (last branch) in whorls of 1–3, (4.0–) 4.6–5.9 (–6.9) × (0.9–) 1.2–1.6 (–1.8) μm; phialides 1–3, cylindrical or ellipsoidal, without or with short cylindrical neck and smooth to verrucose walls, (3.6–) 4.8–6.9 (–10.0) × (0.8–) 1.1–1.4 (–1.6) μm. Conidia hyaline, smooth, wide oval shape, (1.1–) 1.5–2.2 (–2.2) × (1.0–) 1.1–1.5 (–1.7) μm. Conidia formed in long, non-persistent conidial chains. Substrate conidia absent.
MEA, 8 days: Colony diameter 17–26 mm at 20°C, 24–36 mm at 25°C, and 20–29 mm at 30°C. At 5 and 35°C, the mycelia grew slowly. After 8 days of culture, the colony diameter was 4–6 and 3–5 mm. The optimal growth temperature is 25°C. Colonies at 25°C, 8 days, plane with radial rows and slightly raised centrally, texture velutinous (powdery); beige to off-white; reverse milky white; soluble pigment and exudate absent. When incubated at 35°C, the colonies are the same as described above. MEA, 37°C, 8 days: no growth.
Host: Hibiscus tiliaceus, Rhus chinensis.
Beetle vectors: Cryphalus kyotoensis, Ernoporus japonicus, Hypothenemus sp. L636.
Distribution: Fujian, Guangdong, Shandong.
Notes: Isolates SNM1304 and SNM1298 formed a monophyletic clade on both ITS, TUB2, TEF1-α, and RPB2 trees (Supplementary Figures S1–S4). On the ITS tree, SNM1304 and SNM1298 were distinct from all other species. On the TUB2 tree, SNM1304 and SNM1298 are linked to G. pulverea but have no strong support. On TEF1-α and RPB2 trees, they are nested in a clade including not only G. pulverea but also several other species.
Additional cultures examined: China, Guangdong Province, Zhuhai City (22°16′48′′ N, 113°30′28′′ E), from the gallery of Ernoporus japonicus LW320 in the twig of Hibiscus tiliaceus, 21 June 2021, W. Lin (SNM1298).
Geosmithia pulverea R. Chang and X. Zhang, sp. nov.
MycoBank MB839259
Etymology: pulverea, powdery in Latin. On MEA medium, G. pulverea has powdery sporulation.
Diagnosis: Geosmithia pulverea produces long spore chains, while its closely related species does not (Figure 7).
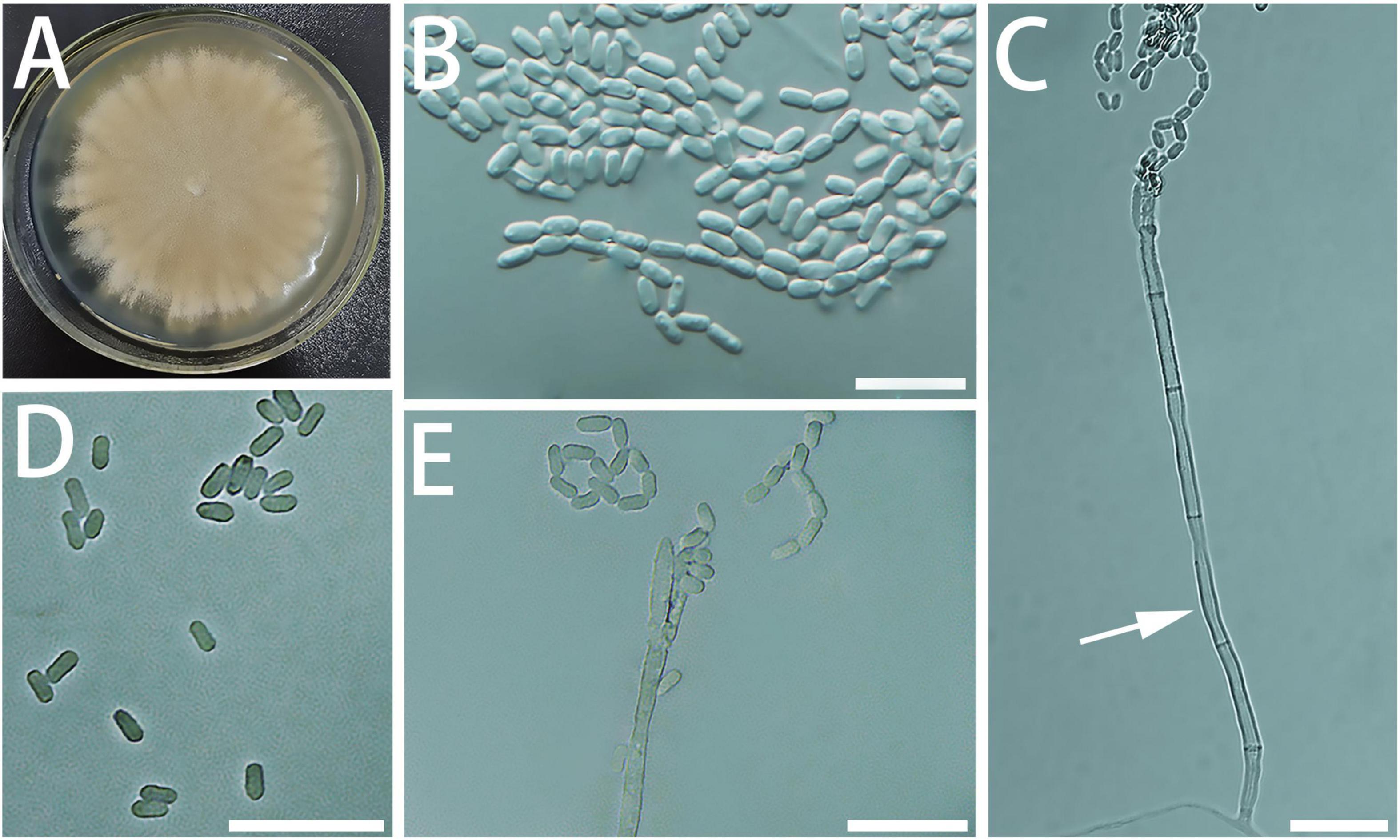
Figure 7. Morphological characteristics of Geosmithia pulverea sp. nov. (SNM885 = CGMCC3.20255, SNM270, SNM888). (A) Eight-day-old culture on 2% malt extract agar. (B–E) Conidiophores and conidia. The stipe (indicated with arrows) are slender and abundant with spores. Scale bars: 10 μm (B–E).
Type: China, Guangdong Province, Shenzhen City (21°55′12″ N, 101°16′12″ E), from the gallery of Dinoderus sp. L489 in the vine of Gnetum luofuense, 12 April 2018, Y. Li (HMAS 249922 – holotype, SNM885 = CGMCC3.20255 – ex-holotype culture).
Description: Sexual state not observed. Asexual state penicillium-like and (17.5-) 30.9–84.3 (-120.1)-μm long. Conidiophores arising from substrate or aerial mycelium with all parts verrucose; base often consisting of curved and atypically branched cell; stipe (16.2–) 32.7–85.7 (–153.9) × (1.9–) 2.5–3.7 (–4.7) μm; penicillus, biverticillate to quaterverticillate, symmetric or asymmetric, often irregularly branched, rarely more, rami (1st branch) in whorls of 2–4, (8.2–) 10.2–14.4 (–18.9) × (2.2–) 2.5–3.3 (–3.9) μm, metulae (last branch) in whorls of 2–3, (6.3–) 7.5–10.9 (–15.8) × (1.8–) 2.1–2.8 (–3.5) μm; phialides 1–3, cylindrical or ellipsoidal, without or with short cylindrical neck and smooth to verrucose walls, (5.3–) 7.0–9.6 (–12.3) × (1.5–) 1.8–2.5 (–3.0) μm. Conidia hyaline, smooth, narrowly cylindrical to ellipsoidal, (2.1–) 2.5–3.4 (–5.1) × (1.1–) 1.2–1.6 (–2.0) μm. Conidia formed in long, non-persistent conidial chains. Substrate conidia absent.
MEA, 8 days: Colony diameter 23–29 mm at 20°C, 30–37 mm at 25°C, and 31–36 mm at 30°C. No growth at 5°C. At 35°C, the mycelia grew slowly. After 8 days of culture, the colony diameter was 1.5–4 mm, with a yellow soluble pigment. The optimal growth temperature is 25–30°C. Colonies at 25°C, 8 days, plane with radial rows and slightly raised centrally, texture velutinous (powdery); sporulation abundant, light brownish yellow to buff; reverse yellowish to slightly avellaneous brown; soluble pigment and exudate absent. When incubated at 35°C, the colonies are the same as described above. MEA, 37°C, 8 days: no growth.
Host: Acacia pennata, Gnetum luofuense, Liquidambar formosana, L. styraciflua, Choerospondias axillaris, Lauraceae, Eriobotrya japonica, Rhus chinensis, Ulmus spp.
Beetle vectors: Sinoxylon cf. cucumella, Acanthotomicus suncei, Crossotarsus emancipatus, Dinoderus sp. L489, Microperus sp. L589, Phloeosinus sp., Scolytus semenovi, Scolytus jiulianshanensis, Cryphalus kyotoensis, Cryphalus eriobotryae.
Distribution: Fujian, Guangdong, Guangxi, Hunan, Jiangxi, Yunnan, Shandong, Shanghai.
Notes: Geosmithia pulverea colony was powdery and brown-yellow. One of the most obvious features is the long spore chain. According to the tree made by ITS sequence, SNM888, SNM885, and SNM248 were clustered with Geosmithia sp. 3, and SNM886, SNM887, and SNM270 were clustered with Geosmithia sp. 23 (Supplementary Figure S1). However, in the trees with TUB2, TEF1-α, and RPB2, these strains did not have a clear subclassification (Supplementary Figures S2–S4). It was consequently recognized, using multigene phylogeny, together with Geosmithia sp. 23, as a well-defined phylogenetic species inside the G. pallida species complex (Huang et al., 2017; Kolařík et al., 2017). The colony of G. pulverea was very similar to Geosmithia sp. 3 on MEA, but Geosmithia sp. 3 was darker and wrinkled (Kolařík et al., 2004). Geosmithia pulverea seems to have a smaller stipe size, but other features fit the morphology of Geosmithia sp. 3 (Kolařík et al., 2004). In this study, we are providing a formal description for the Chinese strains related to Geosmithia sp. 3 and sp. 23 which are known to be distributed over various bark beetle hosts in Temperate Europe in the case of Geosmithia sp. 3 (Kolařík et al., 2004, 2008; Strzałka et al., 2021) or seems to have a global distribution and many bark beetle hosts across Temperate Europe (Strzałka et al., 2021), the Mediterranean Basin (Kolařík et al., 2007), Northern America (Huang et al., 2017, 2019; Kolařík et al., 2017), and Seychelles (Kolařík et al., 2017). Further study is needed to assess the taxonomic relationships between G. pulverea, Geosmithia sp. 3, and Geosmithia sp. 23.
Additional cultures examined: China, Guangxi Province, Shangsi City, Shiwandashan Mt. (21°54′12′′ N, 107°54′14′′ E), from the body surface of Crossotarsus emancipates, 27 March 2018, Y. Li (SNM887).
China, Hunan Province, Changsha City, Yuelushan Mt. (28°10′56′′ N, 112°55′41′′ E), from the gallery of Microperus sp. L589 on the trunk of Choerospondias axillaris, 15 July 2019, Y. Li (SNM888).
Geosmithia fusca R. Chang and X. Zhang, sp. nov.
MycoBank MB841506
Etymology: fusca, referring to the brown appearance of the colony on MEA.
Diagnosis: The difference with closely related species G. cucumellae is reflected in such a way that the conidia of G. fusca are smooth and do not produce long spore chains (Figure 8).
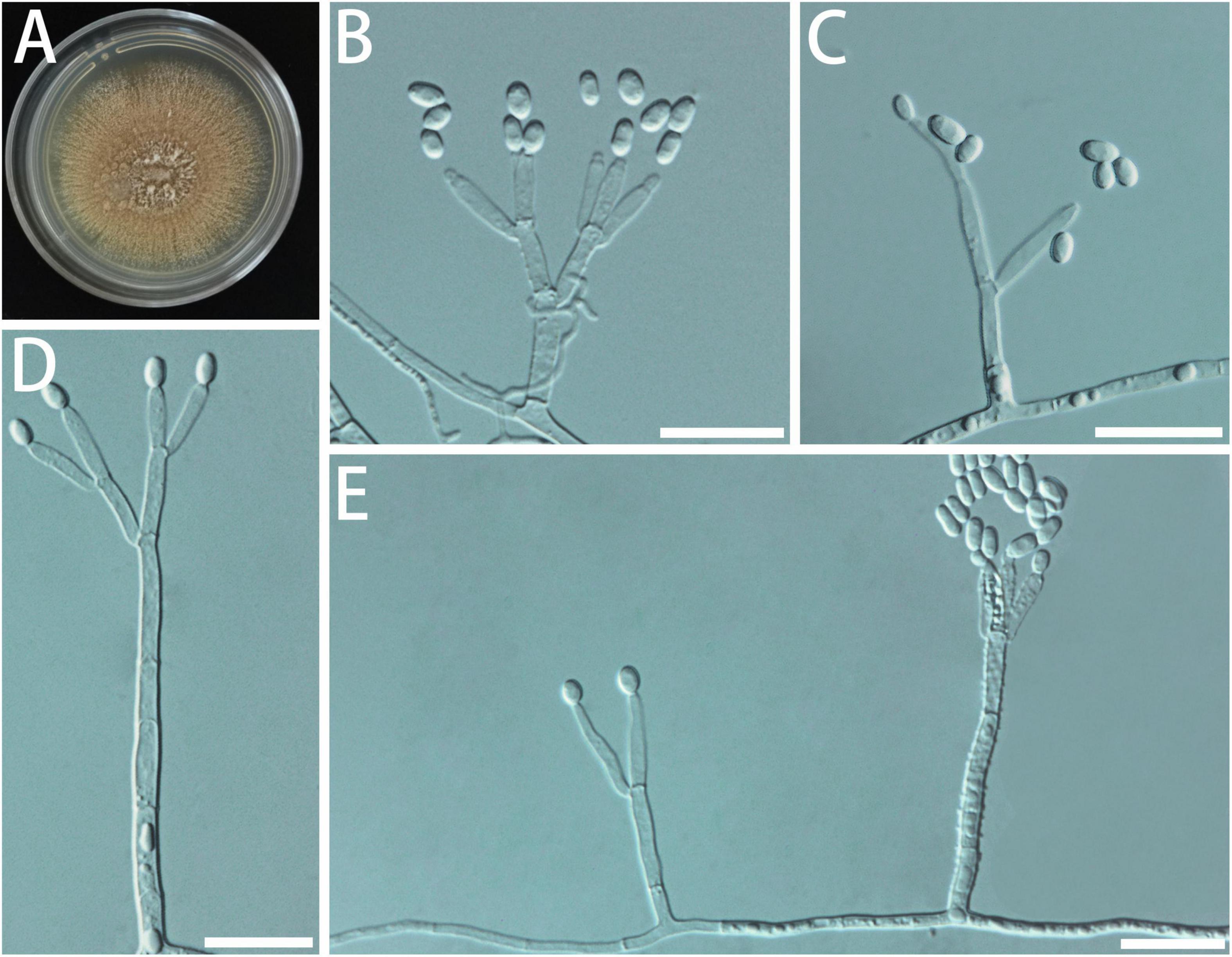
Figure 8. Morphological characteristics of Geosmithia fusca sp. nov. (SNM1578 = CGMCC3.20626, SNM1577). (A) Eight-day-old culture on 2% malt extract agar. (B–E) Conidiophores and conidia. Conidia hyaline, smooth, wide oval shape. Scale bars: 10 μm (B–E).
Type: China, Guangdong Province, Zhuhai City, Agricultural Science Research Center (22°18′9′′ N, 113°31′40′′ E), from the gallery of Xylocis tortilicornis on Phyllanthus emblica, 6 July 2021, W. Lin (HMAS 351570 - holotype, SNM1578 = CGMCC3.20626 – ex-holotype culture).
Description: Sexual state not observed. Asexual state penicillium-like and (16.3–) 20.2–55.8 (–94.3)-μm long. Conidiophores variable in shape and size, emerging from a surface mycelium, with all segments smooth or minutely verrucose to distinctly verrucose, septate, stipe (8.6–) 10.1–38.5 (–70.1) × (1.2–) 1.5–2.1 (–2.6) μm; penicilli typically shorter than the stipe, terminal, monoverticillate or biverticillate, symmetric or asymmetric, irregularly branched; metulae in whorls of 2–3, (4.9–) 6.0–8.3 (–9.9) × (1.1–) 1.3–1.8 (–2.1) μm; phialides in whorls of 1–3, smooth, (4.6–) 5.8–8.1 (–9.4) × (1.0–) 1.3–1.7 (–1.9) μm. Conidia cylindrical to ellipsoid, (1.5–) 2.0–2.7 (–3.4) × (0.9–) 1.1–1.7 (–1.7) μm. Conidia formed in long, non-persistent conidial chains. Substrate conidia absent.
MEA, 8 days: Colony diameter 21–26 mm at 20°C, 25–36 mm at 25°C, and 26–32 mm at 30°C. At 5 and 35°C, the mycelia grew slowly. After 8 days of culture, the colony diameter was 1–3 and 7–11 mm, respectively. The optimal growth temperature is 25–30°C. At 25°C, 8 days: Colonies flat with radial rows, surface texture powdery; sporulation abundant, brown; central hyphae were raised and white flocculent; reverse yellowish to brown; without exudate and insoluble pigment. MEA, 37°C, 8 days: germinating only.
Host: Hibiscus tiliaceus, Phyllanthus emblica, Acacia pennata.
Beetle vectors: Ernoporus japonicus, Xylocis tortilicornis, Sinoxylon cf. cucumella.
Distribution: Guangdong, Yannan.
Notes: In the phylogenetic tree, SNM1012, SNM1067 and SNM1577, SNM1578 formed very close separate branches (Figure 1 and Supplementary Figures S1–S4), but combined with morphological analysis, it was found that SNM1012, SNM1067 and SNM1577, SNM1578 had no significant difference except for a small difference in spore length (Supplementary Figure S5). So, they are described as the same species.
Additional cultures examined: China, Guangdong Province, Zhuhai City, Agricultural Science Research Center (22.3025 N, 113.5277 E), from the gallery of Xylocis tortilicornis LW319 on Phyllanthus emblica, 6 July 2021, W. Lin (SNM1577).
China, Yunnan Province, Xishuangbanna City, Xishuangbanna Botanical Garden (21°55′1′′ N, 101°16′1′′ E), from the gallery of Sinoxylon cf. cucumella on the trunk of Acacia pennata, 1 May, 2021, Y. Dong and Y. Li (SNM1012, SNM1167).
Geosmithia pumila R. Chang and X. Zhang, sp. nov.
MycoBank MB841507
Etymology: pumila, referring to the tree host of Ulmus pumila where this species has been isolated.
Diagnosis: Isolates of G. pumila formed a monophyletic clade on all the phylogenetic trees (Figure 9).
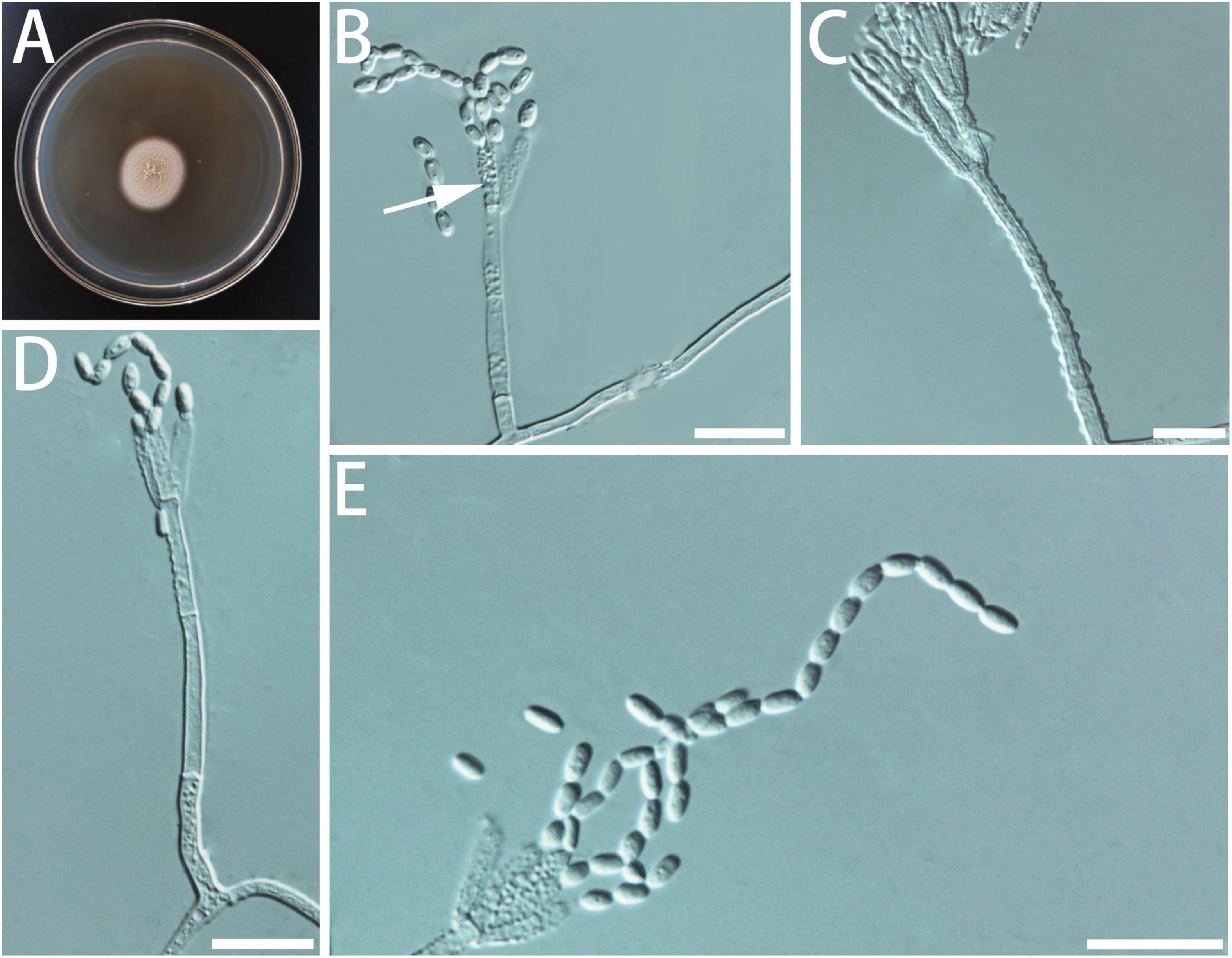
Figure 9. Morphological characteristics of Geosmithia pumila sp. nov. (SNM1653 = CGMCC3.20630, SNM1657). (A) Eight-day-old culture on 2% malt extract agar. (B–E) Conidiophores and conidia. Most phialides (indicated with arrows) are not smooth. Scale bars: 10 μm (B–E).
Type: China, Jiangsu Province, Nanjing City, Nanjing Forestry University (32°3′36′′ N, 118°48′36′′ E), from the gallery of Scolytus semenovi in the branch of Ulmus sp., 25 August 2021, S. Lai (HMAS 351571 - holotype, SNM1653 = CGMCC3.20630 – ex-holotype culture).
Description: Sexual state not observed. Asexual state penicillium-like and (12.9–) 35.9–72.7 (–109.4)-μm long. Conidiophores arising from substrate or aerial mycelium with all parts verrucose; stipe (9.9–) 19.7–51.5 (–77.9) × (1.2–) 1.4–2.2 (-2.6) μm; penicillus, monoverticillate or biverticillate, mostly monoverticillate, symmetric or asymmetric, often irregularly branched, rarely more, metulae in whorls of 2 to 3, (5.1–) 6.3–8.9 (–10.5) × (1.1–) 1.4–2.0 (–2.3) μm; phialides 1–3, smooth to verrucose walls, (5.0–) 5.7–7.3 (–8.5) × (1.1–) 1.2–1.6 (–1.8) μm. Conidia hyaline, smooth, ellipsoidal, (1.5–) 1.9–2.5 (–2.9) × (0.9–) 1.1–1.5 (–1.9) μm. Conidia formed in long, non-persistent conidial chains. Substrate conidia absent.
MEA, 8 days: Colony diameter 25–29 mm at 20°C, 25–33 mm at 25°C, and 22–26 mm at 30°C. At 35°C: germinating only. At 5°C, the mycelia grew slowly. After 8 days of culture, the colony diameter was 7–10 mm. The optimal growth temperature is 20–25°C. Colonies at 25°C, 8 days, plane with radial rows, texture velutinous (powdery), slightly funiculus centrally; sporulation medium, light yellow to rice white; reverse milk-white; soluble pigment and exudate absent. MEA, 37°C, 8 days: no growth.
Host: Ulmus sp.
Beetle vectors: Scolytus semenovi.
Distribution: Jiangsu.
Notes: Based on ITS, TUB2, TEF1-α, and RPB2 trees (Supplementary Figures S1–S4), SNM1653 and SNM1657 were grouped with Geosmithia sp. 2. Therefore, we considered that SNM1653, SNM1657, and Geosmithia sp. 2 were the same species. This extends the geographical range to Europe, the Mediterranean Basin (Kolařík et al., 2007, 2008), the whole United States (Huang et al., 2017, 2019; Kolařík et al., 2017), Peru (Kolařík et al., 2004), and South Africa (Machingambi et al., 2014), which is reported to be in association with a large number of insect and tree hosts.
Additional cultures examined: China, Jiangsu Province, Nanjing City, Nanjing Forestry University (32°3′36′′ N, 118°48′36′′ E), from the gallery of Scolytus semenovi in the branch of Ulmus sp., 25 August 2021, S. Lai (SNM1657).
Geosmithia bombycina R. Chang and X. Zhang, sp. nov.
MycoBank MB 840535
Etymology: bombycina, referring to the cotton appearance of the colony on MEA.
Diagnosis: Isolates of G. bombycina formed a monophyletic clade on all the phylogenetic trees (Figure 10).
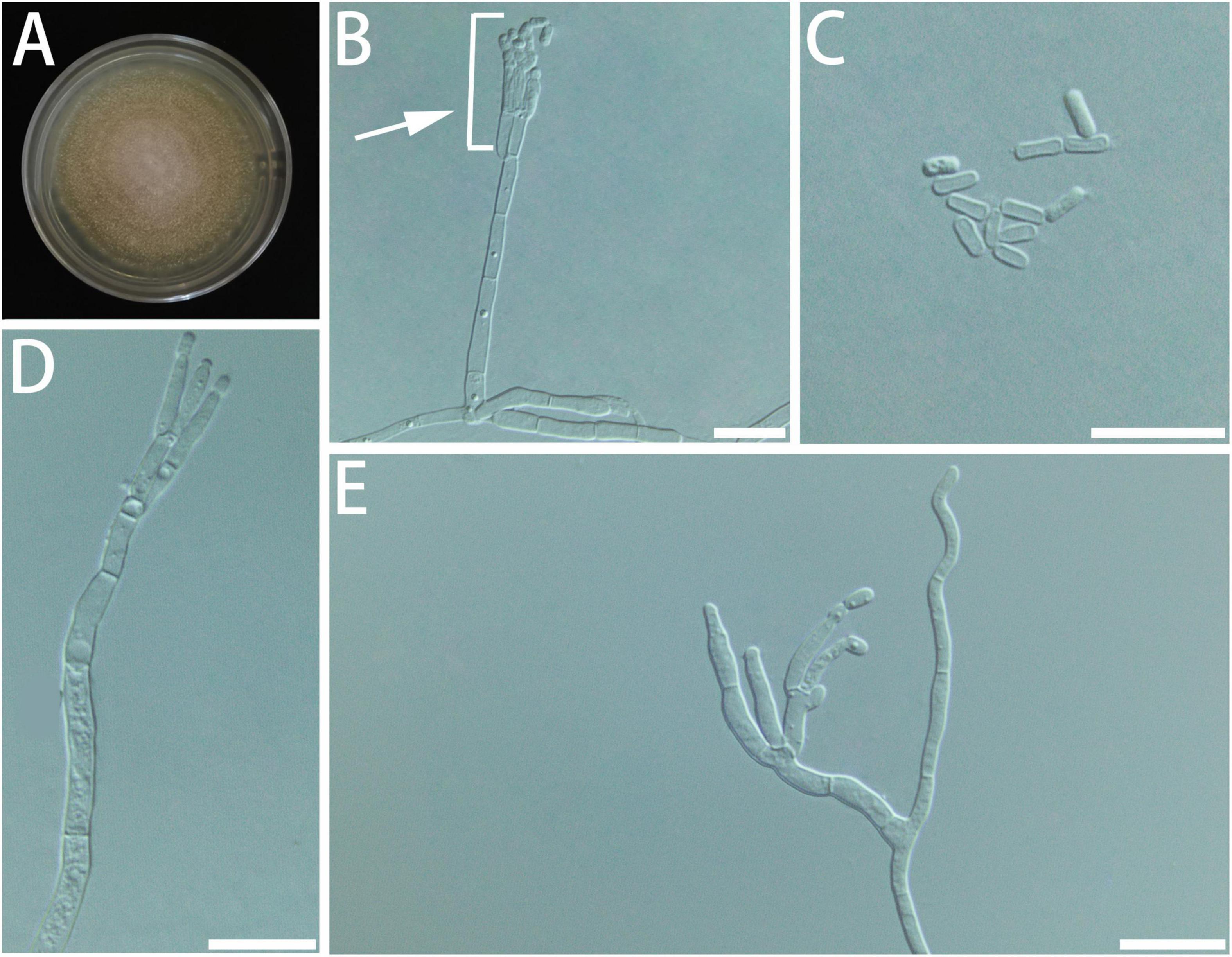
Figure 10. Morphological characteristics of Geosmithia bombycina sp. nov. (SNM933 = CGMCC3.20578, SNM934). (A) Eight-day-old culture on 2% malt extract agar. (B–E) Conidiophores and conidia. The penicilli (indicated with arrows) are typically shorter than the stipe, terminal, monoverticillate, biverticillate or terverticillate, symmetric or asymmetric. Scale bars: 10 μm (B–E).
Type: China, Fujian Province, Fuqing City (25°71′ N, 119°15′ E), from the gallery of Cryphalus eriobotryae on Eriobotrya japonica, 8 April 2021, Y. Li (HMAS 350284 – holotype, SNM933 = CGMCC3.20578 – ex-holotype culture).
Description: Sexual state not observed. Asexual state penicillium-like, (14.0–) 20.2–41.0 (–62.6) μm in length. Conidiophores emerging from hyphae, smooth, septate; stipe (5.4–) 9.4–30.0 (–47.5) × (0.9–) 1.4–2.0 (–2.4) μm; penicilli typically shorter than the stipe, terminal, monoverticillate, biverticillate or terverticillate, symmetric or asymmetric, often irregularly branched, rarely more; metulae in whorls of 1–2, (5.1–) 5.9–8.3 (–10.5) × (1.0–) 1.2–1.7 (–2.1) μm; phialides in whorls of 2–4, smooth, (4.9–) 5.8–9.4 (–12.6) × (0.9–) 1.3–1.7 (–2.0) μm. Conidia hyaline, smooth, narrow, and oval, (2.1–) 2.4–3.3 (–4.1) × (0.8–) 0.9–1.3 (–1.5) μm, produced in non-persistent chains. Substrate conidia absent.
MEA, 8 days: Colony diameter 20–23 mm at 20°C, 24–31 mm at 25°C, and 22–30 mm at 30°C. The hyphae grow slowly at 5 and 35°C. After 8 days of culture, the colony diameter was less than 1 and 5–8 mm, respectively. The optimal temperature for growth was 25–30°C. At 25°C, 8 days: The colonies were flat, like annual rings; central hyphae were raised and white flocculent; filamentous, diffuse, basal mycelium sparse; conidiogenesis moderate, milk-white; reverse creamy white; no exudate and insoluble pigment. When incubated at 35°C, the colonies grew, and the mycelia were sparse and snowflake-shaped, with no soluble pigment. MEA, 37°C, 8 days, no growth.
Host: Eriobotrya japonica.
Beetle vectors: Cryphalus eriobotryae.
Distribution: Currently only known from Fujian.
Notes: According to ITS, TUB2, and TEF1-α trees (Supplementary Figures S1–S4), SNM933 and SNM934 formed a monophyletic clade and nested with Geosmithia sp. 22, Geosmithia sp. 24, G. longistipitata, G. pazoutovae, and G. fagi. The RPB2 sequences for those species were not available on GenBank. Therefore, SNM933 and SNM934 formed a distinct clade that was far away from all the known species on the RPB2 tree.
Additional cultures examined: China, Fujian Province, Fuqing City (25°71′ N, 119°15′ E), from the gallery of Cryphalus eriobotryae on Eriobotrya japonica, 8 April 2021, Y. Li (SNM934).
Discussion
This is the first relatively comprehensive study of Geosmithia species associated with bark beetle in China. The samples were collected from 9 provinces, 12 tree hosts, and 12 bark and ambrosia beetles. A total of 178 strains of Geosmithia were isolated in this study. The analyses of ITS, RBP2, TUB2, and TEF1-α showed that those isolates were separated into 12 taxa, with three strains previously described, G. xerotolerans, G. putterillii, and G. pallida, and the other nine were novel species, described as G. luteobrunnea, G. radiata, G. brevistipitata, G. bombycina, G. granulata (Geosmithia sp. 20), G. subfulva, G. pulverea (Geosmithia sp. 3 and Geosmithia sp. 23), G. fusca, and G. pumila in this study. Those species were isolated from larvae, frass, and wood dust in the beetle galleries of dying, stressed, or weakened broad-leaf and conifer tree hosts, such as Liquidambar spp., Ulmus sp., and Cupressus sp.
The dominant species obtained in this study were G. luteobrunnea and G. pulverea, with 39 and 33 strains, respectively (Table 1). The reason for their abundance in our dataset is the fact that our study focused on sampling from Altinginaceae. Two species, G. putterillii and G. radiata, have only been isolated in Jiangxi (Table 1). The samples collected from Guangxi and Hunan only yielded G. pulverea.
Geosmithia putterillii was isolated from bark beetles feeding on plants from the family of Rosaceae (Kolařík et al., 2008) and Lauraceae in Europe (Kolařík et al., 2004) and on various families of angiosperms and gymnosperms in the Western United States (Kolařík et al., 2017). The type strain was isolated from timber in New Zealand (Pitt, 1979). In this study, G. putterillii was isolated from the gallery of Phloeosinus sp. on Lauraceae log (Jiangxi). This study is the first report of G. putterillii in China. It is becoming clear that G. putterillii is widely distributed globally, across many beetle hosts.
Another known species collected in this study is G. pallida, originally isolated from cotton yarn and soil (Kolařík et al., 2004). Later, it was found to be associated with beetles, such as ambrosia beetle Xylosandrus compactus (Vannini et al., 2017), and plants such as Brucea mollis (Deka and Jha, 2018). G. pallida was previously reported to induce dieback poisoning on coast live oak (Quercus agrifolia) by Lynch et al. (2014). Later, it was proved that the identification was incorrect, and the causal agent of this disease was confirmed to be Geosmithia sp. 41 (Kolařík et al., 2017). Two isolates were obtained from the gallery of Sinoxylon cf. cucumella on Acacia pennata in this study, which is the first report of G. pallida in China.
Most of G. luteobrunnea were isolated from the galleries of A. suncei (Table 1). Acanthotomicus suncei was recorded on Liquidambar in Fujian, Jiangsu, Jiangxi, Zhejiang, and Shanghai, China (Li et al., 2021). The hosts of this beetle were limited to sweet gum trees, such as L. styraciflua and L. formosana. The beetle was recorded as an agent of great damage to the imported American sweetgum L. styraciflua in Shanghai and neighboring Jiangsu Province (Gao and Cognato, 2018). The role of the fungus in this outbreak and the tree pathology remain uninvestigated, although the authors of this paper noted small lesions around the beetle galleries. The other five isolates were isolated from the galleries of S. jiulianshanensis on Ulmus sp., which suggests that G. luteobrunnea might colonize a wide range of tree hosts.
Geosmithia radiata was only isolated in samples from Jiangxi Province, from two plant families: Altinginaceae and Ulmaceae (Table 1). The colony of G. radiata is similar to G. luteobrunnea in morphology, but the difference can be seen in the micromorphology (Supplementary Figure S5). In addition, G. luteobrunnea can grow faster at 35°C, while G. radiata grows slower, and G. luteobrunnea could grow at 35°C, but G. radiata could not (Table 4).
Geosmithia brevistipitata and G. xelotolerans were isolated from the gallery of Phloeosinus cf. hopehi. This is not the first time that Geosmithia species were isolated from the gallery of Phloeosinus species. According to previous reports, G. flava, G. longdonii, G. putterillii, G. Lavandula, etc., are all related to Phloeosinus (Kolařík et al., 2017). It is now more certain that Phloeosinus and Geosmithia are closely related. Geosmithia xelotolerans is cosmopolite, known from the Mediterranean on many bark beetle species infecting Fabaceae, Moraceae, Oleaceae (Kolařík et al., 2007), in Western US on Cupressaceae, Pinaceae Fagaceae, Rosaceae (Kolařík et al., 2017), and in Eastern US on Cupressaceae, Fagaceae (Huang et al., 2017, 2019), and wall of the wall (Spain, Crous et al., 2018). Our study expanded the distribution range of G. xelotolerans.
Geosmithia bombycina was isolated from the gallery of C. eriobotryae on E. japonica. Cryphalus eriobotryae is one of the beetle pests that infest loquat (Zheng et al., 2019). This is the first study about the fungal association of this beetle.
Geosmithia granulata was isolated from the gallery of Sinoxylon cf. cucumella on Acacia pennata, Ernoporus japonicus on Hibiscus tiliaceus, and Scolytus semenovi on Ulmus sp. in this study. It was reported that it could be vectored by different beetle species which infested several plant hosts (Kolařík et al., 2007). In this study, we expanded the range of its beetle vectors and tree host.
Geosmithia pulverea is a species closely related to Geosmithia sp. 3 and Geosmithia sp. 23, which are known from various bark beetle hosts in Europe, United States, and Seychelles (Kolařík et al., 2007, 2008, 2017; Huang et al., 2017, 2019). Further study is needed to clarify the evolutionary relationship among these three lineages. In this study, we isolated G. pulverea from Aca. gracilipes, Alt. gracilipes, E. japonica, Gne. luofuense, L. formosana, L. styraciflua, Rhus chinensis, and Ulmus sp. (Table 1), which suggested that this species could colonize a very wide variety of plant hosts. It is also the most widely distributed species, isolated from Guangdong, Guangxi, Hunan, Jiangsu, Jiangxi, Shandong, and Shanghai (Table 1) and vectored by several beetle species, such as S. jiulianshanensis, A. suncei, C. emancipatus, C. kyotoensis, Dinoderus sp., Microperus sp., and Phloeosinus sp. (Table 1). Moreover, the abundance of Geosmithia species associated with A. suncei in the current study was also consistent with the frequent occurrence in Shanghai and Jiangxi (Gao et al., 2021).
In addition to G. pallida, Geosmithia pulverea, and Geosmithia fusca are the species found in the G. pallida species complex in this study. Only eight isolates of G. fusca were obtained from the gallery of Sinoxylon cf. cucumella on Acacia pennata. Two isolates of G. pallida, eight isolates of G. pulverea, and two isolates of G. granulata were also obtained from this beetle. Information about this beetle was very limited. As far as we know, it was found on Wendlandia tinctoria and distributed in the Himalayan mountain area and Burma, Thailand, Laos, and Vietnam (Liu, 2010; Liu and Beaver, 2018; Borowski, 2021). This is the first report in China, and this is the first study on its fungal associations.
Conclusion
This study does not provide sufficient data to determine the structure of the Geosmithia community in China, as was inferred in Europe and United States after a significantly greater sampling effort (Kolařík et al., 2007, 2008, 2017; Kolařík and Jankowiak, 2013; Jankowiak et al., 2014; Huang et al., 2017, 2019). Fungal communities are regulated by several factors, including geographic location, host tree species, and bark beetle vectors. Further sampling is needed to understand the determinants (Veselská et al., 2019). It is clear, however, that the diversity of China’s subcortical fungi is substantial. Fungal communities associated with trees need to be further investigated because many currently unknown species may cause plant diseases.
Data Availability Statement
The datasets presented in this study can be found in online repositories. The names of the repository/repositories and accession number(s) can be found in the article/Supplementary Material.
Author Contributions
RC, MD, and YL designed the research. YL, HS, and GZ collected the samples. XZ, RC, and YL isolated and purified the fungal cultures. XZ, RC, and XJ completed the data acquisition, analyses, and interpretation. XZ and RC completed the writing of the manuscript. MK, JH, and YL revised the text, taxonomy, and phylogeny. All authors approved the manuscript.
Funding
JH was partially funded by a cooperative agreement with the USDA Forest Service, by the USDA APHIS Plant Protection Act, and by the National Science Foundation.
Author Disclaimer
This publication may not necessarily express the views of APHIS.
Conflict of Interest
The authors declare that the research was conducted in the absence of any commercial or financial relationships that could be construed as a potential conflict of interest.
Publisher’s Note
All claims expressed in this article are solely those of the authors and do not necessarily represent those of their affiliated organizations, or those of the publisher, the editors and the reviewers. Any product that may be evaluated in this article, or claim that may be made by its manufacturer, is not guaranteed or endorsed by the publisher.
Acknowledgments
We would like to thank Ling Zhang, Yufeng Cao, Shengchang Lai (Jiangxi Agricultural University), Dr. Yongying Ruan (Shenzhen Polytechnic), Yiyi Dong (University of Florida), and Dr. Lei Gao (Shanghai Academy of Landscape Architecture Science and Planning) for assisting in insect collection, Dr. Sarah Smith and Prof. Anthony Cognato (Michigan State University) and Mr. Wei Lin (Technical Center of Gongbei Customs District People’s Republic of China) for assisting with beetle identification, and Dr. Shuping Wang (Shanghai Entry-Exit Inspection and Quarantine Bureau) and Jue Wang (Beijing Forest University) for assisting with sequencing.
Supplementary Material
The Supplementary Material for this article can be found online at: https://www.frontiersin.org/articles/10.3389/fmicb.2022.820402/full#supplementary-material
Abbreviations
BI, Bayesian inference; ITS, nuclear ribosomal internal transcribed spacer; TEF1- α, translation elongation factor 1- α; TUB2, β-tubulin; ML, maximum likelihood; PCR, polymerase chain reaction; CGMCC, China General Microbiological Culture Collection Center; HMAS, Herbarium Mycologicum, Academiae Sinicae; TCD, thousand cankers disease.
Footnotes
- ^ http://tree.bio.ed.ac.uk/software/tracer
- ^ https://www.treebase.org/
- ^ https://imagej.net/
- ^ https://www.ibm.com/analytics/spss-statistics-software
References
Borowski, T. (2021). World inventory of beetles of the family Bostrichidae (Coleoptera). Part 2. Check list from 1758 to 2007. World News Nat. Sci. 36, 9–41.
Crous, P. W., Luangsa-Ard, J. J., Wingfield, M. J., Carnegie, A. J., Hernández-Restrepo, M., Lombard, L., et al. (2018). Fungal Planet description sheets: 785-867. Persoonia 41, 238–417. doi: 10.3767/persoonia.2018.41.12
Darriba, D., Taboada, G. L., Doallo, R., and Posada, D. (2012). jModelTest 2: more models, new heuristics and parallel computing. Nat. Methods 9:772. doi: 10.1038/nmeth.2109
Deka, D., and Jha, D. K. (2018). Optimization of culture parameters for improved production of bioactive metabolite by endophytic Geosmithia pallida (KU693285) isolated from Brucea mollis Wall ex. Kurz, an endangered medicinal plant. J. Pure Appl. Microbiol. 12, 1205–1213. doi: 10.22207/jpam.12.3.21
Dori-Bachash, M., Avrahami-Moyal, L., Protasov, A., Mendel, Z., and Freeman, S. (2015). The occurrence and pathogenicity of Geosmithia spp. and common blue-stain fungi associated with pine bark beetles in planted forests in Israel. Eur. J. Plant Pathol. 143, 627–639.
Gao, L., and Cognato, A. I. (2018). Acanthotomicus suncei, a new sweetgum tree pest in China (Coleoptera: Curculionidae: Scolytinae: Ipini). Zootaxa 447, 595–599. doi: 10.11646/zootaxa.4471.3.12
Gao, L., Li, Y., Wang, Z.-X., Zhao, J., Hulcr, J., Wang, J.-G., et al. (2021). Biology and associated fungi of an emerging bark beetle pest, the sweetgum inscriber Acanthotomicus suncei (Coleoptera: Curculionidae). J. Pure Appl. Microbiol. 145, 508–517. doi: 10.1111/jen.12861
Gardes, M., and Bruns, T. D. (1993). ITS primers with enhanced specificity for basidiomycetes – application to the identification of mycorrhizae and rusts. Mol. Ecol. 2, 113–118. doi: 10.1111/j.1365-294X.1993.tb00005.x
Glass, N. L., and Donaldson, G. C. (1995). Development of primer sets designed for use with the PCR to amplify conserved genes from filamentous ascomycetes. Appl. Environ. Microb. 61, 1323–1330. doi: 10.1128/aem.61.4.1323-1330.1995
Grum-Grzhimaylo, A. A., Georgieva, M. L., Debets, A. J., and Bilanenko, E. N. (2013). Are alkalitolerant fungi of the Emericellopsis lineage (Bionectriaceae) of marine origin? IMA fungus 4, 213–228. doi: 10.5598/imafungus.2013.04.02.07
Hamelin, R., Tanguay, P., Uzunovic, A., and Seifert, K. (2013). Molecular Detection Assays of Forest Pathogens. Sault Ste. Marie, ON: Canadian Forest Service, Natural Resources Canada.
Hishinuma, S. M., Dallara, P. L., Yaghmour, M. A., Zerillo, M. M., Parker, C. M., Roubtsova, T. V., et al. (2015). Wingnut (Juglandaceae) as a new generic host for Pityophthorus juglandis (Coleoptera: Curculionidae) and the thousand cankers disease pathogen, Geosmithia morbida (Ascomycota: Hypocreales). Can. Entomol. 148, 83–91. doi: 10.4039/tce.2015.37
Huang, Y. T., Kolařík, M., Kasson, M. T., and Hulcr, J. (2017). Two new Geosmithia species in G. pallida species complex from bark beetles in eastern USA. Mycologia 109, 790–803. doi: 10.1080/00275514.2017.1410422
Huang, Y.-T., Skelton, J., Johnson, A. J., Kolařík, M., and Hulcr, J. (2019). Geosmithia species in southeastern USA and their affinity to beetle vectors and tree hosts. Fungal Ecol. 39, 168–183. doi: 10.1016/j.funeco.2019.02.005
Jankowiak, R., Kolařík, M., and Bilański, P. (2014). Association of Geosmithia fungi (Ascomycota: Hypocreales) with pine- and spruce-infesting bark beetles in Poland. Fungal Ecol. 11, 71–79. doi: 10.1016/j.funeco.2014.04.002
Juzwik, J., Banik, M. T., Reed, S. E., English, J. T., and Ginzel, M. D. (2015). Geosmithia morbida Found on Weevil Species Stenomimus pallidus in Indiana. Plant Health Prog. 16, 7–10. doi: 10.1094/PHP-RS-14-0030
Katoh, K., and Standley, D. M. (2013). MAFFT multiple sequence alignment software version 7: improvements in performance and usability. Mol. Biol. Evol. 30, 772–780. doi: 10.1093/molbev/mst010
Kirschner, R. (2001). “Diversity of filamentous fungi in bark beetle galleries in central Europe,” in Trichomycetes and Other Fungal Groups, Professor Robert W. Lichtwardt Commemoration Volume, eds J. K. Misra and B. W. Horn (Plymouth: Enfield, B.W. Science Publishers, Inc), 175–196. doi: 10.1007/s10482-020-01510-6
Kolařík, M., Freeland, E., Utley, C., and Tisserat, N. (2011). Geosmithia morbida sp. nov., a new phytopathogenic species living in symbiosis with the walnut twig beetle (Pityophthorus juglandis) on juglans in USA. Mycologia 103, 325–332. doi: 10.3852/10-124
Kolařík, M., Hulcr, J., Tisserat, N., De Beer, W., Kostovčík, M., Kolaříková, Z., et al. (2017). Geosmithia associated with bark beetles and woodborers in the western USA: taxonomic diversity and vector specificity. Mycologia 109, 185–199. doi: 10.1080/00275514.2017.1303861
Kolařík, M., and Jankowiak, R. (2013). Vector Affinity and Diversity of geosmithia fungi living on subcortical insects inhabiting pinaceae species in central and Northeastern Europe. Microb. Ecol. 66, 682–700. doi: 10.1007/s00248-013-0228-x
Kolařík, M., and Kirkendall, L. R. (2010). Evidence for a new lineage of primary ambrosia fungi in Geosmithia Pitt (Ascomycota: Hypocreales). Fungal Biol. 114, 676–689. doi: 10.1016/j.funbio.2010.06.005
Kolařík, M., Kostovčík, M., and Pažoutová, S. (2007). Host range and diversity of the genus Geosmithia (Ascomycota: Hypocreales) living in association with bark beetles in the Mediterranean area. Mycol. Res. 111, 1298–1310. doi: 10.1016/j.mycres.2007.06.010
Kolařík, M., Kubátová, A., Hulcr, J., and Pažoutová, S. (2008). Geosmithia fungi are highly diverse and consistent bark beetle associates: evidence from their community structure in temperate europe. Mycol. Res. 55, 65–80. doi: 10.1007/s00248-007-9251-0
Kolařík, M., Kubátová, A., PažoutovÁ, S., and Šrůtka, P. (2004). Morphological and molecular characterisation of Geosmithia putterillii, G. pallida comb. nov. and G. flava sp. nov., associated with subcorticolous insects. Mycol. Res. 108, 1053–1069. doi: 10.1017/S0953756204000796
Kolarik, M., Kubatova, A., van Cepicka, I., Pazoutova, S., and Srutka, P. (2005). A complex of three new white-spored, sympatric, and host range limited Geosmithia species. Mycol. Res. 109, 1323–1336. doi: 10.1017/S0953756205003965
Kubátová, A., Kolarik, M., Prasˇil, K., and Novotny, D. (2004). Bark beetles and their galleries: well-known niches for little known fungi on the example of Geosmithia. Czech Mycol. 56, 1–18. doi: 10.33585/cmy.56101
Li, Y., Wan, Y., Lin, W., Ernstsons, A. S., and Gao, L. (2021). Estimating potential distribution of sweetgum pest Acanthotomicus suncei and potential economic losses in nursery stock and Urban Areas in China. Insects 12:155. doi: 10.3390/insects12020155
Lin, Y.-T., Shih, H.-H., Huang, Y.-T., Lin, C.-S., and Chen, C.-Y. (2016). Two species of beetle-associated Geosmithia in Taiwan. Fungal Sci. 31, 29–36. doi: 10.33585/cmy.67103
Liu, L. (2010). New records of Bostrichidae (Insecta: Coleoptera, Bostrichidae, Bostrichinae, Lyctinae, Polycaoninae, Dinoderinae, Apatinae). Mitt. Munch. Entomol. Ges. 100, 103–117.
Liu, L., and Beaver, R. A. (2018). “A synopsis of the powderpost beetles of the Himalayas with a key to the genera (Insecta: Coleoptera: Bostrichidae)” in Biodiversität und Naturausstattung im Himalaya VI, eds M. Hartmann, M. Barclay, and J. Weipert (Germany: Naturkundemuseum Erfurt), 407–422.
Liu, Y. J., Whelen, S., and Hall, B. D. (1999). Phylogenetic relationships among ascomycetes: evidence from an RNA polymerse II subunit. Mol. Biol. Evol. 16, 1799–1808. doi: 10.1093/oxfordjournals.molbev.a026092
Lynch, S. C., Wang, D. H., Mayorquin, J. S., Rugman-Jones, P. F., Stouthamer, R., and Eskalen, A. (2014). First report of Geosmithia pallida causing foamy bark canker, a new disease on coast live oak (Quercus agrifolia), in association with Pseudopityophthorus pubipennis in California. Plant Dis. 98:1276. doi: 10.1094/PDIS-03-14-0273-PDN
Machingambi, N. M., Roux, J., Dreyer, L. L., and Roets, F. (2014). Bark and ambrosia beetles (Curculionidae: Scolytinae), their phoretic mites (Acari) and associated Geosmithia species (Ascomycota: Hypocreales) from Virgilia trees in South Africa. Fungal Biol. 118, 472–483. doi: 10.1016/j.funbio.2014.03.006
McPherson, B. A., Erbilgin, N., Bonello, P., and Wood, D. L. (2013). Fungal species assemblages associated with Phytophthora ramorum-infected coast live oaks following bark and ambrosia beetle colonization in northern California. For. Ecol. Manag. 291, 30–42. doi: 10.1016/j.foreco.2012.11.010
Miller, M. A., Pfeiffer, W., and Schwartz, T. (2010). “Creating the CIPRES science gateway for inference of large phylogenetic trees,” in Paper Presented at: 2010 Gateway Computing Environments Workshop (GCE), (New Orleans, LA).
Montecchio, L., Fanchin, G., Simonato, M., and Faccoli, M. (2014). First record of thousand cankers disease fungal pathogen Geosmithia morbida and Walnut Twig Beetle Pityophthorus juglandis on Juglans regia in Europe. Plant Dis. 98:1445. doi: 10.1094/PDIS-07-14-0719-PDN
O’Donnell, K., and Cigelnik, E. (1997). Two Divergent Intragenomic rDNA ITS2 types within a monophyletic lineage of the fungus Fusarium are Nonorthologous. Mol. Phylogenet. Evol. 7, 103–116. doi: 10.1006/mpev.1996.0376
Pepori, A. L., Kolařík, M., Bettini, P. P., Vettraino, A. M., and Santini, A. (2015). Morphological and molecular characterisation of Geosmithia species on European elms. Fungal Biol. 119, 1063–1074. doi: 10.1016/j.funbio.2015.08.003
Pitt, J. I. (1979). Geosmithia gen. nov. for Penicillium lavendulum and related species. Can. J. Bot. 57, 2021–2030. doi: 10.1139/b79-252
Rehner, S. A., and Buckley, E. (2005). A Beauveria phylogeny inferred from nuclear ITS and EF1-α sequences: evidence for cryptic diversification and links to Cordyceps teleomorphs. Mycologia 97, 84–98. doi: 10.1080/15572536.2006.11832842
Ronquist, F., Teslenko, M., van der Mark, P., Ayres, D. L., Darling, A., Höhna, S., et al. (2012). MrBayes 3.2: efficient bayesian phylogenetic inference and model choice across a large model space. Syst. Biol. 61, 539–542. doi: 10.1093/sysbio/sys029
Seifert, K., De Beer, Z. W., and Wingfield, M. (2013). The Ophiostomatoid Fungi: Expanding Frontiers. Utrecht: CBS-KNAW Fungal Biodiversity Centre.
Seybold, S. J., Haugen, D., O’Brien, J., and Graves, A. D. (2013). Thousand Cankers Disease. USDA Forest Service, Northeastern Area State and Private Forestry Pest Alert NA-PR-02e10. Milwaukee, WI: USDA Forest Service.
Six, D. L., and Bentz, B. J. (2007). Temperature determines symbiont abundance in a multipartite bark beetle-fungus ectosymbiosis. Microb. Ecol. 54, 112–118. doi: 10.1007/s00248-006-9178-x
Skelton, J., Jusino, M. A., Li, Y., Bateman, C., Thai, P. H., Wu, C., et al. (2018). Detecting symbioses in complex communities: the fungal symbionts of bark and ambrosia beetles within Asian Pines. Microb. Ecol. 76, 839–850. doi: 10.1007/s00248-018-1154-8
Stamatakis, A. (2014). RAxML version 8: a tool for phylogenetic analysis and post-analysis of large phylogenies. Bioinformatics 30, 1312–1313. doi: 10.1093/bioinformatics/btu033
Stodůlková, E., Kolařík, M., Køesinová, Z., Kuzma, M., Šulc, M., Man, P., et al. (2009). Hydroxylated anthraquinones produced by Geosmithia species. Folia Microbiol. 54, 179–187. doi: 10.1007/s12223-009-0028-3
Strzałka, B., Kolařík, M., and Jankowiak, R. (2021). Geosmithia associated with hardwood-infesting bark and ambrosia beetles, with the description of three new species from Poland. Antonie Van Leeuwenhoek 114, 169–194. doi: 10.1007/s10482-020-01510-6
Tisserat, N., Cranshaw, W., Leatherman, D., Utley, C., and Alexander, K. (2009). Black walnut mortality in colorado caused by the walnut twig beetle and thousand cankers disease. Plant Health Prog. 10:10. doi: 10.1094/PHP-2009-0811-01-RS
Tisserat, N., Cranshaw, W., Putnam, M. L., Pscheidt, J., Leslie, C. A., Murray, M., et al. (2011). Thousand cankers disease is widespread in black walnut in the Western United States. Plant Health Prog. 12:35. doi: 10.1094/PHP-2011-0630-01-BR
Utley, C., Nguyen, T., Roubtsova, T., Coggeshall, M., Ford, T. M., Grauke, L. J., et al. (2012). Susceptibility of walnut and hickory species to Geosmithia morbida. Plant Dis. 97, 601–607. doi: 10.1094/PDIS-07-12-0636-RE
Vannini, A., Contarini, M., Faccoli, M., Dalla Valle, M., Morales-Rodríguez, C., Mazzetto, T., et al. (2017). First report of the ambrosia beetle Xylosandrus compactus and associated fungi in the Mediterranean maquis in Italy, and new host-pest associations. Bull. OEPP 47, 100–103. doi: 10.1111/epp.12358
Veselská, T., Skelton, J., Kostovčík, M., Hulcr, J., Baldrian, P., Chudíčková, M., et al. (2019). Adaptive traits of bark and ambrosia beetle-associated fungi. Fungal Ecol. 41, 165–176. doi: 10.1016/j.funeco.2019.06.005
White, T. J., Bruns, T., Lee, S., and Taylor, J. (1990). “Amplification and direct sequencing of fungal ribosomal RNA genes for phylogenetics,” in PCR Protocols: A Guide to Methods and Applications, Vol. 18, eds M. A. Innis, D. H. Gelfand, J. J. Sninsky, and T. J. White (New York, NY: Academic Press, Inc), 315–322.
Keywords: fungal community, symbiosis, 9 new taxa, Geosmithia, bark beetles
Citation: Zhang X, Li Y, Si H, Zhao G, Kolařík M, Hulcr J, Jiang X, Dai M and Chang R (2022) Geosmithia Species Associated With Bark Beetles From China, With the Description of Nine New Species. Front. Microbiol. 13:820402. doi: 10.3389/fmicb.2022.820402
Received: 23 November 2021; Accepted: 11 January 2022;
Published: 14 March 2022.
Edited by:
Peter H. W. Biedermann, University of Freiburg, GermanyReviewed by:
Pepijn Wilhelmus Kooij, Universidade Estadual Paulista, BrazilZhang Shouke, Zhejiang Agriculture and Forestry University, China
Copyright © 2022 Zhang, Li, Si, Zhao, Kolařík, Hulcr, Jiang, Dai and Chang. This is an open-access article distributed under the terms of the Creative Commons Attribution License (CC BY). The use, distribution or reproduction in other forums is permitted, provided the original author(s) and the copyright owner(s) are credited and that the original publication in this journal is cited, in accordance with accepted academic practice. No use, distribution or reproduction is permitted which does not comply with these terms.
*Correspondence: Meixue Dai, daimeixue@sdnu.edu.cn; Runlei Chang, changrunlei@163.com
†These authors have contributed equally to this work