- State Key Laboratory of Characteristic Chinese Medicine Resource of Southwest China, School of Pharmacy, Chengdu University of Traditional Chinese Medicine, Chengdu, China
The genus Talaromyces belongs to the phylum Ascomycota of the kingdom Fungi. Studies have shown that Talaromyces species yield many kinds of secondary metabolites, including esters, terpenes, steroids, alkaloids, polyketides, and anthraquinones, some of which have biological activities such as anti-inflammatory, bacteriostatic, and antitumor activities. The chemical constituents of fungi belonging to the genus Talaromyces that have been studied by researchers over the past several years, as well as their biological activities, are reviewed here to provide a reference for the development of high-value natural products and innovative uses of these resources.
Introduction
As new diseases have emerged in recent years in response to environmental changes, the search for new sources to develop effective and safe drugs cannot be delayed. Natural resources offer the potential to find new structural classes with unique bioactivities for disease treatment. Endophytic fungi represent a rich source of bioactive metabolites (Uzma et al., 2018). The genus Talaromyces is widely distributed in soil, plants, sponges, and foods. Recent findings have demonstrated that Talaromyces are very abundant in marine environments (Nicoletti and Vinale, 2018). This may be due to the fact that the ocean itself is rich in species resources. Moreover, the extreme living conditions of the oceans have led marine microorganisms to develop more specific metabolic patterns and Talaromyces can produce a number of structurally diverse active substances. Their metabolites have a wide range of biological activities, such as anti-inflammatory meroterpenoids, thioester-containing benzoate derivatives that exhibit significant α-glucosidase inhibitory activity and oxaphenalenone dimers with broad antibacterial activity. In this paper, we will summarize and describe the research on the secondary metabolites of Talaromyces species and their biological activities over the past several years, to provide a reference for subsequent research on Talaromyces, and to provide an outlook on the problems in the isolation and analysis of fungal secondary metabolites and the prospect of Talaromyces species. The current problems in the isolation and analysis of fungal secondary metabolites are summarized and the prospects of their utilization are provided.
Research status of Talaromyces species
Talaromyces belongs to the fungal phylum, ascomycete subphylum, ascomycetes, sporangia, and fungal family, which are widely distributed in sponges, plants, and soil. The colonies started out yellow and slowly turned gray-green over the course of a week. The middle of the back is yellow, and the edges are white (Figure 1). Talaromyces has various species (Figure 2). T. marneffei, T. funiculosum, and T. purpureogenus are the most studied strains at present. In addition, new strains, such as T. rubrifaciens, T. australis, T. kendrickii, T. veerkampii, T. fuscoviridis, and T. stellenboschiensi were isolated and purified (Visagie et al., 2015; Luo et al., 2016), and the corresponding chemical constituents were studied, which greatly enriched the species of chemical constituents of the fungi. The secondary metabolites of Talaromyces are rich in species, have novel structures and have good biological activity, which provides a basis for the development and application of endophytes. At present, the compounds isolated from the secondary metabolites of Talaromyces include esters, terpenoids and steroids, alkaloids, polyketones, anthraquinones and others, and most of them have good biological activities such as anti-inflammatory, antibacterial and antitumor activities.
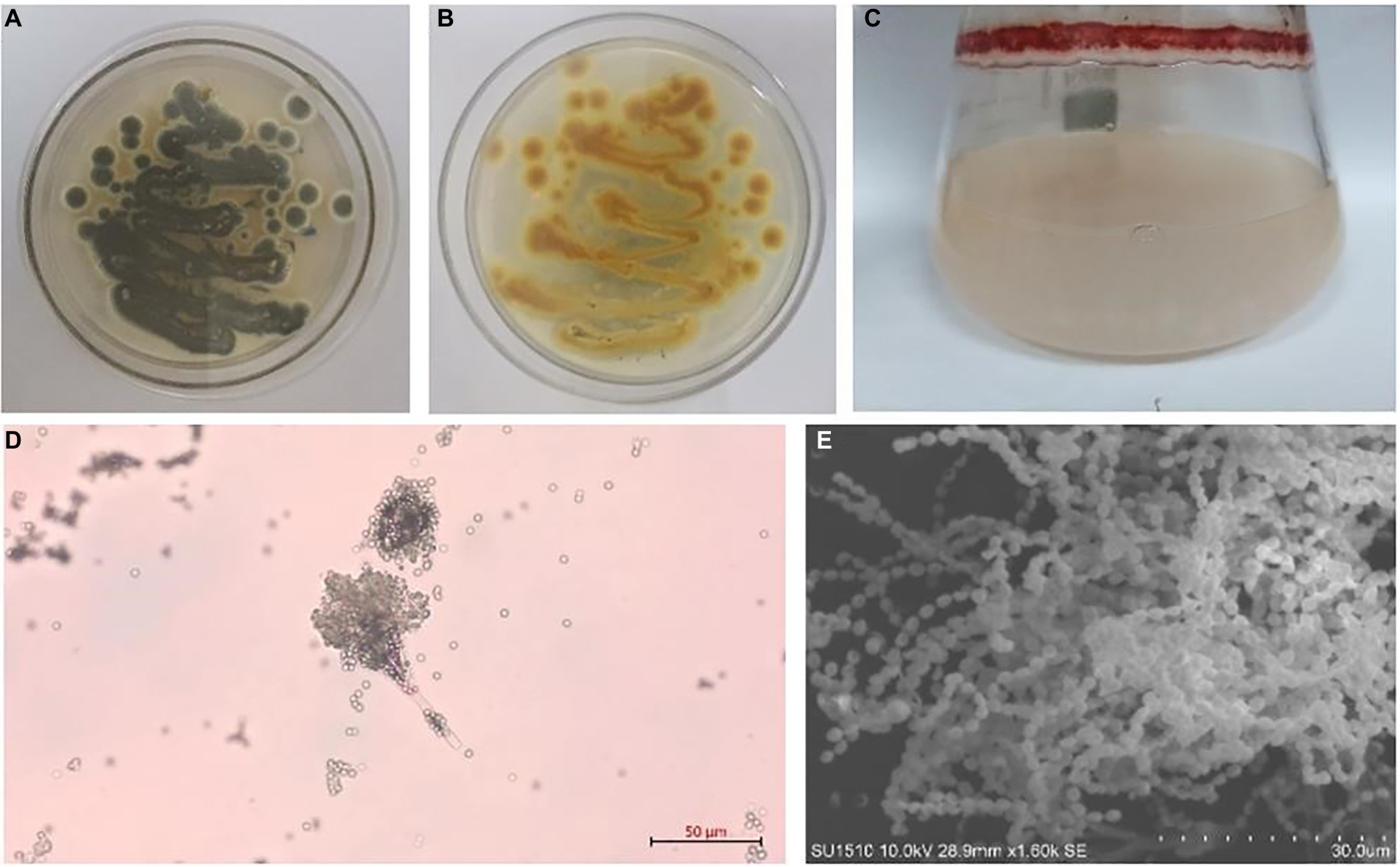
Figure 1. Talaromyces amestolkiae (CBS 365.48) in vitro. (A,B) Growth of T. amestolkiae on M1 semisolid medium at 30°C after 7 d and (C) in liquid M1 medium at 30°C after 7 d; (D) conidia, scale bar = 10 μm; (E) T. amestolkiae, SEM.
Related studies have shown that Talaromyces species have great potential in agriculture, food, cosmetics, medicine, and environmental protection. In the field of agriculture, Talaromyces species can inhibit pathological changes in crops and promote crop growth. T. tratensis can be used as a biological control agent to control brown spot and dirty panicle diseases in rice (Dethoup et al., 2018). The secondary metabolites in T. tratensis, such as glucanase, can effectively treat rot disease that affects the yield of cucumbers and tomatoes (Halo et al., 2019). T. flavus not only promotes the growth of cotton and potatoes (Naraghi et al., 2012) but also produces an enzyme that plays an important role in resisting plant diseases for their strong capacity of degrading chitin (Xian et al., 2011). Most Talaromyces species can produce a red pigment (Frisvad et al., 2013; Venkatachalam et al., 2018), which can be used as a natural colorant in cosmetics and foods. The thermostable enzyme produced in T. emersonii can effectively improve bread quality with respect to hardness, staling, and loaf volume (Waters et al., 2010). An aspartic protease from T. leycettanus has strong proteolytic activity and improves the clarity of fruit juice (Guo et al., 2019). Talaromyces species can produce many other bioactive secondary metabolites, and these compounds have been found to have antibacterial, anti-inflammatory, antitumor, antioxidant, nematocidal, and other effects in medical research. Secondary metabolites from an Australian Marine Tunicate-Associated Fungus Talaromyces sp. (CMB-TU011) exhibit certain antibacterial activities (Dewapriya et al., 2018). GH3 β-glucosidases from T. amestolkiae expressed in Pichia pastoris can transglycosylate phenolic molecules, and the resulting transglycosylation products can improve the biological activity of the original aglycones against breast cancer cells (Méndez-Líter et al., 2019). Talaraculones from a strain of T. aculeatus can inhibit the activity of α-glucosidase and can be used to prevent the progression of type II diabetes, as well as for the early treatment of type II diabetes (Ren et al., 2017). In the field of environmental protection, biosorption by microorganisms has been proven to be an effective technique for removing heavy metals from wastewater. A biological adsorbent formed by combining T. amestolkiae with a specific chitosan sponge can effectively remove trace heavy metals or high concentrations of lead from industrial wastewater (Wang et al., 2019). Talaromyces sp. KM-31 can remove arsenic from heavily polluted wastewater and can thus be employed in bioremediation strategies (Nam et al., 2019).
According to the classification of the chemical components, this paper will summarize and explain research carried out on secondary metabolites from Talaromyces species and their biological activities over the past 10 years with the aim of providing references for follow-up studies of Talaromyces, at the same time, the problems existing in the separation and analysis of fungal secondary metabolites and the prospect of Talaromyces species, as well as summarizing existing problems in the separation and analysis of fungal secondary metabolites and prospects for the use of secondary metabolites from Talaromyces species.
Studies on the chemical constituents and activity of Talaromyces
Ester-based compounds
Esters
Esters are chemical compounds derived by reacting an oxoacid with a hydroxyl compound such as an alcohol or phenol (Sparkman et al., 2011). Dinapinones AB1 and AB2 (1 and 2), dinapinones AC1 and AC2 (3 and 4), dinapinones AD1 and AD2 (5 and 6), and dinapinones AE1 and AE2 (7 and 8; Figure 3), which were isolated from the fermentation broth of T. pinophilus FKI-3864 in 2013 (Kawaguchi et al., 2013), were identified and characterized as ester derivatives. These dinaphthoquinones have the same backbone of aryl dihydronaphthoquinone and consist of one monapinone A and one different monapinone in a heterodimer. Compound 2 had a strong inhibitory effect on triacylglycerol synthesis in intact mammalian cells, with an IC50 value of 1.17 μM.
Seventeen new polyesters were isolated from the fermentation products of the wetland soil-derived fungus T. flavus, namely, talapolyesters A–F (9–12, 22 and 24), 15G256ν (13), 15G256ν-me (14), 15G256π (15), 15G256β-2 (16), 15G256α-2 (17), 15G256α-2-me (18), 15G256ι (19), 15G256β (20), 15G256α (21), 15G256α-1 (23) (Figure 4), and 15G256ω (25) (He et al., 2014b). All macrocyclic polyesters (19–25) were cytotoxic to HL-60, SMMC-7721, A-549, MCF-7, and SW480 tumor cells, while linear polyesters (9–18) were inactive with IC50 > 40 mM compared to cisplatin. This suggests that a macrocyclic structure is required for cytotoxicity. Among them, 20 and 25 showed significant cytotoxic activity against MCF-7 cell lines with IC50 of 3.27 and 4.32 μM, respectively. The cytotoxic activity of 15G256 polyester was systematically investigated for the first time and a tight conformational relationship is presented.
Talaromycolides A–C (26–28), rubralide C (29), sclerotinin A (30), alternariol (31), and penicillide (32) were obtained from the epiphytic fungal strain T. pinophilus AF-02, which was isolated from green Chinese onion, in 2015 (Zhai et al., 2015). Compound 26 [minimum inhibitory concentration (MIC) = 12.5 μg/ml] showed stronger inhibitory activity against Clostridium perfringens than erythromycin, streptomycin, acheomycin, and ampicillin. Compound 26 (MIC = 6.25 μg/ml) showed similar inhibitory activity to acheomycin and was superior to levofloxacin, ampicillin, and streptomycin against Bacillus subtilis. Compound 27 (MIC = 12.5 μg/ml) showed higher inhibitory activity than erythromycin and ampicillin against Bacillus megaterium and higher inhibitory activity than erythromycin, ampicillin, and streptomycin against Escherichia coli (MIC = 25 μg/ml). Compound 28 (MIC = 25 μg/ml) was more active against C. perfringens than erythromycin, streptomycin, acheomycin and ampicillin.
In 2015, the structures of compounds 33 and 34 were characterized as deacetylisowortmins A and B, which were isolated from T. wortmannii LGT-4 derived from the leaves of a mangrove plant Acanthus ilicifolius (Fu et al., 2016). Four esters, talaromyones A and B (35 and 36), penicillide (32), and purpactin A (37), were obtained from a fermentation product of the mangrove endophytic fungus T. stipitatus SK-4 in 2016 (Cai et al., 2017). Compound 36 exhibited antibacterial activity against B. subtilis with an MIC value of 12.5 μg/ml. In the α-glucosidase inhibition assay, compounds 36 and 37 showed some inhibitory activity with an IC50 values of 48.4–99.8 μM.
Five butenolides (38–42), seven (3S)-resorcylide derivatives (43–49) (Figure 5), two butenolide-resorcylide dimers (50 and 51) were yielded by culture on a solid rice medium of T. rugulosus isolated from the Mediterranean sponge Axinella cannabina (Küppers et al., 2017). The butenolide–resorcylide dimers talarodilactones A and B (50 and 51) was highly cytotoxic to the L5178Y mouse lymphoma cell line with IC50 of 3.9 μM and 1.3 μM, respectively.
Talaromycin A (52) and clearanol A (53) were isolated from the endophytic fungus Talaromyces sp. MH551540 associated with Xanthoparmelia angustiphylla in 2018 (Yuan et al., 2018). Compound 52 and 53 had selective cytotoxicity against MDA-MB-231 cells. Compound 54, which was identified as wortmannine F, was obtained from cultures of the endophytic fungus T. wortmannii LGT-4 isolated from Tripterygium wilfordii and has a strong phosphoinositide-3-kinase-α (PI3K-α) inhibitory activity with an IC50 value of 25 μM (Zhao et al., 2019b). Pentalsamonin (55) was isolated from submerged fermentation on Bengal gram husk (BegH) of T. purpureogenus CFRM-02 (Pandit et al., 2018). The MIC and MBC of pentalsamonin (55) against B. subtilis, Staphylococcus aureus, E. coli, and Klebsiella pneumoniae were 62.5–125 and 125–250 μg/ml, respectively.
Talaromarnine A (56) and talaromarnine B (57) were obtained from cultures of T. marneffei, an endophytic fungus of Epilobium angustifolium (Yang et al., 2021). Two previously undescribed phthalides, amestolkins A (58) and B (59) were isolated from T. amestolkiae derived from Syngnathus acus Linnaeus in Lingshui Li Autonomous County, Hainan Province, China, which has the same planar structure of (1,5-dihydroxyhexyl)-7-hydroxyisobenzofuran-1(3H)-one. They were shown to inhibit gene expressions of proinflammatory factors including C-C motif chemokine ligand 2 (CCL-2), tumor necrosis factor-α (TNF-α), and interleukin-6 (IL-6) as well as reducing the secretion of inducible nitric oxide synthase (iNOS) in BV2 microglia at the concentration of 30 μM (Huang et al., 2022).
Coumarins
Coumarinic compounds are lactones resulting from the fusion of a benzene ring and a α-pyrone ring (Batista et al., 2021). Talacoumarins A and B (60 and 61), which were characterized as coumarins, were isolated from the fermentation broth of the wetland soil fungus T. flavus (He et al., 2014c). Activity tests showed that compounds 60 and 61 exhibited moderate activity against the aggregation of Aβ42. This was the first report to state that a coumarin can inhibit Aβ42 aggregation. A new compound 62, chloropestalasin A was isolated from T. amestolkiae derived from submerged wood collected from fresh water, along with 3-hydroxymethyl-6,8-dimethoxycoumarin (63) and pestalasin A (64) (El-Elimat et al., 2021).
Isocoumarin
Isocoumarin is the common name for 1H-2-benzopyran-1-one skeleton (Braca et al., 2012). Three dihydroisocoumarins (65–67) were yielded by culture on a solid rice medium of T. rugulosus isolated from the Mediterranean sponge Axinella cannabina (Küppers et al., 2017). Six new isocoumarin derivatives, talaromarins A-F (68–73), and 17 known analogues (67, 74–89), were isolated from the mangrove-derived fungus T. flavus (Eurotiales: Trichocomaceae) TGGP35 (Cai et al., 2022). Compounds 67, 73–78, 84–85 and 87–89 showed similar or better IC50 values for antioxidant activity ranged from 0.009 mM to 0.27 mM, compared to the positive control trolox (IC50 = 0.29 mM). Compounds 77, 84, 87 and 89 showed strong inhibitory activity. IC50 values of 0.10 ~ 0.62 mM against α-glucosidase and 0.5 mM for the positive control acarbose activity at 50 μg/ml and 1 mg/ml concentrations. These results suggest that isocoumarins have important applications in the development of antioxidants and in the control of diabetes mellitus. Talaroisocoumarin A (73) was obtained from marine-derived Talaromyces sp. ZZ1616 in potato dextrose broth medium. The MIC values of talaroisocoumarin A against methicillin-resistant S. aureus, E. coli and Candida albicans were 36.0 μg/ml, 32.0 μg/ml and 26.0 μg/ml, respectively (Ma et al., 2022).
Polyketones
Polyketides were named in the 1890s to refer to a structurally diverse group of natural products that contained many carbonyls and alcohols, generally separated by methylene carbons. They are synthesized by a series of decarboxylative condensation reactions between small carboxylic acids and malonate using polyketide synthases (PKSs; Richardson and Khosla, 1999). Two polyketones, mitorubrin (90) and monascorubrin (91) (Figure 6), were isolated from T. atroroseus (Frisvad et al., 2013). Because no citrinin was found in any Talaromyces species, it may be a good alternative for red pigment production. Compound 92, which was characterized as a polyketone and named talaroxanthone, was obtained from the fermentation products of an endophytic strain of a Talaromyces sp. isolated from the Amazonian rainforest plant Duguetia stelechantha root (Koolen et al., 2013). Five compounds, 9a-epi-bacillisporin E (93), 1-epi-bacillisporin F (94), and bacillisporins F-H (95–97) were isolated from the fermentation products of the soil fungus T. stipitatus (Zang et al., 2016). Compound 97 exhibited some antibacterial activity and some cytotoxicity against HeLa cells. Compounds 98–100, wortmannilactones I1-I3, which were identified and characterized as three new polyketides, were purified from T. wortmannii using the one strain–many compounds strategy. These compounds showed selective inhibitory activity against NADH fumarate reductase (Liu et al., 2016).
The polyketone 3-O-methylfunicone (101) was isolated from the culture filtrate of an endophytic strain of T. pinophilus obtained from the strawberry tree (Arbutus unedo) in 2017 (Vinale et al., 2017). On water agar at a concentration of 0.1 mg/ml, it completely inhibited the growth of phytopathogenic fungi such as Rhizoctonia solani (De Stefano et al., 1999). Eleven polyketones, talaraculones A–F (102–107), pinazaphilone B (108), pinophilin B (109), Sch 725680 (110), (−)-mitorubrin (111), and (−)-mitorubrinol (112), were obtained from the fungus T. aculeatus, which was isolated from saline-alkali soil (Ren et al., 2017). The results of the activity tests showed that compounds 102 and 103 exhibited very high levels of inhibitory activity against α-glucosidase than the positive control acarbose (IC50 = 101.5 μM), with IC50 values of 78.6 and 22.9 μM, respectively. Compounds that were defined and characterized as six polyketones, paecillin D (113), secalonic acid A (114), blennolide G (115), versixanthone A (116) (Figure 7), penicillixanthone A (117), and paecillin B (118), were isolated from the fermentation products of three Amazonian plants endophytic strains of T. stipitatus in 2018 (da Silva et al., 2017). Activity tests showed that compounds 113 and 116 were active against yeasts (MICs of 15.6 μg/ml and 31.3 μg/ml, respectively).
Six new nonadride derivatives, named talarodrides A–F (119–124), were isolated from the antarctic sponge-derived fungus Talaromyces sp. HDN1820200. Talarodride A (119) and talarodride B (120) showed selective inhibitory effects against Proteus mirabilis and Vibrio parahemolyticus with MICs of 3.13–12.5 μM (Zhao et al., 2021b).
Anthraquinone
Anthraquinones (AQs) are derived from anthracenes and have two keto groups, mostly in positions 9 and 10. The basal compound, anthraquinone (9,10-dioxoanthracene), can be substituted in various ways, resulting in a great diversity of structures (Vasil et al., 1984). Two anthraquinone compounds skyrin (125) and emodin (126) (Figure 8) were obtained from an extract of the mangrove endophytic fungus Talaromyces sp. ZH-154, which was isolated from the stem bark of Kandelia candel (Liu et al., 2010). Both compounds exhibited moderate cytotoxic activity against KB and KBv200 cells. The anthraquinone monomer (126) showed higher bioactivity than the dimer dianthraquinone (125). A new anthraquinones biemodin (127) and five known anthraquinones emodic acid (128), skyrin (125), oxyskyrin (129), and rugulosins A and B (130 and 131) were isolated from cultures of the endophytic fungus T. wortmannii obtained from healthy inner tissues of Aloe vera (Bara et al., 2013a). In the same year, two anthraquinone compounds, talaromannins A and B (132 and 133), were obtained from T. wortmannii in A. vera (Bara et al., 2013b). Both compounds displayed moderate MICs in a comparable concentration range for S. aureus and 132 represented the most active congeners.
Five anthraquinones were isolated from the solid fermentation products of the endophytic fungus Talaromyces sp. YE3016 (Xie et al., 2016). These compounds were 3-demethyl-3-(2-hydroxypropyl)-skyrin (134), skyrin (125), oxyskyrin (129), emodin (126), and 1,3,6-trihydroxy-8-methylanthraquinone (135). Activity tests showed that compounds 134, 125, and 129 displayed moderate cytotoxic activity against the MCF-7 cell line. Six anthraquinone compounds, 2,2′-bis-(7-methyl-1,4,5-trihydroxy-anthracene-9,10-dione) (136), emodin (126), questinol (137), citreorosein (138), fallacinol (139), and rheoemodin (140), were obtained from an ethyl acetate extract of a culture of the fungus T. stipitatus KUFA 0207, which is derived with a marine sponge (Noinart et al., 2017). Emodin (126), questinol (137), citreorosein (138), fallacinol (139), and rheoemodin (140) were tested for their anti-obesity activity using the zebrafish Nile red assay. The results showed that only the anthraquinones questinol (137) and citreorosein (138) had significant anti-obesity activity. Questinol (137) and citreorosein (138) reduced >60% and > 90% of the stained lipids with the IC50 values of 0.95 and 0.17 μM, respectively. The positive control resveratrol (REV) had an IC50 value of 0.6 μM. Emodin (140) caused toxicity (death) for all exposed zebrafish larvae after 24 h, while fallacinol (139) and rheoemodin (140) did not have any significant effects. It is interesting to observe that questinol (137), citreorosein (138) and fallacinol (139) are structurally similar, all having a hydroxymethyl group on C-6 and a hydroxyl group on C-8. Replacing the hydroxyl group on C-1 by a methoxyl group, as in questinol (137), diminishes the activity whereas replacing the hydroxyl group on C-3 with a methoxyl group, as in fallacinol (139), completely removes the anti-obesity activity. Therefore, it seems that the hydroxymethyl group on C-6 and the hydroxyl groups on C-3 and C-8 are necessary for the anti-obesity activity of the polyhydroxy anthraquinones.
Terpenoids
Terpenoids otherwise known as isoprenoids are a large and diverse class of naturally occurring compounds derived from five carbon isoprene units (Reyes et al., 2018). Terpenoids are classified as hemiterpenes (C5), monoterpenes (C10), sesquiterpenes (C15), diterpenes (C20), sesterterpenes (C25), triterpenes (C30), and tetraterpenes/carotenoids (C40) (Adefegha et al., 2022). Compound 141 (Figure 9), which was characterized as a new fusicoccane diterpene and named pinophicin A, was obtained from the endophytic fungus T. pinophilus collected from the aerial parts of Salvia miltiorrhiza in 2019 (Zhao et al., 2021a). Four new sesquiterpene peroxides, talaperoxides A–D (142–145), were isolated from the fermentation products of the mangrove endophytic fungus T. flavus (Li et al., 2011). Of these compounds, compounds 143 and 145 showed cytotoxicity against human cancer cell lines MCF-7 and MDA-MB-435, HepG2, HeLa and PC-3 with IC50 values between 0.70 and 2.78 μg/ml. Compound 146, which was characterized as a new nardosinane-type sesquiterpene and named talaflavuterpenoid A, was isolated from the fermentation products of T. flavus (He et al., 2014a).
The new diterpenoid roussoellol C (147) was isolated from the fermentation products of T. purpureogenus (Wang et al., 2018). Compound 147 had an inhibitory effect on the MCF-7 cancer cell line, with an IC50 value of 6.5 μM. A new spiroaxane sesquiterpenoid talaminoid A (148) and two drimane sesquiterpenoid talaminoids B and C (149 and 150), together with four known compounds (151–154) were obtained from the fermentation broth of T. minioluteus (Nie et al., 2019). Compounds 148, 151, and 152 showed significant suppressive effect on the production of NO on LPS-induced BV-2 cells, with IC50 values ranging from 4.97 to 7.81 μM. In addition, 148, 151, and 152 exhibited significant anti-inflammatory activities against the production of TNF-α and IL-6. Further immunofluorescence experiments revealed the mechanism of action to be inhibitory the NF-kB-activated pathway. The structure of compound 155 was defined and characterized as sordarin, which was isolated from the Australian fungus Talaromyces sp. CMB-TU011, which is associated with a marine tunicate (Dewapriya et al., 2017). According to a related study, this compound exhibited antifungal activity (Domínguez et al., 1998). Four new sesquiterpene lactones (156–159) and three known compounds, purpuride (151), berkedrimane B (152) and purpuride B (160), were isolated from cultures of the marine fungus T. minioluteus (Ngokpol et al., 2015). Compounds 152, 156, 159 exhibited weak cytotoxic activity against the HepG2 cancer cell line.
Meroterpenoids
Meroterpenoids are natural products that are partially derived from terpenoid biosynthetic pathways, since the prefix “mero-” has the meanings of “part,” “partial,” and “fragment” (Matsuda and Abe, 2020). Four meroterpenoids talaromyolides A–D (161–164) and Talaromytin (165) (Figure 10) were isolated from the marine fungus Talaromyces sp. CX11 (Nie et al., 2019). Compound 164 exhibited potent antiviral activity against pseudorabies virus (PRV) with a IC50 value of 3.35 μM. Activity tests showed that this compound did not exhibit in vitro growth-inhibiting activity against MCF-7 breast adenocarcinoma, NCI-H460 non-small-cell lung cancer, or A375-C5 melanoma cell lines by a method based on the protein-binding dye sulforhodamine B.
A new meroterpenoid, taladrimanin A (166), was isolated from the marine-derived fungus Talaromyces sp. HM6-1-1. Compound 166 exhibited antitumor activity against MGC803 and MKN28 gastric cancer cells; it also inhibited colony formation and induced apoptosis in MGC803 cells both in a concentration-dependent manner. Additionally, 166 displayed selective antibacterial activity against S. aureus 6538P, and low activities toward strains of V. parahaemolyticus and E. coli (Hong et al., 2022). The structures of compounds 167–173, which were obtained from the fermentation products of the soil fungus Talaromyces sp. YO-2 in Osaka, Japan, were defined and characterized as the seven meroterpenoids chrodrimanin A–H (Hayashi et al., 2012a,b). Chrodrimanin B (168) exhibited insecticidal activity with an LD50 value of 10 ug/g of diet. Chrodrimanins D–F (170–172) showed insecticidal activity against silkworms with respective LD50 values of 20, 10, and 50 ug/g of diet. Compounds 145–148, which were identified as the four meroterpenoid compounds talarolutin A–D, were isolated from the fermentation broth of a strain of the fungus T. minioluteus obtained from healthy surface sterilized leaves of milk thistle (Kaur et al., 2016).
Steroids
Steroids are extremely important medicinally active organic compounds with four rings constructed in a highly specific perhydrocyclopentano[α]phenanthrene orientation. In general, the steroid core structure has 17 carbon atoms connected with 4 fused rings in a specific way. Three of these are cyclohexanes (A, B, and C) and one is cyclopentane system (D ring) (Borah and Banik, 2020). Talasterone A (174) (Figure 11), an unprecedented 6/6/5 tricyclic 13 (14 → 8) abeo-8,14-seco-ergostane steroid, was characterized from T. adpressus isolated from soil collected from Yalong Bay in Sanya, Hainan (Zhang et al., 2022a). A new compound 3-acetylergosterol-5,8-endoperoxide (175) was obtained from the fermentation products of the sponge endophytic fungus T. trachyspermus KUFA 0021 (Kuml et al., 2014). In 2017, the new compound talarosterone (176) and cyathisterone (177) were obtained from the fermentation products of the sponge fungus T. stipitatus KUFA 0207 (Noinart et al., 2017). A new withanolide, talasteroid (178) was obtained from rice culture of the marine-derived fungus T. stollii HBU-115 (Zhang et al., 2022c). Five undescribed sterol derivatives (179–183), (22E,24R)-7α-methoxy-5α,6α-epoxyergosta-8(14),22-diene-3β,15β-diol, (22E,24R)-5α,6α-epoxyergosta-8(14),22-diene-3β,7β,15α-triol, (22E,24R)-3β,5α-dihydroxy-14β,15β-epoxyergosta-7,22-diene-6-one, (22E,24R)-6α-methoxy-7α,15β-dihydroxyergosta-4,8(14),22-triene-3-one, and (25S)-ergosta-7,24(28)-diene-3β,4α,6α,26-tetraol were isolated from the extract of T. stipitatus (Zhang et al., 2021). The antiproliferative activities of compound 179–183 were mainly mediated by inducing cell apoptosis.
Nitrogen-containing compound
Alkaloids
Alkaloids are structurally diverse compounds generally classified as such due to the basic character of the molecule (from Latin alkali) and a presence of at least one nitrogen atom, preferably in a heterocycle (Zotchev, 2013). The compound PP-R (184) (Figure 12) was isolated from T. atroroseus (Frisvad et al., 2013). The red pigments is of interest for the industry as they are stable and non-toxic and can be used as food colorants. Herquline B (185) was isolated from the culture filtrate of an endophytic strain of T. pinophilus obtained from the strawberry tree (A. unedo) (Vinale et al., 2017). In 2011, six indole alkaloids, talathermophilins A–E (186–188,190–191) and cyclo(glycyltryptophyl) (189), were obtained from the thermophilic fungal strain T. thermophilus YM3-4 (Guo et al., 2011, 3–4). ZG-1494α (192) was isolated from an ethyl acetate extract of a culture broth of T. atroroseus (Frisvad et al., 2013). According to a related study, compound 192 can be used as a novel inhibitor of platelet-activating factor acetyl-transferase (West et al., 1996). Nine alkaloids, 2-[(S)-hydroxy(phenyl)methyl]-3-methylquinazolin-4(3H)-one (193), 2-[(R)-hydroxy(phenyl)methyl]-3-methylquinazolin-4(3H)-one (194), roquefortine C (195), Z-roquefortine C (196), viridicatol (197), penitrem A (198), penijanthine A (199), paspaline (200), and 3-deoxo-4b-deoxypaxilline (201), were isolated from the fermentation broth of the algal endophytic fungus Talaromyces sp. cf-16 in 2014, of which compounds 196–199 could inhibit S. aureus (Yang et al., 2016).
Five new compounds, namely talaromanloid A (202), 10-hydroxy-8-demethyltalaromydine and 11-hydroxy-8-demethyltalaromydine (203 and 204) and ditalaromylectones A and B (205 and 206) were identified from the marine-derived fungus T. mangshanicus BTBU20211089, which was isolated from a sediment sample collected from the South China Sea. Compound 205 showed an inhibitory effect against C. albicans with an MIC value of 200 μg/ml (Zhang et al., 2022b). The endophytic fungus T. radicus isolated from Catharanthus roseus was cultured in M2 liquid fermentation medium and PDA fermentation medium. Vincristine (207) and vinblastine (208) were obtained from this fungus, of which HeLa cells exhibited the highest susceptibility to vincristine. In addition, the apoptosis-inducing activity of vincristine obtained from this fungus was established via cell cycle analysis, loss of mitochondrial membrane potential, and DNA fragmentation patterns (Palem et al., 2015). In 2017, the alkaloid talaramide A (209) was obtained by culturing of the mangrove endophytic fungus Talaromyces sp. HZ-YX1 on a solid rice medium with sea water displayed promising inhibition of the activity of mycobacterial protein kinase G, with an IC50 value of 55 μM. A possible biosynthetic pathway was proposed in the paper (Chen et al., 2017).
Amides
Amides are amines with a carbonyl group associated with the ammonia-associated carbon (Jackson, 2008). Six macrolides, thermolides A–F (210–215) (Figure 13), were isolated from the fermentation products of the thermophilic fungus T. thermophilus in 2012 (Guo et al., 2012). Of these compounds, compounds 210 and 211 exhibited strong inhibitory activity against nematodes, with LC50 values of 0.5–1.0 μg/ml. Two new compounds, namely talaromydene (216) and talaromylectone (217) were identified from the marine-derived fungus T. mangshanicus BTBU20211089, which was isolated from a sediment sample collected from the South China Sea (Zhang et al., 2022b). Cerebroside C (218) was obtained from the endophytic fungus T. purpureogenus hosted in Tylophora ovate (Zhao et al., 2020).
Acid
A compound, namely, (R)-2-[5-methoxycarbonyl-4-methyl-6-oxo-3,6-dihydro-2H-pyran-2-yl] acetic acid (61), which was obtained from cultures of the endophytic fungus T. purpureogenus hosted in T. ovate, showed some inhibitory activity against XOD at a concentration of 10 μM with the inhibition rate of 69.9% (Zhao et al., 2020). A new octadienoic acid derivative, oxoberkedienoic acid (219) (Figure 14), was isolated from a culture of T. verruculosus FKI-5393. The IC50 value against Jurkat cells of 219 was 6.1 μg/ml (Sakai et al., 2018). The IC50 value against Jurkat cells of 219 was 6.1 mg/ml. (R)-(−)-Hydroxysydonic acid (220) was isolated from the strain Talaromyces sp. C21-1 obtained from the coral Porites pukoensis collected in Xuwen, Guangdong Province (Nie et al., 2019). The compound 220 showed moderate inhibitory activities to C. albicans and methicillin-resistant S. aureus (MRSA) with the MICs at 0.075 mM and 0.2 mM, respectively. Rubratoxin acid A-E (221–225) were isolated from the endophytic fungus T. purpureogenus obtained from fresh leaves of the toxic medicinal plant T. ovate (Zhao et al., 2019a). Compound 221 showed significant inhibitory activity against NO production in LPS-induced RAW264.7 cells with an IC50 value of 1.9 μM. Compounds 222 showed moderate inhibitory activities toward XOD and PTP1b at 10 μM with inhibition rates of 67%. Compound 226, which was identified as a new spiculisporic acid derivative, spic ulisporic acid E, was isolated from a culture of the fungus T. trachyspermus KUFA 0021, which is associated with a marine sponge (Kuml et al., 2014).
Others
The compounds 2,2',3,5'-tetrahydroxy-3'-methylbenzophenone (227) and 2,2',5'-trihydroxy-3-methoxy-3'-methylbenzophenone (228) (Figure 15), were obtained from T. islandicus EN-501, which is an endophytic fungus obtained from the freshly collected marine red alga Laurencia okamurai (Li et al., 2016). Compounds 227–228 showed strong antioxidant activity against DPPH and ABTS radicals with IC50 values of 0.58 ~ 6.92 μg/ml, which were stronger than the positive controls BHT and ascorbic acid. Compounds 227 displayed potent activities against three human pathogens (E. coli, Pseudomonas aeruginosa, and S. aureus) and three aquatic bacteria (V. alginolyticus, V. harveyi, and V. parahaemolyticus) with MIC values ranging from 4 to 32 μg/ml. compound 228 showed weak activity against the tested bacteria (IC50 > 64 μg/ml), suggesting that methoxylation at C-3 weakened the antibacterial activities. A new phenylpentenol, wortmannine H (229), was isolated from T. wortmannii LGT-4, which is an endophytic fungus obtained from T. wilfordii (Li et al., 2021).
Talarodride (230) were isolated from the endophytic fungus T. purpureogenus obtained from fresh leaves of the toxic medicinal plant T. ovate (Zhao et al., 2019a). Compounds 230 showed moderate inhibitory activities toward XOD and PTP1b, respectively at 10 μM with inhibition rates of 76%. Four wortmannin derivative compounds, wortmannin B (231), wortmannin (232), amino adduct 3a (233), and wortmannin-diol (VIII) (234), were obtained from cultures of the aloe endophytic fungus T. wortmannii in 2013 (Bara et al., 2013a) Three new diphenyl ether derivatives, talaromycins A–C (235–237), together with a known analog (238), were obtained from a gorgonian-derived Talaromyces sp. (Chen et al., 2015). Compounds 237 showed potent antifouling activities against the larval settlement of the barnacle Balanus amphitrite with the EC50 values ranging from 2.2 to 4.8 mg/ml. Compounds 238 showed strong cytotoxicity against the human hepatoma HepG2 and Hep3B, human breast cancer MCF-7/ADR, human prostatic cancer PC-3, and human colon carcinoma HCT-116 cell lines with the IC50 values ranging from 4.3 to 9.8 mM.
Summary
Owing to their wide variety of species and abundance in secondary metabolites, Talaromyces fungi have great potential in medicine, food, cosmetics, agriculture, and environmental protection. In this paper, the secondary metabolites produced by Talaromyces species that have been studied over the past several years are classified and summarized according to the types of compounds (Table 1). Secondary metabolites from more than ten Talaromyces species, including T. wortmannii, T. pinophilus, T. flavus, T. stipitatus, T. purpureogenus, and T. minioluteus, have been covered in this paper. These metabolites included 89 esters, 35 polyketones, 16 anthraquinone, 20 terpenoids, 13 meroterpenoids, 10 steroids, 35 nitrogen compounds, 8 acids, and 12 other compounds. Most of these compounds have useful biological activities, such as anti-inflammatory, antibacterial, antitumor, hypolipidemic, or nematocidal activities or inhibition of α-glucosidase, xanthine oxidase, acetyltransferase, NADH fumarate reductase, PI3K-α, Aβ42 aggregation, or the production of NO induced by lipopolysaccharide.

Table 1. Name of Talaromyces’ secondary metabolites, source strains, activity and their culture media.
Prospects
Talaromyces fungi include some of the most important species of microorganisms. The secondary metabolites from Talaromyces species that have unique structures and useful activities are of great value in research and development. However, there are still some problems to be solved in the study of fungal secondary metabolites. Firstly, owing to the limitations of strain isolation techniques and fungal culture conditions, some fungi cannot be isolated or do not grow well. Now often use fungal culture mediums are: PDA medium, PDB medium, BegH medium, rice solid medium and so on. Among them, rice medium is the most used (Table 1), which may be due to more fungal metabolites cultured in solid medium than liquid medium. It also reflected the problems of single nutrition and limited culture in the application of fungus synthesis medium. It is hoped that unconventional media can be used and new media can be developed. Secondly, it had been reported in available reports that the addition of epigenetic modifications to the culture medium can stimulate the expression of silenced genes thereby enabling the production of novel secondary metabolites. However, none of the literature in the study of secondary metabolites of the Talaromyces has investigated the effect of epigenetic modifiers. Therefore, epigenetic modifiers can be added to stimulate the expression of their silent genes. Finally, many existing studies have been done on the ethyl acetate part of the ferment, which is moderately polar and easy to separate. The aqueous part, on the other hand, has been ignored or even discarded due to its high polarity and difficulty of separation. Therefore, it is hoped that methods for the separation of compounds with high polarity will be developed as well as the development of related fillers. In a word, we should make full use of modern scientific and technological methods to carry out an in-depth study of the secondary metabolites produced by Talaromyces fungi and identify new active components to provide lead compounds for the research and development of innovative drugs.
Author contributions
L-RL, L-QG, and M-YJ wrote the paper. JG, RW, and RL cultured and identified the fungus. L-RL, M-DL, and LH collected the STM data. YD checked the paper. G-ZW and DW verified the content. All authors have read and agreed to the published version of the manuscript.
Funding
This research was funded by the National Natural Science Foundation of China (81973189, 81973460), Science and Technology Department of Sichuan Province (2021ZYD0079), Chengdu University of Traditional Chinese Medicine (CZYJC1905, 2020XSGG016, 2020JCRC006, SKL2021-19, SKL2021-42), and National Interdisciplinary Innovation Team of Traditional Chinese Medicine (ZYYCXTD-D-202209).
Conflict of interest
The authors declare that the research was conducted in the absence of any commercial or financial relationships that could be construed as a potential conflict of interest.
Publisher’s note
All claims expressed in this article are solely those of the authors and do not necessarily represent those of their affiliated organizations, or those of the publisher, the editors and the reviewers. Any product that may be evaluated in this article, or claim that may be made by its manufacturer, is not guaranteed or endorsed by the publisher.
References
Adefegha, S. A., Oboh, G., and Oluokun, O. O. (2022). Chapter 11 – Food bioactives: the food image behind the curtain of health promotion and prevention against several degenerative diseases. Stud. Nat. Prod. Chem. 72, 391–421. doi: 10.1016/B978-0-12-823944-5.00012-0
Bara, R., Aly, A. H., Pretsch, A., Wray, V., Wang, B., Proksch, P., et al. (2013a). Antibiotically active metabolites from Talaromyces wortmannii, an endophyte of Aloe vera. J. Antibiot. 66, 491–493. doi: 10.1038/ja.2013.28
Bara, R., Zerfass, I., Aly, A. H., Goldbach-Gecke, H., Raghavan, V., Sass, P., et al. (2013b). Atropisomeric dihydroanthracenones as inhibitors of multiresistant Staphylococcus aureus. J. Med. Chem. 56, 3257–3272. doi: 10.1021/jm301816a
Batista, Â. G., Silva-Maia Da, J. K., and Maróstica, M. R. (2021). “Generation and alterations of bioactive organosulfur and phenolic compounds,” in Chemical Changes during Processing and Storage of Foods. eds. D. B. Rodriguez-Amaya and J. Amaya-Farfan (London, UK: Elsevier). 537–577.
Borah, P., and Banik, B. K. (2020). “12 – Diverse synthesis of medicinally active steroids,” in Green Approaches in Medicinal Chemistry for Sustainable Drug Design Advances in Green and Sustainable Chemistry. ed. B. K. Banik (London, UK: Elsevier), 449–490.
Braca, A., Bader, A., and De Tommasi, N. (2012). Plant and fungi 3,4-dihydroisocoumarins. Stud. Nat. Prod. Chem 37, 191–215. doi: 10.1016/B978-0-444-59514-0.00007-9
Cai, R., Chen, S., Long, Y., Li, C., Huang, X., and She, Z. (2017). Depsidones from Talaromyces stipitatus SK-4, an endophytic fungus of the mangrove plant Acanthus ilicifolius. Phytochem. Lett. 20, 196–199. doi: 10.1016/j.phytol.2017.04.023
Cai, J., Zhu, X. C., Zeng, W. N., Wang, B., Luo, Y. P., Liu, J., et al. (2022). Talaromarins A–F: six new isocoumarins from mangrove-derived fungus Talaromyces flavus TGGP35. Mar. Drugs 20, 361. doi: 10.3390/md20060361
Cao, X., Shi, Y., Wu, X., Wang, K., Huang, S., Sun, H., et al. (2019). Talaromyolides A-D and talaromytin: polycyclic meroterpenoids from the fungus Talaromyces sp. CX11. Org. Lett. 21, 6539–6542. doi: 10.1021/acs.orglett.9b02466
Chen, M., Han, L., Shao, C. L., She, Z. G., and Wang, C. Y. (2015). Bioactive Diphenyl ether derivatives from a gorgonian-derived fungus Talaromyces sp. Chem. Biodivers. 12, 443–450. doi: 10.1002/cbdv.201400267
Chen, S., He, L., Chen, D., Cai, R., Long, Y., Lu, Y., et al. (2017). Talaramide A, an unusual alkaloid from the mangrove endophytic fungus Talaromyces sp. (HZ-YX1) as an inhibitor of mycobacterial PknG. New J. Chem. 41, 4273–4276. doi: 10.1039/C7NJ00059F
Chen, C., Sun, W., Liu, X., Wei, M., Liang, Y., Wang, J., et al. (2019). Anti-inflammatory spiroaxane and drimane sesquiterpenoids from Talaromyces minioluteus (Penicillium minioluteum). Bioorg. Chem. 91:103166. doi: 10.1016/j.bioorg.2019.103166
da Silva, P., de Souza, M., Bianco, E., da Silva, S., Soares, L., Costa, E., et al. (2017). Antifungal polyketides and other compounds from amazonian endophytic Talaromyces fungi. J. Braz. Chem. Soc. 29, 622–630. doi: 10.21577/0103-5053.20170176
De Stefano, S., Nicoletti, R., Milone, A., and Zambardino, S. (1999). 3-o-Methylfunicone, a fungitoxic metabolite produced by the fungus Penicillium pinophilum. Phytochemistry 52, 1399–1401. doi: 10.1016/S0031-9422(99)00320-9
Dethoup, T., Kaewsalong, N., Songkumorn, P., and Jantasorn, A. (2018). Potential application of a marine-derived fungus, Talaromyces tratensis KUFA 0091 against rice diseases. Biol. Control 119, 1–6. doi: 10.1016/j.biocontrol.2017.11.008
Dewapriya, P., Khalil, Z. G., Prasad, P., Salim, A. A., Cruz-Morales, P., Marcellin, E., et al. (2018). Talaropeptides A-D: structure and biosynthesis of extensively N-methylated linear peptides From an Australian marine tunicate-derived Talaromyces sp. Front. Chem. 6:394. doi: 10.3389/fchem.2018.00394
Dewapriya, P., Prasad, P., Damodar, R., Salim, A. A., and Capon, R. J. (2017). Talarolide A, a cyclic heptapeptide hydroxamate from an Australian marine tunicate-associated fungus, Talaromyces sp. (CMB-TU011). Org. Lett. 19, 2046–2049. doi: 10.1021/acs.orglett.7b00638
Domínguez, J. M., Kelly, V. A., Kinsman, O. S., Marriott, M. S., Gómez de las Heras, F., and Martín, J. J. (1998). Sordarins: a new class of antifungals with selective inhibition of the protein synthesis elongation cycle in yeasts. Antimicrob. Agents Chemother. 42, 2274–2278. doi: 10.1128/AAC.42.9.2274
El-Elimat, T., Figueroa, M., Raja, H. A., Alnabulsi, S. M., and Oberlies, N. H. (2021). Coumarins, dihydroisocoumarins, a dibenzo-α-pyrone, a meroterpenoid, and a merodrimane from Talaromyces amestolkiae. Tetrahedron Lett. 72:153067. doi: 10.1016/j.tetlet.2021.153067
Frisvad, J. C., Yilmaz, N., Thrane, U., Rasmussen, K. B., Houbraken, J., and Samson, R. A. (2013). Talaromyces atroroseus, a new species efficiently producing industrially relevant red pigments. PLoS One 8:e84102. doi: 10.1371/journal.pone.0084102
Fu, G.-C., Yang, Z.-D., Zhou, S.-Y., Yu, H.-T., Zhang, F., and Yao, X.-J. (2016). Two new compounds, deacetylisowortmins A and B, isolated from an endophytic fungus, Talaromyces wortmannii LGT-4. Nat. Prod. Res. 30, 1623–1627. doi: 10.1080/14786419.2015.1129329
Guo, J.-P., Tan, J.-L., Wang, Y.-L., Wu, H.-Y., Zhang, C.-P., Niu, X.-M., et al. (2011). Isolation of talathermophilins from the thermophilic fungus Talaromyces thermophilus YM3-4. J. Nat. Prod. 74, 2278–2281. doi: 10.1021/np200365z
Guo, Y., Tu, T., Yuan, P., Wang, Y., Ren, Y., Yao, B., et al. (2019). High-level expression and characterization of a novel aspartic protease from Talaromyces leycettanus JCM12802 and its potential application in juice clarification. Food Chem. 281, 197–203. doi: 10.1016/j.foodchem.2018.12.096
Guo, J.-P., Zhu, C.-Y., Zhang, C.-P., Chu, Y.-S., Wang, Y.-L., Zhang, J.-X., et al. (2012). Thermolides, potent nematocidal PKS-NRPS hybrid metabolites from thermophilic fungus Talaromyces thermophilus. J. Am. Chem. Soc. 134, 20306–20309. doi: 10.1021/ja3104044
Halo, B. A., Al-Yahyai, R. A., Maharachchikumbura, S. S. N., and Al-Sadi, A. M. (2019). Talaromyces variabilis interferes with pythium aphanidermatum growth and suppresses pythium-induced damping-off of cucumbers and tomatoes. Sci. Rep. 9, 11255. doi: 10.1038/s41598-019-47736-x
Hayashi, H., Oka, Y., Kai, K., and Akiyama, K. (2012a). A new meroterpenoid, chrodrimanin C, from YO-2 of Talaromyces sp. Biosci. Biotechnol. Biochem. 76, 745–748. doi: 10.1271/bbb.110858
Hayashi, H., Oka, Y., Kai, K., and Akiyama, K. (2012b). New chrodrimanin congeners, chrodrimanins D–H, from YO-2 of Talaromyces sp. Biosci. Biotechnol. Biochem. 76, 1765–1768. doi: 10.1271/bbb.120365
He, J.-W., Liang, H.-X., Gao, H., Kuang, R.-Q., Chen, G.-D., Hu, D., et al. (2014a). Talaflavuterpenoid A, a new nardosinane-type sesquiterpene from Talaromyces flavus. J. Asian Nat. Prod. Res. 16, 1029–1034. doi: 10.1080/10286020.2014.933812
He, J.-W., Mu, Z.-Q., Gao, H., Chen, G.-D., Zhao, Q., Hu, D., et al. (2014b). New polyesters from Talaromyces flavus. Tetrahedron 70, 4425–4430. doi: 10.1016/j.tet.2014.02.060
He, J.-W., Qin, D.-P., Gao, H., Kuang, R.-Q., Yu, Y., Liu, X.-Z., et al. (2014c). Two new coumarins from Talaromyces flavus. Molecules 19, 20880–20887. doi: 10.3390/molecules191220880
Hong, X., Guan, X., Lai, Q., Yu, D., Chen, Z., Fu, X., et al. (2022). Characterization of a bioactive meroterpenoid isolated from the marine-derived fungus Talaromyces sp. Appl. Microbiol. Biotechnol. 106, 2927–2935. doi: 10.1007/s00253-022-11914-1
Huang, L.-J., Li, X.-A., Jin, M.-Y., Guo, W.-X., Lei, L.-R., Liu, R., et al. (2022). Two previously undescribed phthalides from Talaromyces amestolkiae, a symbiotic fungus of Syngnathus acus. J. Asian Nat. Prod. Res. 1–9. doi: 10.1080/10286020.2022.2075738
Jackson, R. S. (2008). Chemical constituents of grapes and wine. Wine Sci. 270–331. doi: 10.1016/B978-012373646-8.50009-3
Kaur, A., Raja, H. A., Swenson, D. C., Agarwal, R., Deep, G., Falkinham, J. O., et al. (2016). Talarolutins A-D: meroterpenoids from an endophytic fungal isolate of Talaromyces minioluteus. Phytochemistry 126, 4–10. doi: 10.1016/j.phytochem.2016.03.013
Kawaguchi, M., Uchida, R., Ohte, S., Miyachi, N., Kobayashi, K., Sato, N., et al. (2013). New dinapinone derivatives, potent inhibitors of triacylglycerol synthesis in mammalian cells, produced by Talaromyces pinophilus FKI-3864. J. Antibiot. 66, 179–189. doi: 10.1038/ja.2012.127
Koolen, H. H. F., Menezes, L. S., Souza, M. P., Silva, F. M. A., Almeida, F. G. O., de Souza, A. Q. L., et al. (2013). Talaroxanthone, a novel xanthone dimer from the endophytic fungus Talaromyces sp. associated with Duguetia stelechantha (Diels) R. E. Fries. J. Braz. Chem. Soc. doi: 10.5935/0103-5053.20130104
Kuml, D., Dethoup, T., Buttachon, S., Singburaudom, N., Silva, A. M. S., and Kijjoa, A. (2014). Spiculisporic acid E, a new spiculisporic acid derivative and ergosterol derivatives from the marine-sponge associated fungus Talaromyces trachyspermus (KUFA 0021). Nat. Prod. Commun. 9, 1147–1150. doi: 10.1177/1934578X1400900822
Küppers, L., Ebrahim, W., El-Neketi, M., Özkaya, F., Mándi, A., Kurtán, T., et al. (2017). Lactones from the sponge-derived fungus Talaromyces rugulosus. Mar. Drugs 15, 359. doi: 10.3390/md15110359
Li, H., Huang, H., Shao, C., Huang, H., Jiang, J., Zhu, X., et al. (2011). Cytotoxic norsesquiterpene peroxides from the endophytic fungus Talaromyces flavus isolated from the mangrove plant Sonneratia apetala. J. Nat. Prod. 74, 1230–1235. doi: 10.1021/np200164k
Li, H.-L., Li, X.-M., Liu, H., Meng, L.-H., and Wang, B.-G. (2016). Two new diphenylketones and a new xanthone from Talaromyces islandicus EN-501, an endophytic fungus derived from the marine red alga Laurencia okamurai. Mar. Drugs 14. doi: 10.3390/md14120223
Li, X.-F., Yang, Z.-D., Yang, X., Yang, L.-J., Yao, X.-J., and Shu, Z.-M. (2021). Wortmannine H, a phenylpentenol isolated from an endophytic fungus, Talaromyces wortmannii LGT-4. Nat. Prod. Res. 35, 3204–3209. doi: 10.1080/14786419.2019.1690488
Liu, F., Cai, X.-L., Yang, H., Xia, X.-K., Guo, Z.-Y., Yuan, J., et al. (2010). The bioactive metabolites of the mangrove endophytic fungus Talaromyces sp. ZH-154 isolated from Kandelia candel (L.) Druce. Planta Med. 76, 185–189. doi: 10.1055/s-0029-1186047
Liu, W.-C., Yang, F., Zhang, R., Shi, X., Lu, X.-H., Luan, Y.-S., et al. (2016). Production of polyketides with anthelmintic activity by the fungus Talaromyces wortmannii using one strain-many compounds (OSMAC) method. Phytochem. Lett. 18, 157–161. doi: 10.1016/j.phytol.2016.10.006
Luo, Y., Lu, X., Bi, W., Liu, F., and Gao, W. (2016). Talaromyces rubrifaciens, a new species discovered from heating, ventilation and air conditioning systems in China. Mycologia 108, 773–779. doi: 10.3852/15-233
Ma, M., Yi, W., Qin, L., Lian, X.-Y., and Zhang, Z. (2022). Talaromydien a and talaroisocoumarin A, new metabolites from the marine-sourced fungus Talaromyces sp. ZZ1616. Nat. Prod. Res. 36, 460–465. doi: 10.1080/14786419.2020.1779265
Matsuda, Y., and Abe, I. (2020). Fungal meroterpenoids. Compr. Nat. Prod. III, 445–478. doi: 10.1016/B978-0-12-409547-2.14663-3
Méndez-Líter, J. A., Tundidor, I., Nieto-Domínguez, M., de Toro, B. F., González Santana, A., de Eugenio, L. I., et al. (2019). Transglycosylation products generated by Talaromyces amestolkiae GH3 β-glucosidases: effect of hydroxytyrosol, vanillin and its glucosides on breast cancer cells. Microb. Cell Fact. 18, 97. doi: 10.1186/s12934-019-1147-4
Nam, I. H., Murugesan, K., Ryu, J., and Kim, J. H. (2019). Arsenic (As) removal using Talaromyces sp. KM-31 isolated from as-contaminated mine soil. Fortschr. Mineral. 9, 568. doi: 10.3390/min9100568
Naraghi, L., Heydari, A., Rezaee, S., and Razavi, M. (2012). Biocontrol agent Talaromyces flavus stimulates the growth of cotton and potato. J. Plant Growth Regul. 31, 471–477. doi: 10.1007/s00344-011-9256-2
Ngokpol, S., Suwakulsiri, W., Sureram, S., Lirdprapamongkol, K., Aree, T., Wiyakrutta, S., et al. (2015). Drimane sesquiterpene-conjugated amino acids from a marine isolate of the fungus Talaromyces minioluteus (Penicillium Minioluteum). Mar. Drugs 13, 3567–3580. doi: 10.3390/md13063567
Nicoletti, R., and Vinale, F. (2018). Bioactive compounds from marine-derived Aspergillus, Penicillium, Talaromyces and Trichoderma species. Mar. Drugs 16, 408. doi: 10.3390/md16110408
Nie, Y., Liu, Y., Yang, W., Li, Y., Xu, M., Lei, X., et al. (2019). Bioactive secondary metabolites from the fungus Talaromyces sp. isolated from coral Porites pukoensis. Mycosystema 38, 585–593. doi: 10.13346/j.mycosystema.180227
Noinart, J., Buttachon, S., Dethoup, T., Gales, L., Pereira, J. A., Urbatzka, R., et al. (2017). A new ergosterol analog, a new bis-anthraquinone and anti-obesity activity of anthraquinones from the marine sponge-associated fungus Talaromyces stipitatus KUFA 0207. Mar. Drugs 15, E139. doi: 10.3390/md15050139
Palem, P. P. C., Kuriakose, G. C., and Jayabaskaran, C. (2015). An Endophytic fungus, Talaromyces radicus, isolated from Catharanthus roseus, produces vincristine and vinblastine, which induce apoptotic cell death. PLoS One 10:e0144476. doi: 10.1371/journal.pone.0144476
Pandit, S. G., Puttananjaih, M. H., Harohally, N. V., and Dhale, M. A. (2018). Functional attributes of a new molecule-2-hydroxymethyl-benzoic acid 2′-hydroxy-tetradecyl ester isolated from Talaromyces purpureogenus CFRM02. Food Chem. 255, 89–96. doi: 10.1016/j.foodchem.2018.02.034
Ren, J., Ding, S.-S., Zhu, A., Cao, F., and Zhu, H.-J. (2017). Bioactive azaphilone derivatives from the fungus Talaromyces aculeatus. J. Nat. Prod. 80, 2199–2203. doi: 10.1021/acs.jnatprod.7b00032
Reyes, B. A. S., Dufourt, E. C., Ross, J., Warner, M. J., Tanquilut, N. C., and Leung, A. B. (2018). Selected phyto and marine bioactive compounds: alternatives for the treatment of type 2 diabetes. Stud. Natl. Prod. Chem. 111–143. doi: 10.1016/B978-0-444-64068-0.00004-8
Richardson, M., and Khosla, C. (1999). “1.18- Structure, function, and engineering of bacterial aromatic Polyketide synthases,” in Comprehensive Natural Products Chemistry. eds. S. D. Barton, K. Nakanishi, and O. Meth-Cohn (Pergamon: Oxford), 473–494.
Sakai, K., Asami, Y., Chiba, T., Suga, T., Nonaka, K., Iwatsuki, M., et al. (2018). Oxoberkedienoic acid: a new octadienoic acid derivative isolated from Talaromyces verruculosus using a chemical screening system. J. Gen. Appl. Microbiol. 64, 136–138. doi: 10.2323/jgam.2017.09.001
Sparkman, O. D., Penton, Z., and Kitson, F. G. (2011). Gas Chromatography and Mass Spectrometry: A Practical Guide. 2nd Edn. Boston, MA: Elsevier.
Uzma, F., Mohan, C. D., Hashem, A., Konappa, N. M., Rangappa, S., Kamath, P. V., et al. (2018). Endophytic fungi—alternative sources of cytotoxic compounds: a review. Front. Pharmacol. 9:309. doi: 10.3389/fphar.2018.00309
Vasil, I. K., Constabel, F., Bogorad, L., and Schell, J. S. (1984). Cell Culture and Somatic Cell Genetics of Plants. Orlando, FL: Academic Press.
Venkatachalam, M., Magalon, H., Dufossé, L., and Fouillaud, M. (2018). Production of pigments from the tropical marine-derived fungi Talaromyces albobiverticillius: new resources for natural red-colored metabolites. J. Food Compos. Anal. 70, 35–48. doi: 10.1016/j.jfca.2018.03.007
Vinale, F., Nicoletti, R., Lacatena, F., Marra, R., Sacco, A., Lombardi, N., et al. (2017). Secondary metabolites from the endophytic fungus Talaromyces pinophilus. Nat. Prod. Res. 31, 1778–1785. doi: 10.1080/14786419.2017.1290624
Visagie, C. M., Yilmaz, N., Frisvad, J. C., Houbraken, J., Seifert, K. A., Samson, R. A., et al. (2015). Five new Talaromyces species with ampulliform-like phialides and globose rough walled conidia resembling T. verruculosus. Mycoscience 56, 486–502. doi: 10.1016/j.myc.2015.02.005
Wang, N., Qiu, Y., Xiao, T., Wang, J., Chen, Y., Xu, X., et al. (2019). Comparative studies on Pb(II) biosorption with three spongy microbe-based biosorbents: high performance, selectivity and application. J. Hazard. Mater. 373, 39–49. doi: 10.1016/j.jhazmat.2019.03.056
Wang, W., Wan, X., Liu, J., Wang, J., Zhu, H., Chen, C., et al. (2018). Two new terpenoids from Talaromyces purpurogenus. Mar. Drugs 16, 150. doi: 10.3390/md16050150
Waters, D. M., Murray, P. G., Ryan, L. A., Arendt, E. K., and Tuohy, M. G. (2010). Talaromyces emersonii thermostable enzyme systems and their applications in wheat baking systems. J. Agric. Food Chem. 58, 7415–7422. doi: 10.1021/jf100737v
West, R. R., Ness, J. V., Varming, A. M., Rassing, B., Biggs, S., Gasper, S., et al. (1996). ZG-1494α, a novel platelet-activating factor acetyltransferase inhibitor from Penicillium rubrum, isolation, structure elucidation and biological activity. J. Antibiot. 49, 967–973. doi: 10.7164/antibiotics.49.967
Xian, H., Tang, W. A. L., and Li, D. (2011). Cloning and bioinformatics analysis of chitinase gene from mycoparasitic Talaromyces flavus. J. Agric. Biotechnol. 9, 1089–1098. doi: 10.3969/j.issn.16747968.2011.06.016
Xie, X.-S., Fang, X.-W., Huang, R., Zhang, S.-P., Wei, H.-X., and Wu, S.-H. (2016). A new dimeric anthraquinone from endophytic Talaromyces sp. YE3016. Nat. Prod. Res. 30, 1706–1711. doi: 10.1080/14786419.2015.1136888
Yang, H., Li, F., and Ji, N. (2016). Alkaloids from an algicolous strain of Talaromyces sp. Chin. J. Ocean. Limnol. 34, 367–371. doi: 10.1007/s00343-015-4316-2
Yang, Z.-D., Zhang, X.-D., Yang, X., Yao, X.-J., and Shu, Z.-M. (2021). A norbisabolane and an arabitol benzoate from Talaromyces marneffei, an endophytic fungus of Epilobium angustifolium. Fitoterapia 153:104948. doi: 10.1016/j.fitote.2021.104948
Yuan, W.-H., Teng, M.-T., Sun, S.-S., Ma, L., Yuan, B., Ren, Q., et al. (2018). Active metabolites from endolichenic fungus Talaromyces sp. Chem. Biodivers 15:e1800371. doi: 10.1002/cbdv.201800371
Zang, Y., Genta-Jouve, G., Escargueil, A. E., Larsen, A. K., Guedon, L., Nay, B., et al. (2016). Antimicrobial oligophenalenone dimers from the soil fungus Talaromyces stipitatus. J. Nat. Prod. 79, 2991–2996. doi: 10.1021/acs.jnatprod.6b00458
Zhai, M.-M., Niu, H.-T., Li, J., Xiao, H., Shi, Y.-P., Di, D.-L., et al. (2015). Talaromycolides A–C, novel phenyl-substituted phthalides isolated from the green Chinese onion-derived fungus Talaromyces pinophilus AF-02. J. Agric. Food Chem. 63, 9558–9564. doi: 10.1021/acs.jafc.5b04296
Zhang, M., Deng, Y., Liu, F., Zheng, M., Liang, Y., Sun, W., et al. (2021). Five undescribed steroids from Talaromyces stipitatus and their cytotoxic activities against hepatoma cell lines. Phytochemistry 189:112816. doi: 10.1016/j.phytochem.2021.112816
Zhang, M., Li, Q., Li, S., Deng, Y., Yu, M., Liu, J., et al. (2022a). An unprecedented ergostane with a 6/6/5 tricyclic 13(14 → 8)abeo-8,14-seco skeleton from Talaromyces adpressus. Bioorg. Chem. 127:105943. doi: 10.1016/j.bioorg.2022.105943
Zhang, K., Zhang, X., Lin, R., Yang, H., Song, F., Xu, X., et al. (2022b). New secondary metabolites from the marine-derived fungus Talaromyces mangshanicus BTBU20211089. Mar. Drugs 20, 79. doi: 10.3390/md20020079
Zhang, Y.-H., Zhao, Y.-J., Qi, L., Du, H.-F., Cao, F., and Wang, C.-Y. (2022c). Talasteroid, a new withanolide from the marine-derived fungus Talaromyces stollii. Nat. Prod. Res. 1–7. doi: 10.1080/14786419.2022.2070747
Zhao, J., Liu, Z., Sun, S., and Liu, Y. (2020). Investigation on secondary metabolites of endophytic fungus Talaromyces purpurogenus hosted in Tylophora ovate. China J. Chin. Mater. Med. 6, 1368–1373. doi: 10.19540/j.cnki.cjcmm
Zhao, W.-T., Shi, X., Xian, P.-J., Feng, Z., Yang, J., and Yang, X.-L. (2021a). A new fusicoccane diterpene and a new polyene from the plant endophytic fungus Talaromyces pinophilus and their antimicrobial activities. Nat. Prod. Res. 35, 124–130. doi: 10.1080/14786419.2019.1616727
Zhao, Y., Sun, C., Huang, L., Zhang, X., Zhang, G., Che, Q., et al. (2021b). Talarodrides A-F, nonadrides from the antarctic sponge-derived fungus Talaromyces sp. HDN1820200. J. Nat. Prod. 84, 3011–3019. doi: 10.1021/acs.jnatprod.1c00203
Zhao, J.-Y., Wang, X.-J., Liu, Z., Meng, F.-X., Sun, S.-F., Ye, F., et al. (2019a). Nonadride and Spirocyclic anhydride derivatives from the plant endophytic fungus Talaromyces purpurogenus. J. Nat. Prod. 82, 2953–2962. doi: 10.1021/acs.jnatprod.9b00210
Zhao, J.-W., Yang, Z.-D., Zhou, S.-Y., Yang, L.-J., Sun, J.-H., Yao, X.-J., et al. (2019b). Wortmannine F and G, two new pyranones from Talaromyces wortmannii LGT-4, the endophytic fungus of Tripterygium wilfordii. Phytochem. Lett. 29, 115–118. doi: 10.1016/j.phytol.2018.11.023
Keywords: Talaromyces, secondary metabolite, biological activity, polyketides, terpenoids, nitrogen compounds
Citation: Lei L-R, Gong L-Q, Jin M-Y, Wang R, Liu R, Gao J, Liu M-D, Huang L, Wang G-Z, Wang D and Deng Y (2022) Research advances in the structures and biological activities of secondary metabolites from Talaromyces. Front. Microbiol. 13:984801. doi: 10.3389/fmicb.2022.984801
Edited by:
Peng Zhang, Tobacco Research Institute (CAAS), ChinaReviewed by:
Dong-Lin Zhao, Tobacco Research Institute (CAAS), ChinaGuo-Bo Xu, Guizhou Medical University, China
Copyright © 2022 Lei, Gong, Jin, Wang, Liu, Gao, Liu, Huang, Wang, Wang and Deng. This is an open-access article distributed under the terms of the Creative Commons Attribution License (CC BY). The use, distribution or reproduction in other forums is permitted, provided the original author(s) and the copyright owner(s) are credited and that the original publication in this journal is cited, in accordance with accepted academic practice. No use, distribution or reproduction is permitted which does not comply with these terms.
*Correspondence: Dong Wang, dwang@cdutcm.edu.cn; Yun Deng, dengyun@cdutcm.edu.cn