- 1Key Laboratory of Desert and Desertification, Northwest Institute of Eco-Environment and Resources, Chinese Academy of Sciences, Lanzhou, China
- 2Key Laboratory of Extreme Environmental Microbial Resources and Engineering, Lanzhou, China
- 3Key Laboratory of Stress Physiology and Ecology in Cold and Arid Regions of Gansu Province, Lanzhou, China
- 4Gaolan Station of Agricultural and Ecological Experiment, Northwest Institute of Eco-Environment and Resources, Chinese Academy of Sciences, Lanzhou, China
- 5Institute of Plant Protection, Ningxia Academy of Agriculture and Forestry Sciences, Yinchuan, China
Plant pathogenic fungi such as Fusarium tricinctum cause various plant diseases worldwide, especially in temperate regions. In cereals, F. tricinctum is one of the most common species causing Fusarium head blight (FHB) and root rot. Infection with F. tricinctum results in high yield losses and reduction in quality, mainly due to mycotoxin contamination of grain. Mycotoxins produced by F. tricinctum, such as enniatins (ENs) and moniliformin (MON), which are the most studied mycotoxins, have been reported to have multiple toxic effects on humans and animals. Although chemical control of Fusarium infection has been applied to grains, it is not always effective in controlling disease or reducing the level of mycotoxins in wheat grains. To the contrary, chemical control may significantly increase infection of F. tricinctum in fungicide-treated plots after treatment. Our studies show that the bacterium Bacillus amyloliquefaciens, has good control effects against F. tricinctum. Therefore, its use as a biological control agent against various plant pathogens may be an effective strategy to control the spread of Fusarium pathogens. Here, we conduct a review of the literature involving this plant pathogen, its diversity, virulence, and methods to control.
Introduction
Fusarium tricinctum is one of the most economically important plant pathogens and toxin-producing filamentous fungi in cereals and many other crops in the world (Marasas et al., 1967; Chelkowski et al., 1989; Andersen et al., 1996; Bottalico and Perrone, 2002; Kosiak et al., 2003; O’Donnell et al., 2013, Wiśniewska et al., 2014; Shi et al., 2017). Distribution of Fusarium species is dependent on climate and the genus is frequently observed within suitable climatic conditions. Temperature is the main climatic factor that affects the occurrence and development of Fusarium diseases of crops, although its impact of the climatic factor is not independent of other environmental and host factors (Saremi et al., 1999; Doohan et al., 2003). Among Fusarium species, F. tricinctum is thermal-specific pathogen, and lower temperature is beneficial to its activity and growth (Yan and Nelson, 2020). In fact, F. tricinctum is common in temperate regions, and usually appears as saprophyte or facultative parasite (Chelkowski et al., 1989; Andersen et al., 1996; Kosiak et al., 2003).
F. tricinctum is related to Fusarium disease and prefer crop hosts, which is important to agriculture (Figures 1A–D). Studies have shown that F. tricinctum can potentially infect and colonize undamaged wheat leaves and produce conidia on senescent wheat leaves, resulting in wilt and “Fusarium head blight” (Wagacha et al., 2012), which can highly reduce crop yield. In addition, it is reported that F. tricinctum can produce mycotoxins as secondary metabolites, and the existence of mycotoxins in grains is a great worldwide concern. The presence of mycotoxins in feeds and foods is often associated with chronic or acute mycotoxin diseases in livestock and also in humans (Bottalico and Perrone, 2002).
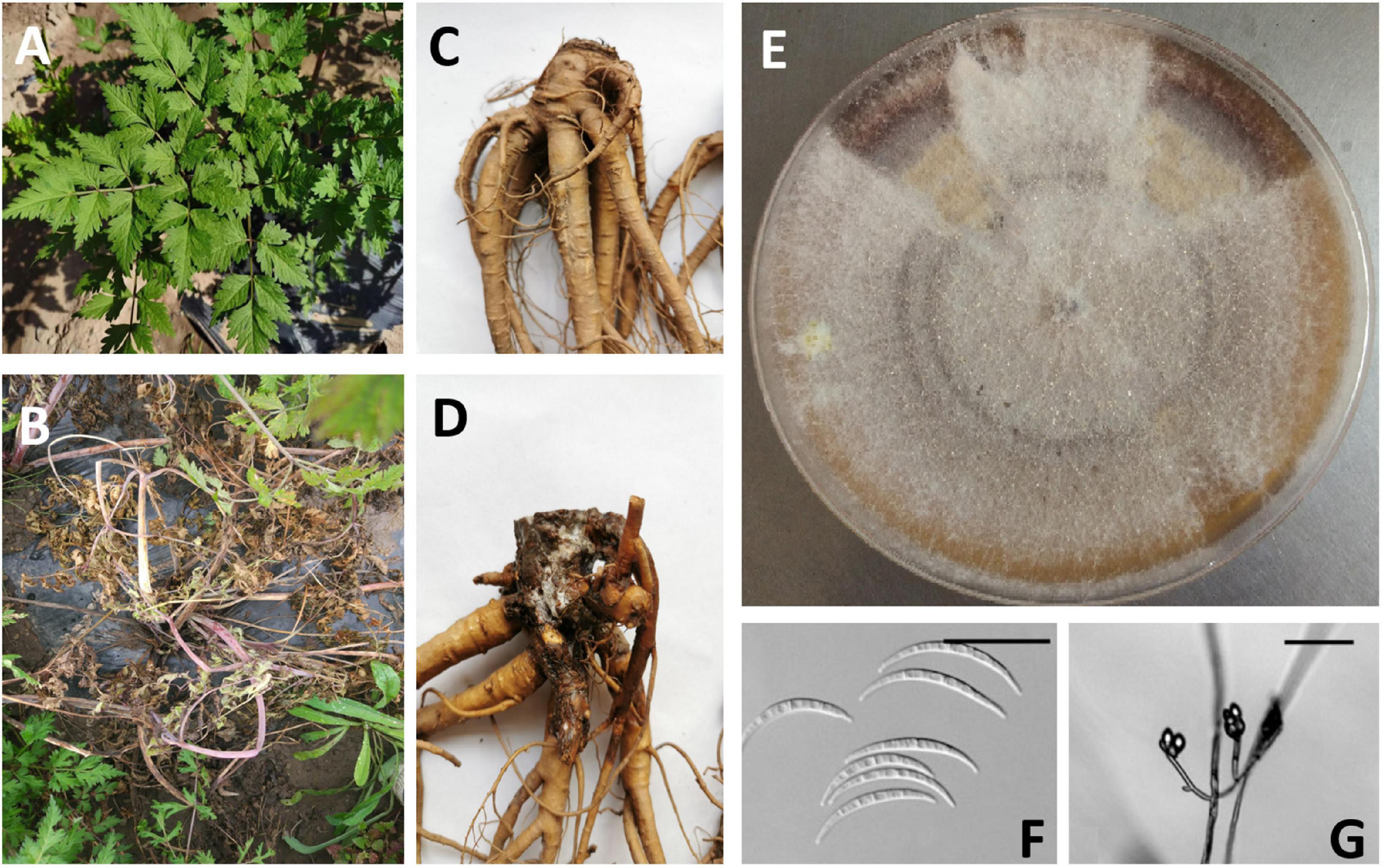
Figure 1. Observation of root rot symptom (Angelica sinensis) resulting from infection of F. tricinctum, above ground (A: healthy plant; B: infected plant) and below ground (C: healthy root; D: infected root); Colony of F. tricinctum on a PDA plate (E); Micrograph image of F. tricinctum macroconidia (F, scale bar = 25 μm) and Microconidia (G, scale bar = 50 μm). (A–E) Photos taken by Dr. Liu of our group; (F,G) borrowed from Leslie and Summerell (2006).
Research on chemical control of Fusarium infection in grains has been reported. For example, use of tebuconazole fungicide has been effective against these fungal pathogens (Mesterhazy et al., 2003). However, fungicides containing tebuconazole and azoxystrobin are not always effective in controlling Fusarium disease, or reducing the levels of their mycotoxins in wheat grain (Pirgozliev et al., 2002; Wegulo et al., 2010). In addition, compared with untreated plots, Fusarium infection significantly increased in fungicide-treated plots, where the frequency of F. tricinctum was most often increased (Henriksen and Elen, 2005). These shortcomings require the search for alternative strategies, including biological control methods, to inhibit the prevalence of Fusarium pathogens. However, Sphingomonas and Bacillus bacteria may offer effective biological control of Fusarium pathogens (Dunlap et al., 2011; Wachowska et al., 2013; Shang et al., 2016).
F. tricinctum strains are saprophytes and plant pathogens of a variety of hosts including wheat and barley, and increasingly have been reported throughout the world (Torbati et al., 2019; Yan and Nelson, 2020; Senatore et al., 2021). However, the systematic synthesis of knowledge on these plant pathogens and more practical biocontrol methods are currently lacking. In this review, we will cover recent insights into the distribution of F. tricinctum, examine current understanding in pathogenicity, and discuss the strategies to control the disease caused by the pathogenic fungi.
Taxonomy of Fusarium tricinctum
Corda first referred to F. tricinctum as Selenosporium “tricnictum,” and Saccardo transferred it to F. tricinctum, which are complex filamentous ascomycete fungi (Figures 1F,G), composed of many toxin-producing plant pathogens with important agricultural significance, then it was neotypified by Neish (1987). Holubová-Jechová et al. (1994) assigned epitypification for this species. F. tricinctum grow rapidly on PDA, forming a large number of dense mycelia that are initially white, but will become pink, red or purple with age, since they can form red pigments in agar (Figure 1E). F. tricinctum can be distinguished from some closely related species in terms of the macroconidial shape and the monophialidic conidiogenous cell. Cultures of F. tricinctum can be confused easily with F. graminearum, F. pseudograminearum, and F. culmorum (Leslie and Summerell, 2006). Differences in the morphology of the macroconidia allow the differentiation of F. tricinctum from F. graminearum, F. pseudograminearum, and F. culmorum. More importantly, as a member of the Section Sporotrichiella, the presence of microconidia distinguishes isolates of F. tricinctum from isolates of which form colonies on PDA similar to those of F. tricinctum. In addition, F. tricinctum cannot produce polyphialides, which is different from some close relatives (Leslie and Summerell, 2006).
F. tricinctum has a very close relationship with F. avenaceum, which together with other Fusarium tricinctum species complex (FTSC), are related with Fusarium head blight (FHB) and seedling diseases (stem and root rot) of all cereals (Bottalico and Perrone, 2002). FTSC members include isolates of F. avenaceum, F. flocciferum, F. petersiae, F. acuminatum, F. tricinctum, and other unclassified FTSC (NCBI Taxonomy, Figure 2). New members belonging to FTSC were described as F. gamsii and F. iranicum from Iran (Torbati et al., 2019), and as FTSC 12, 13, 14, 15 from Italy (Senatore et al., 2021). Members of the FTSC complex produce a number of “emerging” mycotoxins, including enniatins (ENs) and moniformin (MON) that may pose a threat to food safety and human health (Jestoi, 2008). Phylogenetic analysis has shown that the genetic relationship among these species is quite close (Turner et al., 1998), and it is very difficult to distinguish them from each other on the basis of morphological and physiological characteristics, but they can be distinguished by means of molecular methods. DNA sequence data from several marker loci have been used to resolve phylogenetic relationship within the FTSC (Figure 2), including ACL1 (ATP citrate lyase 1), TUB-2 (β-tubulin), ITS rDNA, ESYN1 (enniatin synthetase 1), RPB1 (RNA polymerase subunit 1, RPB2 (RNA polymerase subunit 2), and TEF1 (translation elongation factor 1α) (Turner et al., 1998; Kristensen et al., 2005; Kulik et al., 2007; Niessen et al., 2012; Senatore et al., 2021).
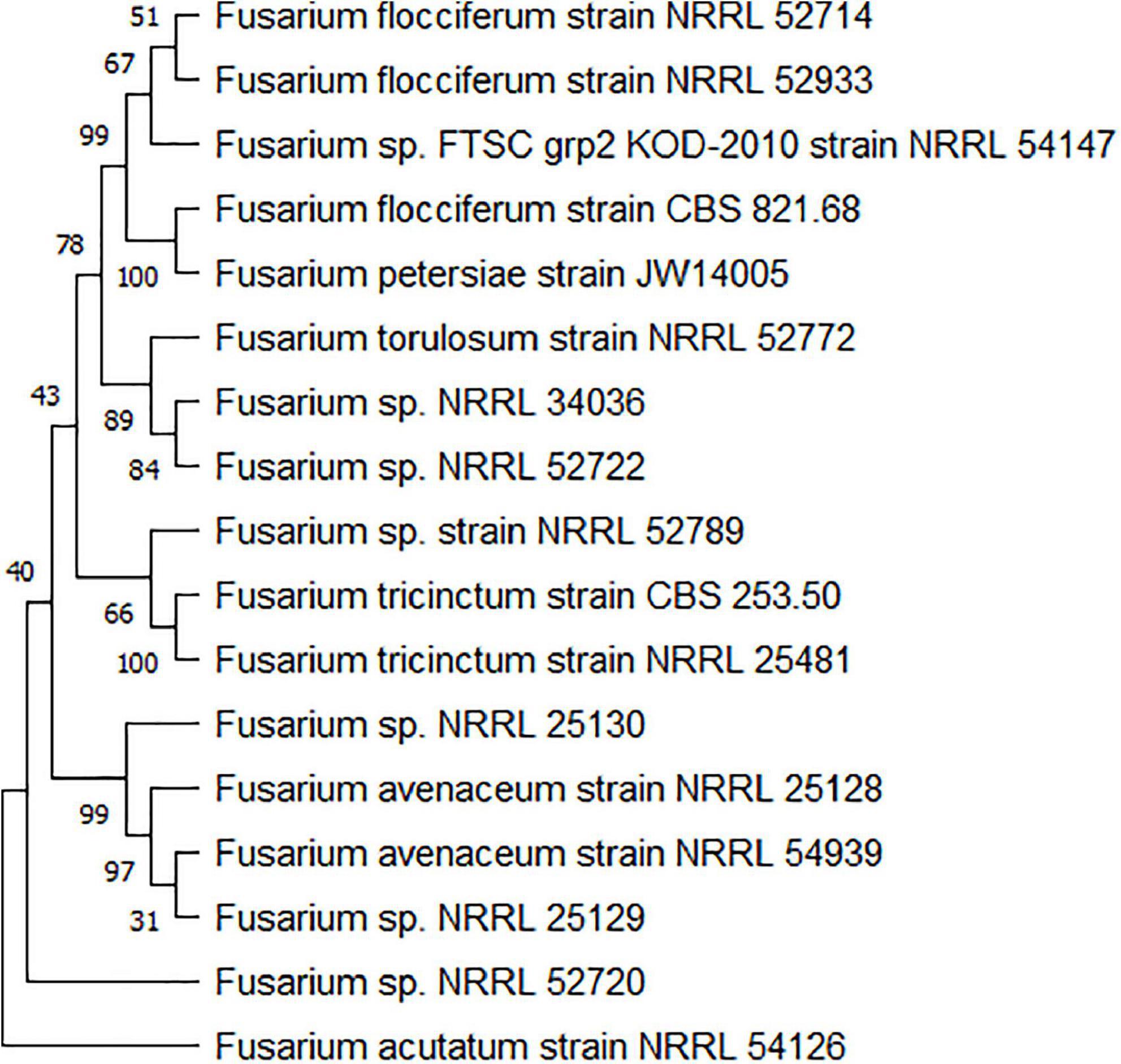
Figure 2. Maximum-likelihood (ML) phylograms obtained from the combined partial TEF and RPB2 data set (2,487 bp) of the Fusarium tricinctum species complex (FTSC) isolates. Sequences were used to conduct BLASTn queries of NCBI GenBank (https://www.ncbi.nlm.nih.gov/). Aligned sequences of 17 FTSC reference strains were combined and analyzed via ML bootstrapping using MEGA 11. Bootstrap values (%) are shown on clades.
The distribution and ecology of Fusarium tricinctum
F. tricinctum is plant pathogen all over the world that have the potential to infect and colonize various cereal crops, such as wheat, rice, maize, and oats in temperate and also semi-tropical cereal-growing areas, including Asia, North America, South Africa, and all Europe (Marasas et al., 1967; Lamprecht et al., 1988; Chelkowski et al., 1989; Andersen et al., 1996; Golinski et al., 1996; Kosiak et al., 2003; Wiśniewska et al., 2014; Shi et al., 2017). F. tricinctum was recently reported as plant pathogen in Argentina, Brazil, Western Australia, and South Australia (Castañares et al., 2010; Barkat et al., 2016; Moreira et al., 2019; Supplementary Table 1 and Figure 3).
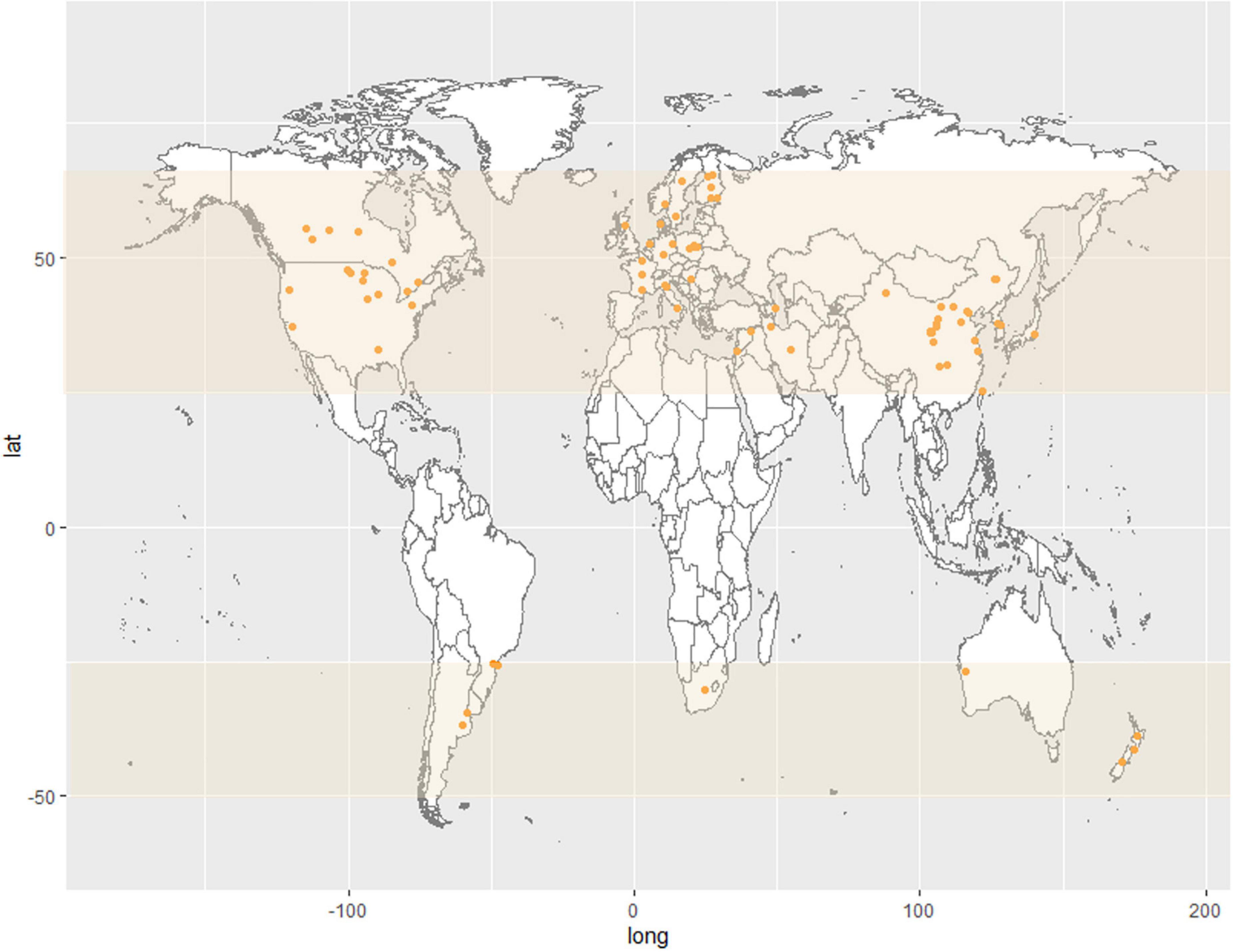
Figure 3. Map showing the global distribution (orange dots) of plant hosts with reported F. tricinctum infection. The distribution is limited to the temperate zone (covered in light yellow).
F. tricinctum was isolated from the diseased stem bases of wheat and was the most frequent species of Fusarium isolated from malted barley in Denmark (Andersen et al., 1996) and from winter wheat in Sweden (Lindblad et al., 2013). Also, it rarely occurred in pods and seeds of mature plants or residue (Nyvall, 1976). F. tricinctum is usually isolated from moldy corn, fescue, and most small grains (Marasas et al., 1967; Bamburg et al., 1968; Burmeister and Hesseltine, 1970). It is also the cause of postharvest rot of onion bulbs and pumpkins (Carrieri et al., 2013), as well as dry rot of seed tubers of potatoes in Michigan (Aktaruzzaman et al., 2018). In addition, F. tricinctum was found as endophytic species in healthy trees and healthy seedlings of Persian oak trees in Iran (Alidadi et al., 2019; Supplementary Table 1). Our work proved that F. tricinctum was the pathogen causing bulbs rotting and spalling from the basal disc, as well as progressive yellowing and defoliation of the leaves in lilies and Lanzhou lilies (Li et al., 2013; Shang et al., 2014). Interestingly, we also found that F. tricinctum was involved in root rot of Chinese herbal medicine, e.g., Angelica sinensis and wolfberry (Liu et al., 2021; Uwaremwe et al., 2022).
Geographic distribution of F. tricinctum appears to be related to climatic conditions, such as temperature and humidity. Climate is the main factor that influences the distribution of Fusarium in soil, and the effects of temperature on the colonization of roots and soil propagule density have also been experimentally determined to be a factor (Saremi et al., 1999). The Fusarium species differ in their climatic distribution and optimum climatic conditions are required for their persistence. Temperature and humidity are the main climatic factors that affect the occurrence of grain Fusarium diseases, although the influence of these climatic factors is not independent of other environmental and host factors. Conditions that are conductive to in vitro growth are usually the most favorable for production of mycotoxins in cereal crops (Doohan et al., 2003).
The incidence of the pathogenic organisms of wheat Fusarium, barley Fusarium, and maize ear rot are often related to different climatic conditions (temperature and rainfall) in different geographic locations. Temperature has a significant influence on Fusarium root rot of soybeans, and F. tricinctum is the major pathogen of the root rot disease and show obvious thermal-specific (Yan and Nelson, 2020). The cooler temperatures might be conductive to the activity of F. tricinctum (Yan and Nelson, 2020). Fusarium species are geographically distributed soil fungi because weather has an important influence on the abundance and activity of the species (Saremi et al., 1999; Doohan et al., 2003). F. tricinctum usually also appears as a saprophyte or a parasite of plants in temperate and semi-tropical regions (Figure 3; Chelkowski et al., 1989; Andersen et al., 1996; Kosiak et al., 2003).
Even before infecting the host, climate has the potential to affect the incidence and severity of Fusarium disease. Once the F. tricinctum inoculum disperses to the host, climate factors, temperature, and humidity play a vital role in the infection and colonization of grains by F. tricinctum (Doohan et al., 2003). Temperature or osmotic stress may indirectly affect the development of disease by inducing the host antifungal defensive mechanisms before attacking of pathogen (Conrath et al., 2002). Although in vitro growth tests showed that Fusarium have temperature ecotypes on the basis of species and origin location, in vitro pathogenicity tests suggested that species is much more important than climatic origin in determining the pathogenicity of Fusarium, regardless of the temperature (Brennan et al., 2003).
The pathogenicity from Fusarium tricinctum
F. tricinctum is related to Fusarium disease, which can reduce crop yield and cause the accumulation of mycotoxins in grain products. The potential of Fusarium to infect and colonize undamaged wheat leaves, and the potential to produce conidia on senescent leaves cultured were investigated. Studies have also shown that F. tricinctum forms sporophores and erupts through the leaf surface to release a large number conidia, resulting in wilt and FHB of cereal crops, such as wheat, oat, barley, and maize (Wagacha et al., 2012).
Many of Fusarium species are well-known for their mycotoxins produced as secondary metabolites, and the presence of mycotoxins in feeds and foods is often associated with chronic or acute mycotoxin diseases in livestock and also in humans. However, there is a great variability in the production of biologically active secondary metabolites between species (Visconti et al., 1992). The main groups mycotoxins commonly found in F. tricinctum are: ENs, MON, and T-2 toxin, which are currently covered by limited literature (Langseth, 1998; D’mello et al., 1999; Thrane, 2001; Jestoi, 2008). ENs, the most studied mycotoxins, produced by F. tricinctum are usually considered to be less toxic than trichothecenes and are known as a phytopathogenic compound causing necrosis and wilt (Cuomo et al., 2013). MON has been frequently purified from cultures of F. tricinctum, and the role of MON (a potassium or sodium salt of a cyclobutene) is to inhibit enzymatic systems and gluconeogenesis (Jestoi et al., 2004). The most reported trichothecenes mycotoxin in F. tricinctum is T-2 toxin, which is associated with acute toxicity (Prelusky et al., 1994). In addition, some of the mycotoxins may also have the potential to be applied as drugs or drug candidates. These mycotoxins include fusarielins (Hemphill et al., 2017), Visoltricin (Visconti et al., 1992), and ENs (Wätjen et al., 2009), which are medicinally used as antibiotics for the treatment of nasopharyngitis (Hemphill et al., 2017).
Enniatins (ENs) are a group of fungal mycotoxins with a hexadepsipeptidic chemical structure and they possess many potent biological activities that can contaminate a variety of foodstuffs increasing the exposure risk for consumers (Cuomo et al., 2013). Among FHB pathogens of cereals, F. tricinctum are the most effective ENs producers in naturally contaminated grain (Jestoi et al., 2004; Jestoi, 2008). The cyclic hexadepsipeptide compounds are known as phytopathogenic toxins from F. tricinctum causing symptoms such as necrosis lesions, rot, and wilt (Hornbogen et al., 2002; Figure 4). The molecule consists of three alternating residues each of a branched chain amino acid and D-hydroxyisovaleric acid (Supplementary Figure 1). ENs are synthesized by a 347 kDa multienzyme (EN synthetase), and the corresponding gene esynl has an open reading frame of 9,393 nucleotides (Hornbogen et al., 2002). The biological activities of the ENs are largely due to their ability to transfer cations through bilayer membranes without forming membrane pores (Jestoi, 2008). ENs are then integrated into cell membranes, forming passive cation-selective channels (Cuomo et al., 2013). ENs contribute to the wilt toxic character of F. tricinctum, and their virulence was significantly reduced after disruption of the esynl gene (Hornbogen et al., 2002). In addition to phytotoxicity, ENs show antimicrobial, insecticidal, herbicidal, and anthelminthic activities (Burmeister and Plattner, 1987; Herrmann et al., 1996; Jeschke et al., 2003; Uhlig et al., 2007), as well as high cytotoxicity to mammalian cells (Jestoi, 2008). ENs A1 and B1 and, to a lesser extent, enniatin B may possess anticarcinogenic properties by induction of apoptosis and disruption of extracellular regulated protein kinase signaling pathway. Further analysis of ENs is necessary to investigate their potential importance for cancer therapy (Wätjen et al., 2009).
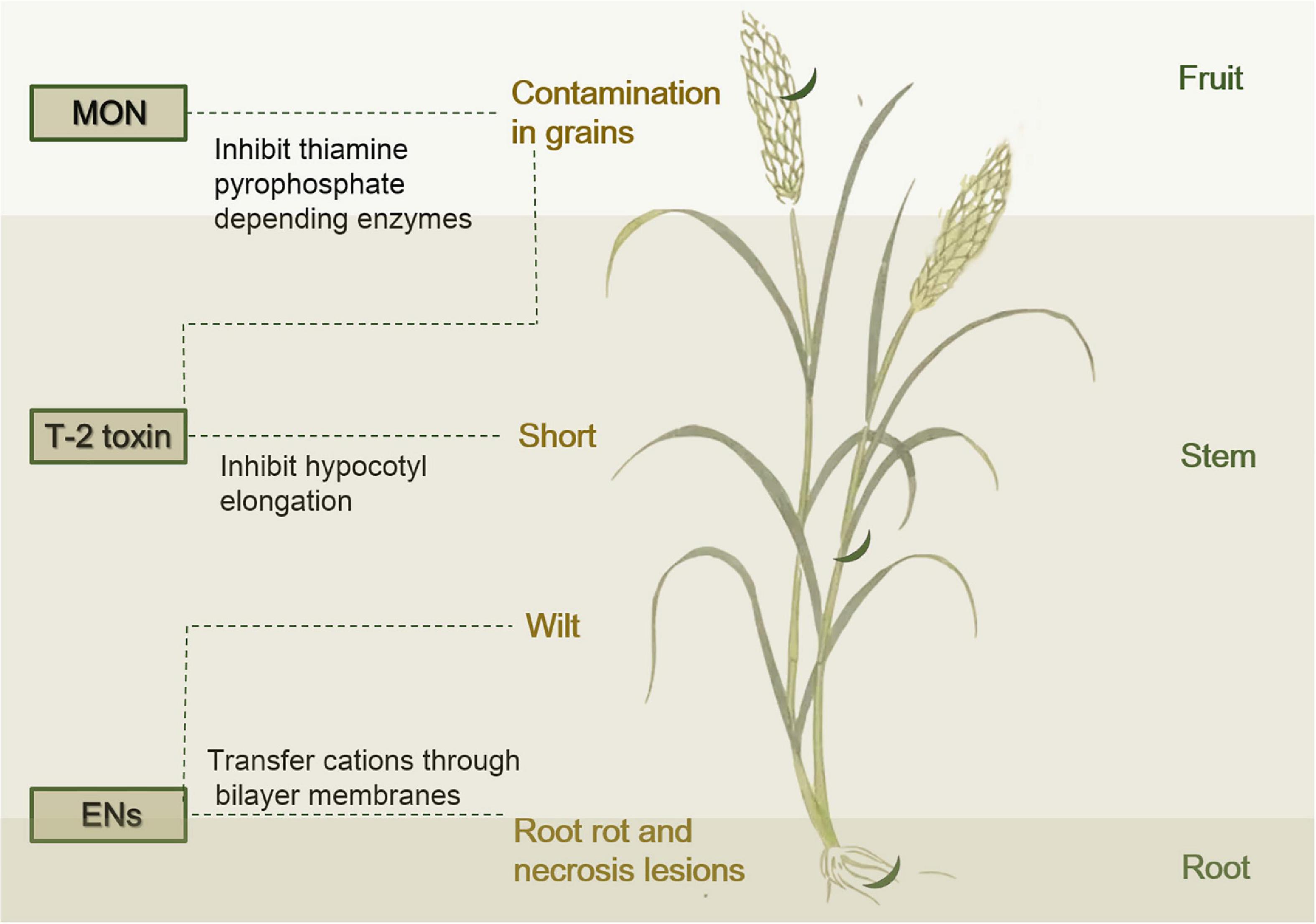
Figure 4. Diagram that F. tricinctum (crescent moon in dark green) infects a plant, releases mycotoxins and causes disease symptoms in plants.
Beauvericin (BEA) is a cyclodepsipeptide metabolite, closely related and co-occurred with ENs (Hornbogen et al., 2002; Supplementary Figure 1). BEA was currently reported to be associated with the presence of F. tricinctum (Hellin et al., 2016), and is a very potent channel-forming molecule as it induces pores in lipid membranes (Moretti et al., 2002). The non-selective toxic activity of BEA could be explained by its capacity to induce biological membrane pores, resulting in interference with the normal gradients of physiologically important monovalent cations on the cell membranes (Moretti et al., 2002). This process could determine the role of BEA in pathogenicity caused by F. tricinctum strains that produce the toxin. However, F. tricinctum strains do not produce very high levels of BEA (Uhlig et al., 2007). BEA is not only highly toxic to insects (Gupta et al., 1991), but also cytotoxic to mammalian cells and tissues, and has been reported to cause apoptosis in mouse and human cell lines (Macchia et al., 1995).
Moniliformin (MON) was structurally characterized and identified as the sodium salt of the semisquaric acid, and the relatively small semisquaric anion can be assumed to chemically behave similarly to inorganic anions (Supplementary Figure 2; Springer et al., 1974). Severe infections of F. tricinctum, were usually responsible for MON occurring in scabby grains, according to the surveys from some European countries (Kostecki et al., 1995). In Austria, MON occurred in freshly harvested durum wheat (Adler et al., 1995). The contamination MON in grains was closely associated with the presence of F. tricinctum in most surveys (Kostecki et al., 1995; Bottalico and Perrone, 2002; Figure 4). MON acts as an inhibitor of thiamine pyrophosphate depending enzymes, such as pyruvate dehydrogenase, ketoglutarate dehydrogenase, and pyruvate decarboxylase (Gathercole et al., 1986; Pirrung et al., 1996). In addition, MON was reported to inhibit glutathione peroxidase, aldose reductase, reductase, and gluconeogenesis (Deruiter et al., 1993; Wu and Vesonder, 1997). MON in diets was associated with reduced performance, hematological diseases, myocardial hypertrophy, and mortality in pigs and rodents (Harvey et al., 1997; Bottalico and Perrone, 2002), as well as muscular weakness and acidosis in poultry (Nagaraj et al., 1996). The major target organs of the mycotoxin are the cardiac and skeletal muscles (Harvey et al., 2002). Long-term exposure to MON has been associated with reduced weight gain, heart muscle damage, and disordered immune system in some laboratory animals (Harvey et al., 1997, 2002; Li et al., 2000). MON has been speculated to be associated with outbreaks of human Keshan disease in some areas of China where ingestion of MON-contaminated maize has caused cardiac lesions (Yu et al., 1995; Uhlig et al., 2007).
T-2 toxin belongs to type A trichothecenes, and is one of the most toxic mycotoxins (Prelusky et al., 1994). T-2 toxin is usually produced at low temperatures by the F. tricinctum (Burmeister, 1971). T-2 toxin can inhibit hypocotyl elongation of soybean (Figure 4). Although the inhibition of elongation by cytokinin was similar to that of T-2 toxin, the two compounds appeared to act in different ways (Stahl et al., 1973). Rats fed the T-2 toxin diet were severely stunted with inflammations of the skin around the mouth and nose (Burmeister, 1971), while a large animal (steer) that received daily intramuscular injections of T-2 toxin lost weight during the study and died after long-term treatment (Grove et al., 1970).
Fusarielins have received little attention in the Fusarium community as metabolite group. The ability of the Fusarium species to produce fusarielins is largely unknown. Fusarielins A and B were isolated from a F. tricinctum strain (Nenkep et al., 2010). Fusarielins have been shown to interfere with the microtubule function (Kobayashi et al., 1995). In antibacterial assays, it was found that fusarielins A and B were both mild antibiotics (Nguyen et al., 2007). Fusarielins have also been shown to have toxic effect on human epithelial carcinoma cell lines, and to be characterized as mycoestrogens, as they stimulate growth of MCF-7 breast cancer cells (Kobayashi et al., 1995; Sørensen et al., 2012).
On the other hand, the pathogenicity of F. tricinctum may be used to control the spread of invasive weeds. The annual herb Bromus tectorum (cheatgrass) has been becoming a serious invasive plant in semi-arid habitats of Northwestern America in winter. F. tricinctum isolated from the local soils is the pathogen of B. tectorum seeds, and is the main reason for the complete death of the invasive grass (Masi et al., 2017). F. tricinctum produce a large number of phytotoxins that participate in the pathogenesis (O’Donnell et al., 2013). Acuminatopyrone and blumenol A showed a significant inhibitory effect on the radicle length of cheatgrass seedlings (Masi et al., 2017). F. tricinctum that can cause B. tectorum infection and death show potential as biocontrol agents against invasive weeds (Masi et al., 2017).
Disease control to Fusarium tricinctum
Some studies have been reported on the chemical control of Fusarium infection in grains (Henriksen and Elen, 2005). Treatment of F. tricinctum cultures with dilute sodium bicarbonate can significantly reduce the production of trichothecene mycotoxins, geraniol, and carotenoids (Roinestad et al., 1994). Chlorine dioxide (ClO2) is a powerful disinfectant with a wide range of high biocidal activity. The treatment with aqueous ClO2 significantly reduced the populations of F. tricinctum and prevented the occurrence of chestnut kernel rot (Chen and Zhu, 2011). In addition, some natural plant extracts have the effect of inhibiting the growth of F. tricinctum under laboratory conditions. Curcuma longa extract is related to the destruction of the synthesis of key proteins and enzymes of fungal cell membrane systems, which may inhibit the synthesis of ergosterol and the respiratory chain, ultimately inhibiting the growth of F. tricinctum. Certain chemical components of C. longa have the potential to be developed as a series of environmentally sustainable bio-fungicides (Chen et al., 2018). The best results were obtained after artificial inoculation tests with fungicide tebuconazolein (Mesterhazy et al., 2003). However, compared with untreated plots, a significant increase of Fusarium infectious was detected in fungicide treated plots. F. tricinctum was the most frequent species detected after fungicide treating (Henriksen and Elen, 2005). The effect of fungicides on Fusarium grain infection was studied in the Norway field trial. Significant increase in Fusarium infection was detected in fungicide-treated plots compared with untreated plots. The level of the dominating species (F. tricinctum) increased after fungicide application. Other Fusarium species were detected only in low frequencies. In the fungicide trials, Diamant (epoxyconazole + kresoximmetyl) and F9215 (spiroketalamin + tebuconazole) increased F. tricinctum significantly, while there were no significant effects on the other Fusarium species (Henriksen and Elen, 2005). According to some reports, fungicides containing tebuconazole and azoxystrobin were not always effective in controlling Fusarium disease in wheat or reducing mycotoxin levels in grain products (Pirgozliev et al., 2002; Wegulo et al., 2010). These shortcomings require the search for alternative strategies, including biological methods, to control the spread of Fusarium pathogens.
Diverse microorganisms may contribute to the biological control of plant pathogenic microbes, and most research work has focused on isolates of some bacteria genera (Mcspadden Gardener and Driks, 2004). The controlling effects of Sphingomonas and Bacillus on winter wheat colonization pathogens, were studied under laboratory conditions. The Sphingomonas S 11 isolate has an antagonistic effect on F. tricinctum. The infection symptoms of winter wheat seedlings treated with a suspension of Sphingomonas S 11 bacteria and inoculated with Fusarium pathogens were significantly lower than those of unprotected seedlings that were inoculated with the above mentioned pathogens (Wachowska et al., 2013).
Bacillus can produce a broad spectrum of antimicrobial compounds, and this activity makes them candidates as biological control agents against a variety of plant pathogens (Mannanov and Sattarova, 2001; Mcspadden Gardener and Driks, 2004). The biological activity of these strains is often related to the production of secondary metabolites, such as antimicrobial cyclic lipopeptides (Dunlap et al., 2011). Our research shows that Bacillus amyloliquefaciens has good control effects on root rot infested by F. tricinctum in Chinese herbal medicine of Angelica sinensis, Lanzhou Lilies, and wolfberries (Shang et al., 2016; Liu et al., 2021; Uwaremwe et al., 2022). B. amyloliquefaciens strains were found to inhibit F. tricinctum fungal mycelial growth, in vitro and in planta, as well as to promote the growth of seedlings (Liu et al., 2021; Uwaremwe et al., 2022).
Whether organic farming can control the activity of F. tricinctum has not been reported. Bernhoft et al. (2010) found that the infection rate of F. tricinctum in organically produced wheat was lower, and the infestation and mycotoxin levels of Fusarium found in organic grains was lower as well (Bernhoft et al., 2010). However, it remains unclear whether organic amendments technology is effective in disease control and suppression, and farmers have often neglected its role in disease management. The control of onion pink rot by organic amendments is not easy to predict and apply on a large scale (Carrieri et al., 2013). In addition, organic solarization amendments cannot completely eliminate the Fusarium population in the soil, as evidenced by the existence of several infectious onion bulbs in solarized treatments (Carrieri et al., 2013). The agricultural intensity index includes the application amount rate of pesticide and nitrogen fertilizer, which may reflect some important differences between organic and conventional agricultural systems. It has been reported that agricultural intensity has an obvious impact on the community structure of Fusarium in wheat grains. Importantly, agricultural intensity increased the abundance of F. tricinctum (Karlsson et al., 2017).
The agroforestry system is a multi-functional plant production system and has attracted attention as a sustainable method that can replace traditional monoculture agriculture. The colonization rate of wheat grain in agroforestry combined with the FHB pathogens F. tricinctum was lower than that in conventional monoculture. Therefore, the biological control of F. tricinctum in wheat grain may be enhanced due to diversification under agroforestry practices (Beule et al., 2019). In addition, altering the microclimate conditions may inhibit the infection of F. tricinctum in the diversification of agroforestry systems.
Interactions with other Fusarium species
A full-scale understanding of the interactions between Fusarium species in grains gives us a better view of the ecological role of F. tricinctum, which is greatly important for limiting Fusarium disease and mycotoxin contamination in crops. There may be both synergistic and competitive interactions within Fusarium communities, F. poae and F. tricinctum, the pair of Fusarium species co-existing in Swedish farmland (Karlsson et al., 2017). F. tricinctum and F. langsethiae were highly correlated in mature cereals in Belgium (Hellin et al., 2016). Two Fusarium species prefer to share the same environmental conditions instead of direct inter-species interactions, that is, symbiosis mode may be reliant upon the external associations. In addition, the co-inoculation of multiple Fusarium species led to competition in a controlled experiment, and the competitive interaction resulted in a decrease in fungal biomass and an increase in the amount of mycotoxin (Xu et al., 2007).
Management of Fusarium disease is complicated, due to the complexity of Fusarium species involved in an infection. Paired Fusarium cultures have different interactions between different isolates (Wagacha et al., 2012). The interaction between species may partly rely on the type of mycotoxins produced during the infection process (Llorens et al., 2006). Compared with deoxynivalenol (DON), ENs is much less phytotoxic to wheat and may has different effects on competing microorganisms. The individual mycotoxins may cause relative competition between species under certain circumstances. However, it is worth noting that other factors, including host plant species, climate factors, and other environmental conditions, may also play an important role in the interaction between Fusarium species (Llorens et al., 2006; Wagacha et al., 2012).
Future prospects
Fusarium disease is destructive for crops. The quality of grains deteriorated due to contamination by a series of mycotoxins produced by Fusarium. Although the disease has economic significance, disease control and prediction are still difficult due to the variety of Fusarium species involved. Different species may have different responses to different control measures, and also may have different interactions (competitive or synergistic) between species. Therefore, a comprehensive understanding of the ecological role of F. tricinctum at the community level is important in agricultural practices.
Such exploratory work requires relatively large amount of investment and may lead to the improvement of powerful new facilities for the research and application of Bacillus-mediated biological control. Obviously, this is an exciting time for basic research on plant–microbe interactions and microbiological ecology, as well as for efforts to improve agricultural technologies. We hope that this information will stimulate new research and will eventually lead to the wider application of safe and effective biocontrol agents, thereby promoting plant health.
Author contributions
YW: literature collection, processing, interpretation, writing, and submitting. YS: sequences blasting and phylogenetic analysis. RW: project coordination and supervising. All authors contributed to the article and approved the submitted version.
Funding
This work was supported by the National Key Research and Development Project (2018YFE0127200), the Key Research and Development Projects of Ningxia Hui Autonomous Region (2022BBF02031), the Gansu Province Science and Technology Major Special Plan (21ZD4NA019),rom the basal disc, a and the Foundation of State Key Laboratory of Permafrost Engineering (SKLFSE201610).
Conflict of interest
The authors declare that the research was conducted in the absence of any commercial or financial relationships that could be construed as a potential conflict of interest.
Publisher’s note
All claims expressed in this article are solely those of the authors and do not necessarily represent those of their affiliated organizations, or those of the publisher, the editors and the reviewers. Any product that may be evaluated in this article, or claim that may be made by its manufacturer, is not guaranteed or endorsed by the publisher.
Supplementary material
The Supplementary Material for this article can be found online at: https://www.frontiersin.org/articles/10.3389/fmicb.2022.939927/full#supplementary-material
References
Adler, A., Lew, H., Brodacz, W., Edinger, W., and Oberforster, M. (1995). Occurrence of moniliformin, deoxynivalenol, and zearalenone in dumm wheat (Triticum durum Desf.). Mycotoxin Res. 11, 9–15. doi: 10.1007/BF03192056
Aktaruzzaman, M., Afroz, T., Lee, Y.-G., and Kim, B.-S. (2018). Morphological and molecular characterization of Fusarium tricinctum causing postharvest fruit rot of pumpkin in Korea. J. Gen. Plant Pathol. 84, 407–413.
Alidadi, A., Kowsari, M., Javan-Nikkhah, M., Jouzani, G. R. S., and Rastaghi, M. E. (2019). New pathogenic and endophytic fungal species associated with Persian oak in Iran. Eur. J. Plant Pathol. 155, 1017–1032.
Andersen, B., Thrane, U., Svendsen, A., and Rasmussen, I. A. (1996). Associated field mycobiota on malt barley. Can. J. Bot. 74, 854–858.
Bamburg, J. R., Riggs, N. V., and Strong, F. M. (1968). The structures of toxins from two strains of Fusarium tricinctum. Tetrahedron 24, 3329–3336. doi: 10.1016/s0040-4020(01)92631-6
Barkat, E. H., Hardy, G. E. S. J., Ren, Y., Calver, M., and Bayliss, K. L. (2016). Fungal contaminants of stored wheat vary between Australian states. Austral. Plant Pathol. 45, 621–628.
Bernhoft, A., Clasen, P. E., Kristoffersen, A. B., and Torp, M. (2010). Less Fusarium infestation and mycotoxin contamination in organic than in conventional cereals. Food Addit. Contaminants A 27, 842–852. doi: 10.1080/19440041003645761
Beule, L., Lehtsaar, E., Rathgeb, A., and Karlovsky, P. (2019). Crop diseases and mycotoxin accumulation in temperate agroforestry systems. Sustainability 11:2925.
Bottalico, A., and Perrone, G. (2002). “Toxigenic Fusarium species and mycotoxins associated with head blight in small-grain cereals in Europe,” in Mycotoxins in Plant Disease: Under the aegis of COST Action 835 ‘Agriculturally Important Toxigenic Fungi 1998-2003’, EU project (QLK 1-CT-1998-01380), and ISPP ‘Fusarium Committee’, eds A. Logrieco, J. A. Bailey, L. Corazza, and B. M. Cooke (Dordrecht: Springer Netherlands), 611–624.
Brennan, J. M., Fagan, B., Van Maanen, A., Cooke, B. M., and Doohan, F. M. (2003). Studies on in vitro growth and pathogenicity of Fusarium fungi. Eur. J. Plant Pathol. 109, 577–587.
Burmeister, H. R. (1971). T-2 toxin production by Fusarium tricinctum on solid substrate. Appl. Microbiol. 21, 739–742. doi: 10.1128/am.21.4.739-742.1971
Burmeister, H. R., and Hesseltine, C. W. (1970). Biological assays for two mycotoxins produced by Fusarium tricinctum. Appl. Microbiol. 20:437. doi: 10.1128/am.20.3.437-440.1970
Burmeister, H. R., and Plattner, R. D. (1987). Enniatin production by Fusarium tricinctum and its effect on germinating wheat seeds. Phytopathology 77, 1483–1487.
Carrieri, R., Raimo, F., Pentangelo, A., and Lahoz, E. (2013). Fusarium proliferatum and Fusarium tricinctum as causal agents of pink rot of onion bulbs and the effect of soil solarization combined with compost amendment in controlling their infections in field. Crop Protect. 43, 31–37.
Castañares, E., Stenglein, S. A., Dinolfo, M. I., and Moreno, M. V. (2010). Fusarium tricinctum associated with head blight on wheat in Argentina. Plant Dis. 95:496. doi: 10.1094/PDIS-07-10-0485
Chelkowski, J., Manka, M., Kwasna, H., Visconti, A., and Golinski, P. (1989). Fusarium sporotrichioides Sherb, Fusarium tricinctum (Corda) Sacc and Fusarium poae (Peck) wollenw - cultural-characteristics, toxinogenicity and pathogenicity towards cereals. J. Phytopathol. Phytopathol. Z. 124, 155–161.
Chen, C., Long, L., Zhang, F., Chen, Q., Chen, C., Yu, X., et al. (2018). Antifungal activity, main active components and mechanism of Curcuma longa extract against Fusarium graminearum. PLoS One 13:e0194284. doi: 10.1371/journal.pone.0194284
Chen, Z., and Zhu, C. (2011). Modelling inactivation by aqueous chlorine dioxide of Dothiorella gregaria Sacc. and Fusarium tricinctum (Corda) Sacc. spores inoculated on fresh chestnut kernel. Lett. Appl. Microbiol. 52, 676–684. doi: 10.1111/j.1472-765X.2011.03061.x
Conrath, U., Pieterse, C. M. J., and Mauch-Mani, B. (2002). Priming in plant–pathogen interactions. Trends Plant Sci. 7, 210–216.
Cuomo, V., Randazzo, A., Meca, G., Moretti, A., Cascone, A., Eriksson, O., et al. (2013). Production of enniatins A, A1, B, B1, B4, J1 by Fusarium tricinctum in solid corn culture: structural analysis and effects on mitochondrial respiration. Food Chem. 140, 784–793. doi: 10.1016/j.foodchem.2012.10.136
Deruiter, J., Jacyno, J. M., Cutler, H. G., and Davis, R. A. (1993). Studies on aldose reductase inhibitors from fungi. II. Moniliformin and small ring analogues. J. Enzyme Inhibition 7, 249–256.
D’mello, J. P. F., Placinta, C. M., and Macdonald, A. M. C. (1999). Fusarium mycotoxins: a review of global implications for animal health, welfare and productivity. Anim. Feed Sci. Technol. 80, 183–205.
Doohan, F. M., Brennan, J., and Cooke, B. M. (2003). “Influence of climatic factors on Fusarium species pathogenic to cereals,” in Epidemiology of Mycotoxin Producing Fungi: Under the Aegis of COST Action 835 ‘Agriculturally Important Toxigenic Fungi 1998–2003’, EU project (QLK 1-CT-1998–01380), eds X. Xu, J. A. Bailey, and B. M. Cooke (Dordrecht: Springer Netherlands), 755–768.
Dunlap, C. A., Schisler, D. A., Price, N. P., and Vaughn, S. F. (2011). Cyclic lipopeptide profile of three Bacillus subtilis strains; antagonists of Fusarium head blight. J. Microbiol. 49, 603–609. doi: 10.1007/s12275-011-1044-y
Gathercole, P. S., Thiel, P. G., and Hofmeyr, J. H. (1986). Inhibition of pyruvate dehydrogenase complex bymoniliformin. Biochem. J. 233, 719–723.
Golinski, P., Perkowski, J., Kostecki, M., Grabarkiewicz-Szczesna, J., and Chelkowski, J. (1996). Fusarium species and Fusarium toxins in wheat in Poland-a comparison with neighbour countries. Sydowia-Horn- 48, 12–22.
Grove, M. D., Yates, S. G., Tallent, W. H., Ellis, J. J., Wolff, I. A., Kosuri, N. R., et al. (1970). Mycotoxins produced by Fusariumn tricinctuin as possible causes of cattle disease. J. Agric. Food Chem. 18, 734–736.
Gupta, S., Krasnoff, S. B., Underwood, N. L., Renwiek, J. A. A., and Roberts, D. W. (1991). Isolation of beauvericin as an insect toxin from Fusarium semitectum and Fusarium moniliforme var. subglutinans. Mycopathologia 115, 185–189. doi: 10.1007/BF00462223
Harvey, R. B., Edrington, T. S., Kubena, L. F., Rottinghaus, G. E., Turk, J. R., Genovese, K. J., et al. (2002). Toxicity of fumonisin from Fusarium verticillioides culture material and moniliformin from Fusarium fujikuroi culture material when fed singly and in combination to growing barrows. J. Food Protect. 65, 373–377. doi: 10.4315/0362-028x-65.2.373
Harvey, R. B., Kubena, R. F., Rottinghaus, G. E., Turk, J. R., and Buckley, S. A. (1997). Effects of fumonisin and moniliformin from culture materials to growing swine. Cereal Res. Commun. 25, 415–417.
Hellin, P., Dedeurwaerder, G., Duvivier, M., Scauflaire, J., Huybrechts, B., Callebaut, A., et al. (2016). Relationship between Fusarium spp. diversity and mycotoxin contents of mature grains in southern Belgium. Food Addit. Contaminants A 33, 1228–1240. doi: 10.1080/19440049.2016.1185900
Hemphill, C. F. P., Sureechatchaiyan, P., Kassack, M. U., Orfali, R. S., Lin, W., Daletos, G., et al. (2017). OSMAC approach leads to new fusarielin metabolites from Fusarium tricinctum. J. Antibiot. 70, 726–732. doi: 10.1038/ja.2017.21
Henriksen, B., and Elen, O. (2005). Natural Fusarium grain infection level in wheat, barley and oat after early application of fungicides and herbicides. J. Phytopathol. 153, 214–220.
Herrmann, M., Zocher, R., and Haese, A. (1996). Enniatin production by Fusarium strains and its effect on potato tuber tissue. Appl. Environ. Microbiol. 62, 393–398. doi: 10.1128/aem.62.2.393-398.1996
Holubová-Jechová, V., Gams, W., and Nirenberg, H. (1994). Revisiones generum obscurorum hyphomycetum: a revision of the selenosporium species described by ACJ Corda. Sydowia 46, 247–256.
Hornbogen, T., Glinski, M., and Zocher, R. (2002). Biosynthesis of depsipeptide mycotoxins in Fusarium. Eur. J. Plant Pathol. 108, 713–718.
Jeschke, P., Benet-Buchholz, J., Harder, A., Etzel, W., Schindler, M., and Thielking, G. (2003). Synthesis and anthelmintic activity of cyclohexadepsipeptides with (S,S,S,R,S,R)-configuration. Bioorgan. Med. Chem. Lett. 13, 3285–3288. doi: 10.1016/s0960-894x(03)00688-7
Jestoi, M. (2008). Emerging Fusarium-mycotoxins fusaproliferin, beauvericin, enniatins, and moniliformin-a review. Crit. Rev. Food Sci. Nutr. 48, 21–49. doi: 10.1080/10408390601062021
Jestoi, M., Rokka, M., Yli-Mattila, T., Parikka, P., Rizzo, A., and Peltonen, K. (2004). Presence and concentrations of the Fusarium-related mycotoxins beauvericin, enniatins and moniliformin in finnish grain samples. Food Addit. Contaminants 21, 794–802. doi: 10.1080/02652030410001713906
Karlsson, I., Friberg, H., Kolseth, A.-K., Steinberg, C., and Persson, P. (2017). Agricultural factors affecting Fusarium communities in wheat kernels. Int. J. Food Microbiol. 252, 53–60. doi: 10.1016/j.ijfoodmicro.2017.04.011
Kobayashi, H., Sunaga, R., Furihata, K., Morisaki, N., and Iwasaki, S. (1995). Isolation and structures of an antifungal antibiotic, fusarielin A, and related compounds produced by a Fusarium sp. J. Antibiotics 48, 42–52. doi: 10.7164/antibiotics.48.42
Kosiak, B., Torp, M., Skjerve, E., and Thrane, U. (2003). The prevalence and distribution of Fusarium species in Norwegian cereals: a survey. Acta Agric. Scand. B Soil Plant Sci. 53, 168–176.
Kostecki, M., Szczesna, J., Chelkowski, J., and And Wisniewska, H. (1995). Beauvericin and moniliformin production by Polish isolates of Fusarium subglutinans and natural co-occurrence of both mycotoxins in maize samples. Microbiol. Aliments Nutr. 13, 67–70.
Kristensen, R., Torp, M., Kosiak, B., and Holst-Jensen, A. (2005). Phylogeny and toxigenic potential is correlated in Fusarium species as revealed by partial translation elongation factor 1 alpha gene sequences. Mycol. Res. 109, 173–186. doi: 10.1017/s0953756204002114
Kulik, T., Pszczółkowska, A., Fordoński, G., and Olszewski, J. (2007). PCR approach based on the esyn1 gene for the detection of potential enniatin-producing Fusarium species. Int. J. Food Microbiol. 116, 319–324. doi: 10.1016/j.ijfoodmicro.2007.02.003
Lamprecht, S. C., Marasas, W. F. O., Vanwyk, P. S., and Knoxdavies, P. S. (1988). Fusarium-tricinctum (Fungi, Hyphomycetes) in South Africa - morphology and pathogenicity. Bothalia 18, 189–194.
Langseth, W. (1998). Mycotoxin production and cytotoxicity of Fusarium strains isolated from Norwegian cereals. Mycopathologia 144, 103–113. doi: 10.1023/a:1007016820879
Leslie, J. F., and Summerell, B. A. (2006). The Fusarium Laboratory Manual. Ames: Blackwell Publishing.
Li, Y. C., Ledoux, D. R., Bermudez, A. J., Fritsche, K. L., and Rottinghaust, G. E. (2000). Effects of moniliformin on performance and immune function of broiler chicks. Poult. Sci. 79, 26–32. doi: 10.1093/ps/79.1.26
Li, Y. Y., Wang, Y. J., Xie, Z. K., Wang, R. Y., Qiu, Y., Pan, H. Q., et al. (2013). First report of lily blight and wilt caused by Fusarium tricinctum in China. Plant Dis. 97:993. doi: 10.1094/PDIS-11-12-1010-PDN
Lindblad, M., Gidlund, A., Sulyok, M., Börjesson, T., Krska, R., Olsen, M., et al. (2013). Deoxynivalenol and other selected Fusarium toxins in Swedish wheat — Occurrence and correlation to specific Fusarium species. Int. J. Food Microbiol. 167, 284–291.
Liu, Y., Tian, Y., Yue, L., Constantine, U., Zhao, X., Zhou, Q., et al. (2021). Effectively controlling Fusarium root rot disease of Angelica sinensis and enhancing soil fertility with a novel attapulgite-coated biocontrol agent. Appl. Soil Ecol. 168:104121.
Llorens, A., Hinojo, M. J., Mateo, R., Medina, A., Valle-Algarra, F. M., González-Jaén, M. T., et al. (2006). Variability and characterization of mycotoxin-producing Fusarium spp isolates by PCR-RFLP analysis of the IGS-rDNA region. Antonie Van Leeuwenhoek 89, 465–478. doi: 10.1007/s10482-005-9045-7
Macchia, L., Di Paola, R., Fornelli, F., Nenna, S., Moretti, A., Napoletano, R., et al. (1995). “Cytotoxicity of beauvericin to mammalian cells,” in Proceedings of the lnternational Seminar on ‘Fusarium: Mycotoxins, Taxonomy and Pathogenicity’, Martina Franca, 72–73.
Mannanov, R. N., and Sattarova, R. K. (2001). Antibiotics produced by Bacillus bacteria. Chem. Nat. Compounds 37, 117–123.
Marasas, W. F. O., Smalley, E. B., Degurse, P. E., Bamburg, J. R., and Nichols, R. E. (1967). Acute toxicity to rainbow trout (Salmo Gairdnerii) of a metabolite produced by fungus Fusarium tricinctum. Nature 214, 817–818.
Masi, M., Meyer, S., Pescitelli, G., Cimmino, A., Clement, S., Peacock, B., et al. (2017). Phytotoxic activity against Bromus tectorum for secondary metabolites of a seed-pathogenic Fusarium strain belonging to the F. tricinctum species complex. Nat. Product Res. 31, 2768–2777. doi: 10.1080/14786419.2017.1297445
Mcspadden Gardener, B. B., and Driks, A. (2004). Overview of the nature and application of biocontrol microbes: Bacillus spp. Phytopathology 94:1244. doi: 10.1094/PHYTO.2004.94.11.1244
Mesterhazy, A., Barto, K. T., and Lamper, C. (2003). Influence of wheat cultivar, species of Fusarium, and isolate aggressiveness on the efficacy of fungicides for control of Fusarium head blight. Plant Dis. 87, 1107–1115. doi: 10.1094/PDIS.2003.87.9.1107
Moreira, G. M., Machado, F. J., Pereira, C. B., Neves, D. L., Tessmann, D. J., Ward, T. J., et al. (2019). First report of the Fusarium tricinctum species complex causing Fusarium head blight of wheat in Brazil. Plant Dis. 104:586.
Moretti, A., Belisario, A., Tafuri, A., Ritieni, A., Corazza, L., and Logrieco, A. (2002). Production of beauvericin by different races of Fusarium oxysporum F. sp. melonis, the Fusarium wilt agent of muskmelon. Eur. J. Plant Pathol. 108, 661–666.
Nagaraj, R. Y., Wu, W., Will, J. A., and Vesonder, R. F. (1996). Acute cardiotoxicity of moniliformin in broiler chickens as measured by electrocardiography. Avian Dis. 40, 223–227.
Nenkep, V., Yun, K., Zhang, D., Choi, H. D., Kang, J. S., and Son, B. W. (2010). Induced production of bromomethylchlamydosporols A and B from the marine-derived fungus Fusarium tricinctum. J. Nat. Products 73, 2061–2063. doi: 10.1021/np1005289
Nguyen, H. P., Zhang, D. H., Lee, U., Kang, J. S., Choi, H. D., and Son, B. W. (2007). Dehydroxychlorofusarielin B, an antibacterial polyoxygenated decalin derivative from the marine-derived fungus Aspergillus sp. J. Nat. Products 70, 1188–1190. doi: 10.1021/np060552g
Niessen, L., Gräfenhan, T., and Vogel, R. F. (2012). ATP citrate lyase 1 (acl1) gene-based loop-mediated amplification assay for the detection of the Fusarium tricinctum species complex in pure cultures and in cereal samples. Int. J. Food Microbiol. 158, 171–185. doi: 10.1016/j.ijfoodmicro.2012.06.021
O’Donnell, K., Rooney, A. P., Proctor, R. H., Brown, D. W., McCormick, S. P., Ward, T. J., et al. (2013). Phylogenetic analyses of RPB1 and RPB2 support a middle Cretaceous origin for a clade comprising all agriculturally and medically important fusaria. Fungal Genet. Biol. 52, 20–31. doi: 10.1016/j.fgb.2012.12.004
Pirgozliev, S. R., Edwards, S. G., Hare, M. C., and Jenkinson, P. (2002). Effect of dose rate of azoxystrobin and metconazole on the development of Fusarium head blight and the accumulation of deoxynivalenol (DON) in wheat grain. Eur. J. Plant Pathol. 108, 469–478.
Pirrung, M. C., Nauhaus, S. K., and Singh, B. (1996). Cofactor-directed, timedependent inhibition of thiamine enzymes by the fungal toxin moniliformin. J. Organ. Chem. 61, 2592–2593. doi: 10.1021/jo950451f
Prelusky, D. B., Rotter, B. A., and Rotter, R. G. (1994). “Mycotoxins in grain. Compounds other than aflatoxin,” in Toxicology of Mycotoxins, eds J. D. Miller and H. L. Trenholm (St. Paul: Eagan Press), 359–404.
Roinestad, K. S., Montville, T. J., and Rosen, J. D. (1994). Mechanism for sodium bicarbonate inhibition of trichothecene biosynthesis in Fusarium tricinctum. J. Agric. Food Chem. 42, 2025–2028.
Saremi, H., Burgess, L. W., and Backhouse, D. (1999). Temperature effects on the relative abundance of Fusarium species in a model plant–soil ecosystem. Soil Biol. Biochem. 31, 941–947.
Senatore, M. T., Ward, T. J., Cappelletti, E., Beccari, G., McCormick, S. P., Busman, M., et al. (2021). Species diversity and mycotoxin production by members of the Fusarium tricinctum species complex associated with Fusarium head blight of wheat and barley in Italy. Int. J. Food Microbiol. 358:109298. doi: 10.1016/j.ijfoodmicro.2021.109298
Shang, Q., Yang, G., Wang, Y., Wu, X., Zhao, X., Hao, H., et al. (2016). Illumina-based analysis of the rhizosphere microbial communities associated with healthy and wilted Lanzhou lily (Lilium davidii var. unicolor) plants grown in the field. World J. Microbiol. Biotechnol. 32:95. doi: 10.1007/s11274-016-2051-2
Shang, Q. H., Zhao, X., Li, Y. Y., Xie, Z. K., and Wang, R. Y. (2014). First report of Fusarium tricinctum causing stem and root rot on lanzhou lily (Lilium davidii var. unicolor) in China. Plant Dis. 98:999. doi: 10.1094/PDIS-11-13-1146-PDN
Shi, W., Tan, Y., Wang, S., Gardiner, D. M., De Saeger, S., Liao, Y., et al. (2017). Mycotoxigenic potentials of Fusarium species in various culture matrices revealed by mycotoxin profiling. Toxins 9:6. doi: 10.3390/toxins9010006
Sørensen, J. L., Hansen, F. T., Sondergaard, T. E., Staerk, D., Lee, T. V., Wimmer, R., et al. (2012). Production of novel fusarielins by ectopic activation of the polyketide synthase 9 cluster in Fusarium graminearum. Environ. Microbiol. 14, 1159–1170. doi: 10.1111/j.1462-2920.2011.02696.x
Springer, J. P., Clardy, J., Cole, R. J., Kirksey, J. W., Hill, R. K., Carlson, M. R., et al. (1974). Structure and synthesis of moniliformin, a novel cyclobutane microbial toxin. J. Am. Chem. Soc. 96, 2267–2268. doi: 10.1021/ja00814a055
Stahl, C., Vanderhoef, L. N., Siegel, N., and Helgeson, J. P. (1973). Fusarium tricinctum T-2 toxin inhibits auxin-promoted elongation in soybean hypocotyl. Plant Physiol. 52, 663–666. doi: 10.1104/pp.52.6.663
Thrane, U. (2001). “Developments in the taxonomy of Fusarium species based on secondary metabolites,” in Fusarium Paul E. Nelson Memorial Symposium, eds B. A. Summerell, J. F. Leslie, D. Backhause, W. L. Bryden, and L. W. Burgess (St. Paul: APS Press), 27–49.
Torbati, M., Arzanlou, M., Sandoval-Denis, M., and Crous, P. W. (2019). Multigene phylogeny reveals new fungicolous species in the Fusarium tricinctum species complex and novel hosts in the genus Fusarium from Iran. Mycol. Prog. 18, 119–133.
Turner, A. S., Lees, A. K., Rezanoor, H. N., and Nicholson, P. (1998). Refinement of PCR-detection of Fusarium avenaceum and evidence from DNA marker studies for phenetic relatedness to Fusarium tricinctum. Plant Pathol. 47, 278–288.
Uhlig, S., Jestoi, M., and Parikka, P. (2007). Fusarium avenaceum — The North European situation. Int. J. Food Microbiol. 119, 17–24. doi: 10.1016/j.ijfoodmicro.2007.07.021
Uwaremwe, C., Yue, L., Wang, Y., Tian, Y., Zhao, X., Liu, Y., et al. (2022). An endophytic strain of Bacillus amyloliquefaciens suppresses Fusarium oxysporum infection of chinese wolfberry by altering its rhizosphere bacterial community. Front. Micobiol. 12:782523. doi: 10.3389/fmicb.2021.782523
Visconti, A., Minervini, F., Solfrizzo, M., Bottalico, C., and Lucivero, G. (1992). Toxicity of some Fusarium section Sporotrichiella strains in relation to mycotoxin production. Appl. Environ. Microbiol. 58:769.
Wachowska, U., Kucharska, K., Jędryczka, M., and Lobik, N. (2013). Microorganisms as biological control agents against Fusarium pathogens in winter wheat. Polish J. Environ. Stud. 22, 591–597.
Wagacha, J. M., Oerke, E.-C., Dehne, H.-W., and Steiner, U. (2012). Interactions of Fusarium species during prepenetration development. Fungal Biol. 116, 836–847. doi: 10.1016/j.funbio.2012.05.001
Wätjen, W., Debbab, A., Hohlfeld, A., Chovolou, Y., Kampkötter, A., Edrada, R. A., et al. (2009). Enniatins A1, B and B1 from an endophytic strain of Fusarium tricinctum induce apoptotic cell death in H4IIE hepatoma cells accompanied by inhibition of ERK phosphorylation. Mol. Nutr. Food Res. 53, 431–440. doi: 10.1002/mnfr.200700428
Wegulo, S. N., Bockus, W. W., Nopsa, J. H., De Wolf, E. D., Eskridge, K. M., Peiris, K. H. S., et al. (2010). Effects of integrating cultivar resistance and fungicide application on Fusarium head blight and deoxynivalenol in winter wheat. Plant Dis. 95, 554–560. doi: 10.1094/PDIS-07-10-0495
Wiśniewska, H., Stępień, Ł, Waśkiewicz, A., Beszterda, M., Góral, T., and Belter, J. (2014). Toxigenic Fusarium species infecting wheat heads in Poland. Central Eur. J. Biol. 9, 163–172.
Wu, W., and Vesonder, R. F. (1997). Inhibition of gluconeogenesis in cultured chicken embryo hepatocytes by Fusarium metabolites. Nat. Toxins 5, 80–85. doi: 10.1002/(SICI)(1997)5:2<80::AID-NT5>3.0.CO;2-G
Xu, X. M., Monger, W., Ritieni, A., and Nicholson, P. (2007). Effect of temperature and duration of wetness during initial infection periods on disease development, fungal biomass and mycotoxin concentrations on wheat inoculated with single, or combinations of, Fusarium species. Plant Pathol. 56, 943–956.
Yan, H., and Nelson, B. (2020). Effect of temperature on Fusarium solani and F. tricinctum growth and disease development in soybean. Can. J. Plant Pathol. 42, 527–537.
Keywords: distribution, pathogenicity, mycotoxins, disease control, Fusarium tricinctum
Citation: Wang Y, Wang R and Sha Y (2022) Distribution, pathogenicity and disease control of Fusarium tricinctum. Front. Microbiol. 13:939927. doi: 10.3389/fmicb.2022.939927
Received: 09 May 2022; Accepted: 26 July 2022;
Published: 26 July 2022.
Edited by:
Lukasz Stepien, Institute of Plant Genetics (PAN), PolandReviewed by:
Abdelfattah A. Dababat, International Maize and Wheat Improvement Center, MexicoSameh Selim, UniLaSalle, France
Copyright © 2022 Wang, Wang and Sha. This is an open-access article distributed under the terms of the Creative Commons Attribution License (CC BY). The use, distribution or reproduction in other forums is permitted, provided the original author(s) and the copyright owner(s) are credited and that the original publication in this journal is cited, in accordance with accepted academic practice. No use, distribution or reproduction is permitted which does not comply with these terms.
*Correspondence: Ruoyu Wang, wangruoyu@lzb.ac.cn