- 1School of Life Sciences, University of Warwick, Coventry, United Kingdom
- 2Carbon, Crop and Soils Group, SRUC, Edinburgh, United Kingdom
The Brassica genus comprises the greatest diversity of agriculturally important crops. Several species from this genus are grown as vegetable and oil crops for food, animal feed and industrial purposes. In particular, B. oleracea has been extensively bred to give rise to several familiar vegetables (cabbage, broccoli, cauliflower, kale and Brussels Sprouts, etc.) that are grouped under seven major cultivars. In 2020, 96.4 million tonnes of vegetable brassicas were produced globally with a 10.6% increase over the past decade. Yet, like other crops, the production of brassicas is challenged by diseases among which, black rot, clubroot, downy mildew and turnip yellows virus have been identified by growers as the most damaging to UK production. In some cases, yield losses can reach 90% depending upon the geographic location of cultivation. This review aims to provide an overview of the key diseases of brassicas and their management practices, with respect to the biology and lifecycle of the causal pathogens. In addition, the existing controls on the market as well as those that are currently in the research and development phases were critically reviewed. There is not one specific control method that is effective against all the diseases. Generally, cultural practices prevent disease rather than reduce or eliminate disease. Chemical controls are limited, have broad-spectrum activity, are damaging to the environment and are rapidly becoming ineffective due to the evolution of resistance mechanisms by the pathogens. It is therefore important to develop integrated pest management (IPM) strategies that are tailored to geographic locations. Several knowledge gaps have been identified and listed in this review along with the future recommendations to control these four major diseases of brassicas. As such, this review paper will act as a guide to sustainably tackle pre-harvest diseases in Brassica crops to reduce food loss.
1. Introduction
Brassica is a genus in the Brassicaceae family, more colloquially known as the mustard or cabbage family. There are 37 species of Brassica (Gupta, 2016), many of which are of agricultural, economical and societal importance. Brassicas are the oldest and most widely consumed plants worldwide and are highly nutritious (Jabeen, 2020). They are consumed as vegetables, oils and condiments that are rich in vitamins A and C, antioxidants, dietary fiber, calcium, magnesium, and iron. The genomic relationship of the six most cultivated Brassica species is often depicted by the Triangle of ‘U’ (Figure 1; Nagaharu, 1935). The corner diploid species are Brassica rapa (genome AA, Chinese cabbage and turnips), Brassica nigra (genome BB, black mustard) and Brassica oleracea (genome CC, vegetable brassicas). The hybridisation of the diploid genomes gave rise to the allotetraploid species Brassica juncea (genome AABB, Chinese or brown mustard), Brassica napus (genome AACC, oilseed rape, swede and fodder kale) and Brassica carinata (genome BBCC, Ethiopian mustard).
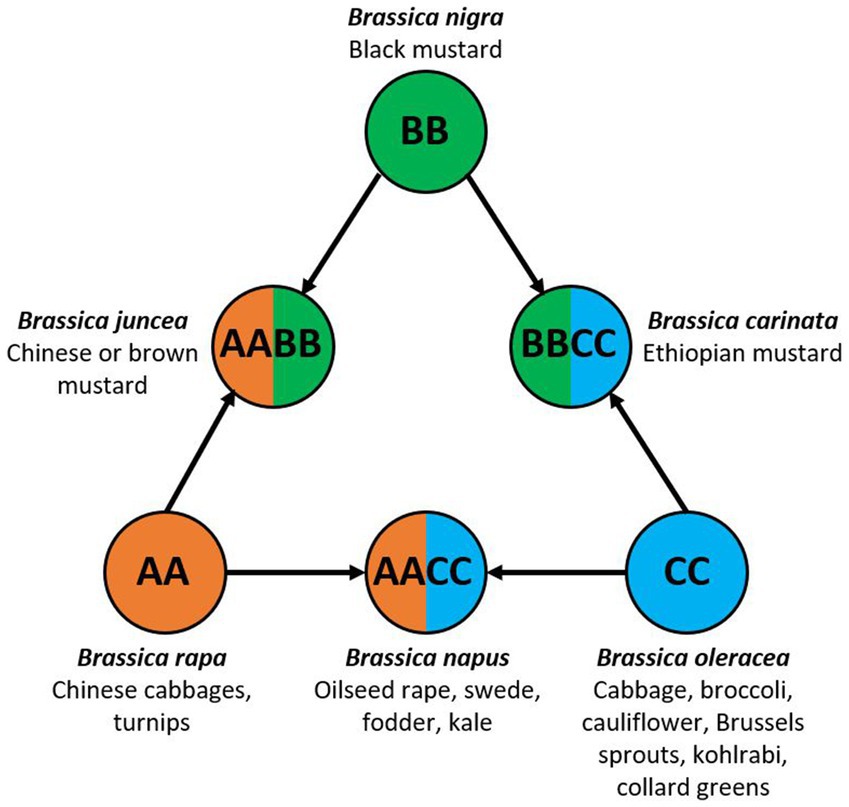
Figure 1. The triangle of ‘U’ showing the genomic relationships of six agronomically important Brassica species and their commonly associated crop types. Modified from Nagaharu (1935).
Of the six species in the triangle of U, B. oleracea is the most diverse and extensively bred. Within the species there are several subspecies/varieties, acephela (kale and collard greens), alboglabra (Chinese kale and broccoli), botrytis (cauliflower and Romanesco broccoli), capitata (cabbage), gemmifera (Brussels sprouts), gongylodes (kohlrabi) and italica (broccoli) (Labana and Gupta, 1993; Figure 1). Collectively they are known as the vegetable brassicas, and they grow best in temperate regions but are grown in most countries worldwide. The turn of the millennium saw a massive 33.8% increase in the production of vegetable brassicas like cabbage, cauliflower and broccoli from 68.1 to 91.1 million tonnes but since then, production has remained constant at an average of 89.7 million tonnes per annum (yearly average 2000–2020) (FAO, 2023a; Figure 2A). The largest producers of cabbage in 2020 were China (35.0 million tonnes), India (9.3 million tonnes) and Russia (2.6 million tonnes) (FAO, 2023a). With improvements in production and yield, the gross value of cabbage, cauliflower and broccoli has tripled to $32.3 billion USD in 2020 (FAO, 2023c; Figure 2A), underlining their global importance in both nutritional and food security.
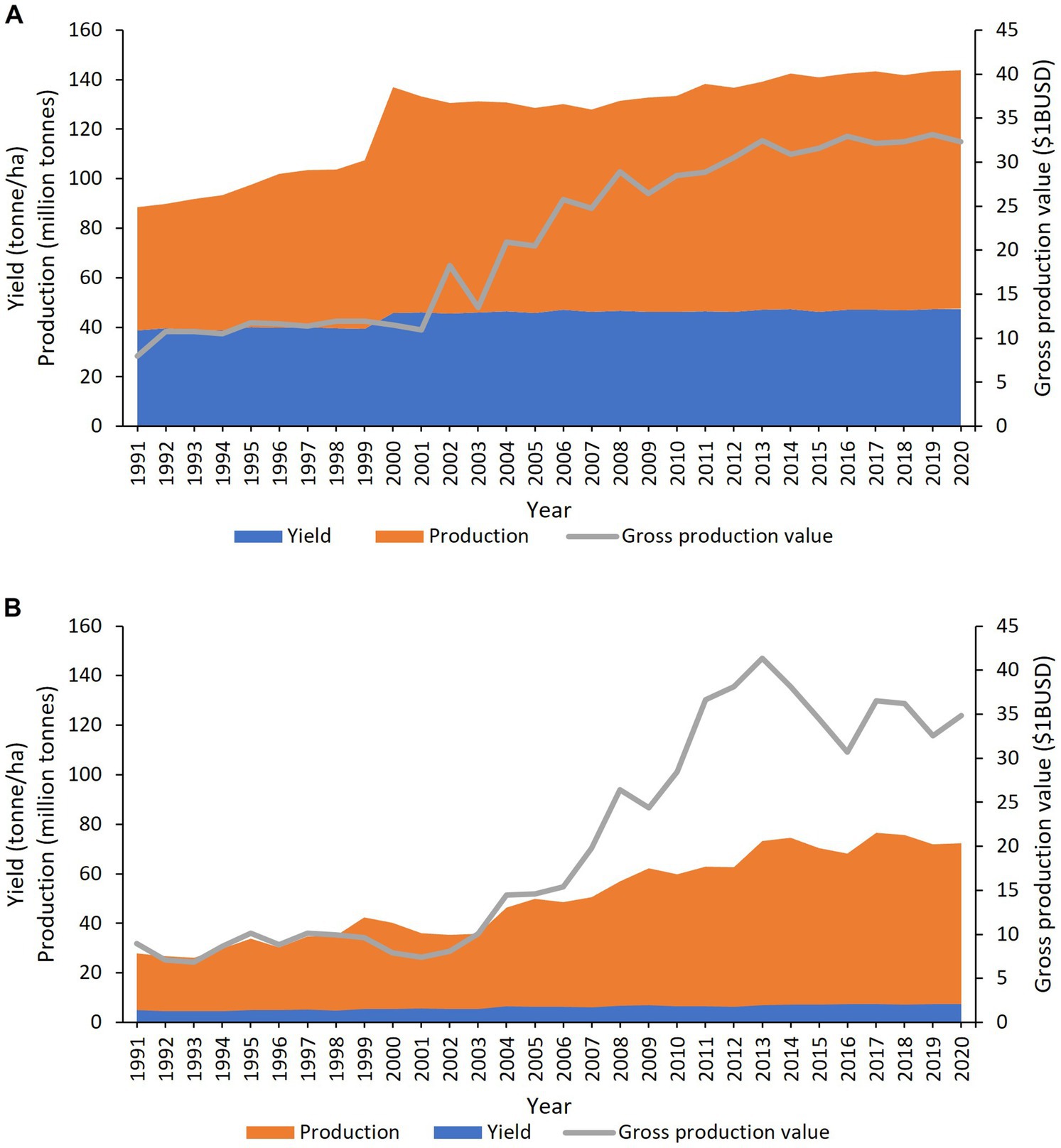
Figure 2. Worldwide production (orange), yield (blue) and gross production value (grey) of (A) vegetable brassicas (Brassica oleracea) cabbage, cauliflower and broccoli. (B) Oilseed brassicas (Brassica napus). Data from FAO (2023a,c).
The oilseed brassicas comprise of B. napus and B. juncea and less significantly B. carinata and B. rapa. Each have their vegetable forms. Rapeseed oil is the third most important oil after palm (Elaeis guineensis) and soybean (Glycine max) (USDA, 2020) and depending on the oil composition are used as edible oils, industrial lubricant and biofuels. The seed meal produced as a by-product of oil production, is used as a nutrient rich animal feed. From 2000 to 2020 rapeseed production increased 79.9% from 40.2 to 72.3 million tonnes but yield has remained consistently low at 1.8 tonnes/ha (FAO, 2023a; Figure 2B). In 2020, the largest producers of rapeseed were Canada (19.5 million tonnes), China (14.0 million tonnes) and India (9.1 million tonnes) (FAO, 2023a). Like vegetable brassicas, oilseed brassicas had a similar production value of $34.9 billion USD in 2020 (Figure 2B).
The FAO state that under ideal conditions cabbages could yield as much as 77 tonnes/ha but realized yield has remained consistently lower, averaging 46.5 tonnes/ha per annum (FAO, 2023a,b; Figure 2A). Similarly, rapeseed is only reaching a fifth of its predicted 9.2 tonnes/ha maximum yield potential (Berry and Spink, 2006; Figure 2B). Several biotic and abiotic factors contribute to this gap in achieved and potential yield, most notably pests and diseases which are thought to cause crop losses of 20–40% worldwide (Savary et al., 2012). The UK’s Research and Innovation strategic guidance, through consultation with farmers and growers has identified black rot (Xanthomonas campestris pv. campestris), aphids and vectored viruses (turnip yellows virus), downy mildew (Hyaloperonospora parasitica) and clubroot (Plasmodiophora brassicae) as the major pests and diseases of brassicas (UKRI, 2022). In agriculture, plant protection is delivered by one or more combinations of the following methods farm practise, chemical and biological controls, resistance cultivars and integration of all these methods, known as an integrated pest management (IPM) strategy. In this review, we will discuss the current knowledge of these diseases and their management practices with respect to the biology and lifecycle of the causal pathogens. We will critically review the existing controls on the market and those in the research and development phases. For each disease we have provided existing and emerging control strategies and also highlighted the current knowledge gaps.
2. Black rot
2.1. Host and impact
Black rot of the Brassicaceae family is caused by the Gram-negative bacterium Xanthomonas campestris pv. campestris (Xcc). The disease was first reported in Kentucky, USA in 1889 (Garman, 1890) and the casual pathogen later described in 1895 (Pammel, 1895), the name of which has changed several times since, Bacillus campestris (Pammel, 1895), Pseudomonas campestris (Smith, 1898), Bacterium campestre (Smith, 1898), Phytomonas campestris (Bergey et al., 1923) and Xanthomonas campestris pv. campestris (Dowson, 1939). There are nine races of Xcc, which are defined by their pathogenicity on a panel of Brassica accessions (Vicente et al., 2001). Races 1, 4, 5, and 6 are the most prevalent worldwide, with races 1 and 4 most common in vegetable brassicas (Vicente et al., 2001; Jensen et al., 2010; Mulema et al., 2012; Singh et al., 2016).
Black rot symptoms are distinct, characterized by ‘V’ shaped chlorotic and necrotic lesions (Figure 3) that begin at the leaf margin, where Xcc enters the vascular xylem tissue through hydathodes. The lesions expand as Xcc migrates toward the leaf mid vein and the vascular tissue can blacken. In severe cases, Xcc can move systemically throughout the plant causing wilting and rot (Figure 3C).
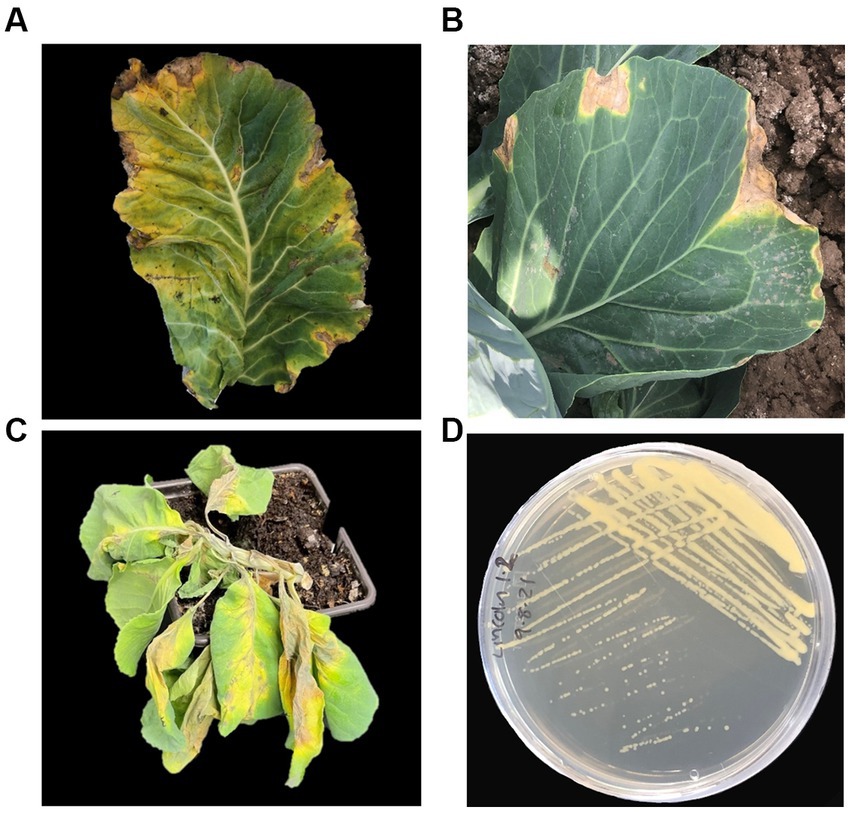
Figure 3. Disease symptoms and morphology of Xanthomonas campestris pv. campestris (Xcc). Symptoms of black rot caused by Xcc on (A) cauliflower (Brassica oleracea var. botrytis) and (B) cabbage (Brassica oleracea var. botrytis). (C) Wilt of cabbage caused by Xcc (photograph by Srayan Ghosh). (D) Xcc plated on King’s B media.
Xcc is now present in 93 countries and 6 continents (CABI, 2022d). It is considered the most damaging bacterial disease of vegetable brassicas worldwide, causing yield losses of up to 60% in susceptible cultivars (Gupta, 1991; McKeen, 2009). Xcc also infects oilseed brassicas but is not widely reported and its impact on yield and oil quality is yet to be investigated (Popović et al., 2013, 2019). Xcc can act as a gateway pathogen for other diseases like soft rots caused by Pseudomonas and Pectobacterium species that can lead to total losses of stored brassicas like white cabbage. Xcc thrives in warm, humid conditions and so disease outbreaks could become more frequent and yield losses exacerbated with climate change (Kůdela et al., 2018).
2.2. Pathogen lifestyle and transmission
Xcc is a seed-transmissible disease, and its worldwide distribution is primarily the result of trading and sowing infected seed. This is supported by the fact that Xcc isolates from different geographic regions can be genetically homogenous (Lange et al., 2015). On a more local scale, Xcc can be transmitted by wounding and water dispersal, a major source being the overhead watering of vegetable brassica seedlings in the glasshouse before transplant to the field. The bacteria can also persist between brassica crop rotations in the soil and crop debris for up to two years, depending on climatic conditions (Silva Júnior et al., 2020; Gazdik et al., 2021) and in weed hosts like Sheperd’s purse and fanweed. However, studies have shown that weed isolates are genetically distinct from those isolated from crops (Ignatov et al., 2007) and most are unable to cause disease in vegetable brassicas (Krauthausen et al., 2018). This suggests that weeds are potentially not a major inoculum source. It has been suggested that Xcc can be transmitted by insects, but this has yet to be proven (Vicente and Holub, 2013; An et al., 2020).
2.3. Diagnostics
As seeds are the primary Xcc inoculum source they are usually tested to prevent transmission. Seed-extracts are cultured on medias such as Fieldhouse-Sasser, mCS20ABN and King’s B (Koenraadt et al., 2005) where Xcc forms yellow-mucoid colonies (Figure 3D). There cultures are then confirmed by serology (Alvarez, 1994), PCR (Berg et al., 2005) or fatty acid methyl ester analysis (FAME) (Massomo et al., 2003). Positive results are then followed up with host plant assays to test pathogenicity (International Seed Federation, 2019). As black root causes distinct symptoms, often diagnosis is based on visual symptoms alone. However, at the point of symptom development, infection is already well established and consequently the effectiveness of control methods is limited. Currently, diagnostic methods are destructive, lab-based and time-consuming so there is a need for the development of rapid in-field diagnostics, e.g., loop-mediated isothermal amplification (LAMP) and Lateral Flow Tests (LFTs), which have been developed for Xanthomonas pathogens of other crops (Hodgetts et al., 2015; López-Soriano et al., 2017; de Paiva et al., 2019).
Some generalized tools for bacterial disease identification and management have been produced for brassicas in Europe, e.g., CropMonitor Pro, Taranis and xavario™ SCOUTING (SmartProtect, 2023). However, there are no specific forecasting tools for black rot incidence and disease severity. Development of such a tool that incorporates environmental conditions (temperature, humidity, rainfall, etc.), Xcc race-type, crop cultivar., watering systems and geographical region could be beneficial in preventing and controlling Xcc.
2.4. Prevention and management
2.4.1. Farm practices
It is routine for brassica seed to be tested and where possible, only certified Xcc-free seed should be sown. Where farm saved seed is used it should be sent for testing and at a minimum sterilized prior to sowing. It is commonly recommended to sterilize brassica seed in hot water (50°C) for 20-30 min and this has been shown to reduce disease levels in the field (Roberts et al., 2022). However, hot water treatment alone does not completely eradicate Xcc on the seed and hydrogen peroxide washes are more effective and do not impact germination (Sanna et al., 2022). Similarly, UV-C treatment of seeds and plants has been shown to reduce disease incidence, but optimal ranges were narrow 2.5–3.6kJm−2; lower doses were not effective in eliminating Xcc and higher doses were detrimental to germination and yield (Brown et al., 2001). The application of UV-C to field crops would also be challenging.
The AHDB (2023a) recommend that brassicas should be sown in sterilized or new pots and ideally watered using an ebb and flow system that reduces water splash transmission of Xcc caused by traditional over-head systems. Inter-cropping with Xcc non-hosts like lettuce and legumes also has the potential to reduce transmission via water splash in the field by reducing crop density. However, this method is not widely practised and has been explored in the context of nutrient acquisition and nitrogen fixation but not black rot disease suppression (Stavridou et al., 2012; Jeromela et al., 2017). The AHDB also recommend that brassicas should be grown in 3-year field rotations to avoid build-up of Xcc in the soil from infected crop debris, which should be removed and burned directly after harvest and not ploughed into the field. It is still recommended to remove cruciferous weeds from the field, even though they are no longer thought to be a major inoculum source (Ignatov et al., 2007; Krauthausen et al., 2018). Field equipment, e.g., tractors and sprayers should be sterilized and used in uninfected areas first to prevent transmission through wounding. Finally diseased material should not be stored to prevent contamination and potential loss of the whole consignment.
2.4.2. Chemical control
There are many chemical control options for Xcc, but they are generally ineffective as they are applied when symptoms are visible, and thus disease already established. Moreover, they are also damaging to the environment and human health. A study by Krauthausen et al. (2011) showed that benzoic acid and copper hydroxide sprays were only 12 and 32% effective, respectively, in reducing transmission in cauliflower transplants watered by overhead systems. Other studies on copper containing chemicals and pesticides (CuSO4, Kocide and copper nanoparticles) have shown a range in effectiveness from no control (Lenka and Ram, 1997) to reduced disease severity (Dong et al., 2020) and enhancing other treatments when used in combination (Patikarnmonthon et al., 2010; Nuñez et al., 2018). The most effective chemical treatment in the Krauthausen et al. (2011) study was enrichment of the overhead irrigation water with chlorine dioxide which reduced transmission by 94%. Antibiotics like streptocycline, streptomycin, oxytetracycline, chloramphenicol and rifampicin used as seed treatments and sprays have been shown to be more effective at controlling Xcc (Lenka and Ram, 1997; Bhat and Masoodi, 2000). However, the use of antibiotics and copper compounds to control bacterial plant pathogens can co-select for antimicrobial resistances (AMR) and so poses a serious threat to human health. Despite this, many countries do not regulate the use of antibiotics in crops, although many EU countries and the UK do not have any licensed as plant protection products (Haynes et al., 2020).
2.4.3. Biological control
There are very few biocontrol agents for black rot approved for commercial use. One such product is Actigard, a broad acting pesticide that induces systemic acquired resistance (SAR) and has been shown to reduce black rot incidence by 51% (Langston and Cummings, 2003). Fungal biocontrol agents like Trichoderma spp. work in a similar way by inducing SAR and have been shown to control Xanthomonas spot of tomato (Fontenelle et al., 2011). Whether this phenotype translates to brassica crops needs further investigation. The Trichoderma metabolite 6-pentyle-α-pyrone (6PP) and botanical extracts also look promising as controls as they have been shown to inhibit Xcc biofilm formation and bacterial growth (Sain et al., 2007; Papaianni et al., 2020).
For more precise targeting of Xcc, bacteriocins and phages can be used. Bacteriocins are small proteins produced by bacteria to kill other closely related bacteria, helping them establish a niche in their environment (Daw and Falkiner, 1996). Bacteriocins targeting other Xanthomonads have been identified (Pham et al., 2004; Bonini et al., 2007; Marutani-Hert et al., 2020) but none that target Xcc. These could be predicted through sequencing and analyses of Xcc and other closely related Xanthomonads and could be applied as seed or spray treatments. Conversely, numerous phages have been identified that target Xcc (Orynbayev et al., 2019; Holtappels et al., 2022), but generally they are fragile and easily degraded by environmental UV-B. A study by Orynbayev et al. (2020) showed that skimmed-milk gave phages the best protection against UV-B. A subsequent study by Nuñez et al. (2018) showed that milk-based products alone can reduce Xcc severity in kale under field conditions by up to 44%, as well as simultaneously improve nutritional value. So, a combination of milk-based products and phage could cumulatively improve Xcc control. While promising, the majority of biocontrols for black rot are in the research and development phases and require considerably more work to realize a marketable product.
2.4.4. Resistant cultivars
Host resistance provides an alternative to chemical control but is challenging due to the genetic diversity and race structure of Xcc. Several brassica varieties are reported to be resistant or have field tolerance to black rot (Table 1) but their effectiveness against different Xcc races is not known and is limited in certain crop types like savoy cabbage and cauliflower. Over 30 resistance sources have been reported in the literature and several QTLs have been mapped (Taylor et al., 2002; Singh et al., 2018; Shaw et al., 2021). Most of the resistances identified are race-specific, mostly to the prevalent races 1 and 4, and in most instances are from other Brassica spp. (B. rapa, B. nigra, B. carinata, B. napus and B. juncea) so require introgression into commercial vegetable brassica (B. oleracea) types, where currently there is limited resistance. This will be time consuming as it can take >10 years to introgress traits using traditional breeding methods. This could be accelerated with gene-editing/modification techniques, but this would require further mapping to refine QTLs and identify specific resistance genes to target. Race-specific diagnostics of Xcc will help inform the deployment of the correct resistances and resistance stacking may be necessary in areas where multiple races are prevalent.
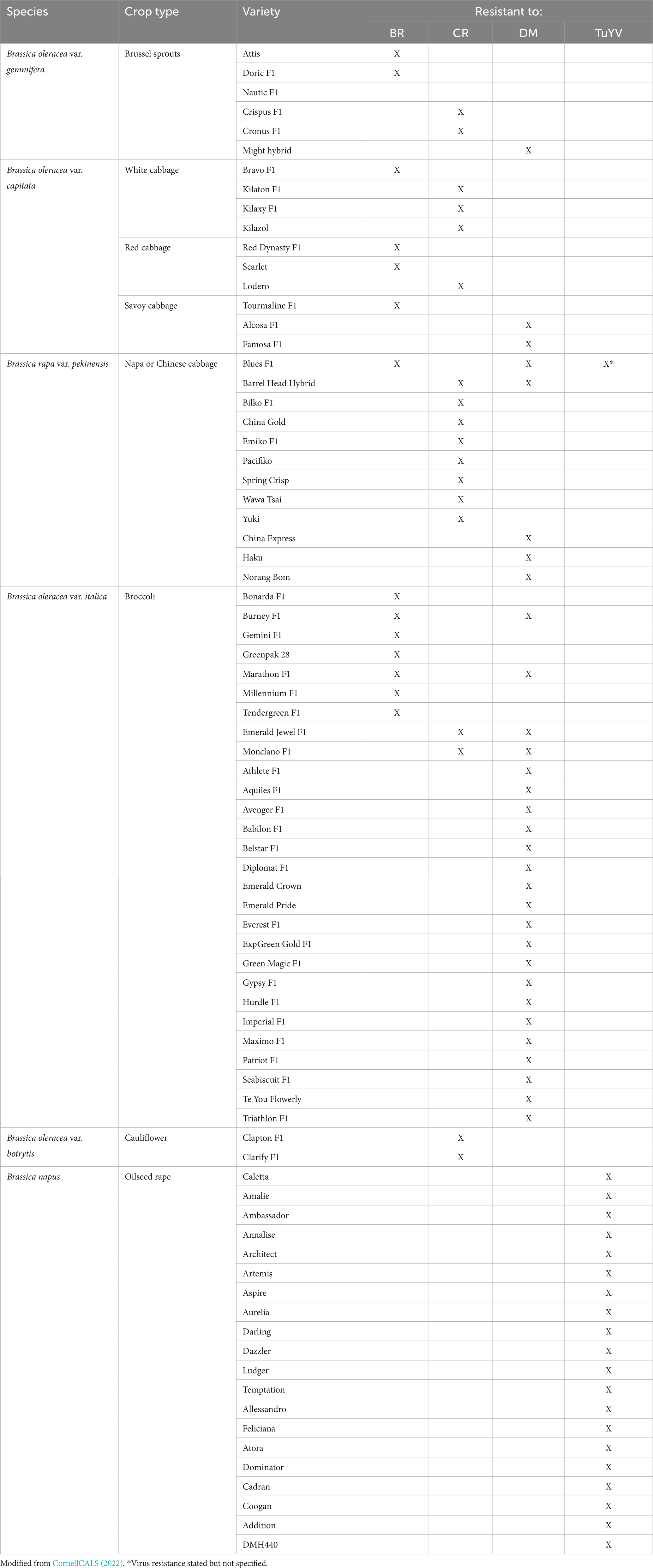
Table 1. Black rot (BR), clubroot (CR), downy mildew (DM), and turnip yellows virus (TuYV) resistant Brassica varieties.
3. Clubroot
3.1. Host and impact
Clubroot disease is an age-old disease that was believed to affect cultivated brassicas in Europe at least as far back as the 13th century and quite possibly as early as the Roman era. It has been ascribed to many causes but in 1873, the protist pathogen Plasmodiophora brassicae was identified as the causal agent (Chupp, 1934). The disease is especially prevalent in, but it is not limited to, moist and temperate areas. The epidemics are developing rapidly as the dietary, and industrial significance and cultivation of Brassica crops increases. The exact origin of clubroot disease is unknown, however, it is believed that the pathogen traveled from Europe to other parts of the world by means of infected plants used as animal fodder (Gibbs, 1931). Clubroot disease is a global problem, it is reported to be distributed in more than 88 countries and can result in a 10–100% reduction in yield (Saharan et al., 2021). In some countries, this disease is widespread and significantly affects yield losses (Table 2).
A classical symptom of clubroot infection is the formation of white-colored solid root galls which later discolor and rot (Figure 4). Above-ground symptoms appear only in the final stages of infection and include stunting and yellowing of the leaves. Under dry conditions, severe galling can inhibit water uptake from the soil and cause wilting. In severely infected areas the whole crop can fail (Saharan et al., 2021).
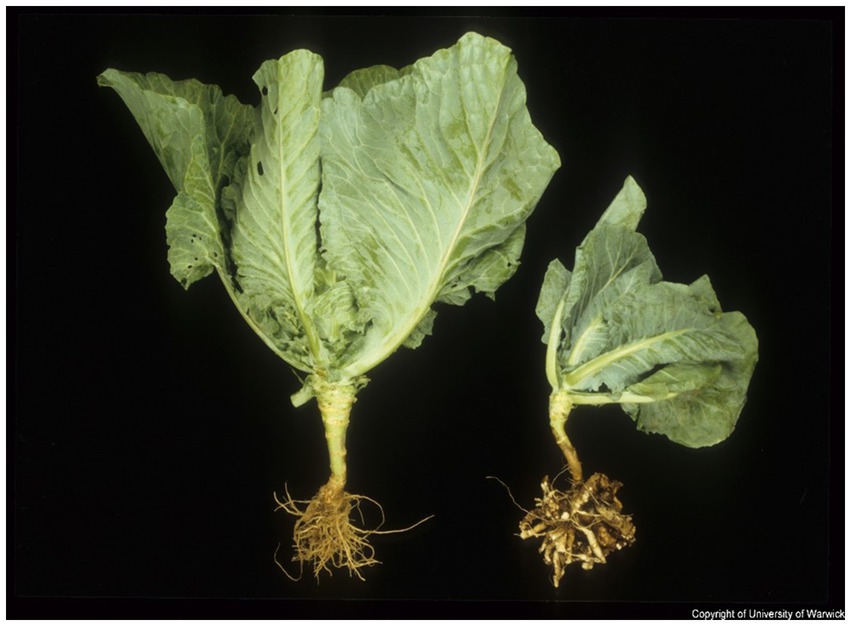
Figure 4. Symptoms of clubroot infection in Brassica caused by Plasmodiophora brassicae (photograph by John Walsh). The left plant is healthy, and the right plant is infected with P. brassicae and shows classic root gall but no foliar symptoms.
It is believed that P. brassicae is a potential pathogen for all 330 genera and 3,700 species in the Brassicaceae family. Apart from most vegetables, oil, and fodder crops, P. brassicae also infects cruciferous weeds such as Shepherd’s purse, stinkweed, wild mustard and ornamental plants such as Matthiola spp. and Cheirantus cheiri (Buczacki, 1979; Howard et al., 2010; Saharan et al., 2021). The wide host range of P. brassicae make it particularly difficult to manage. The incidence and virulence of the pathogen is greatly dependent on the host and is considered a ‘Disease of cultivation’.
3.2. Pathogen lifestyle and transmission
As a soilborne pathogen, P. brassicae presents abundant challenges to agriculturists and biological scientists due to its complex lifecycle (Saharan et al., 2021). The pathogen is obligate, intracellular, non-axenic, microscopic, single-celled, and soilborne with dormant, well-protected resting spores. These spores are composed of layers of chitin and carbohydrates (Moxham and Buczacki, 1983) and can survive for up to 20 years in the soil (Dixon, 2009). Consequently, clubroot disease is almost impossible to eradicate once it is established.
Plasmodiophora brassicae infection can be broadly divided into primary and secondary infections. During primary infection resting spores germinate in the presence of brassica root exudates in the soil (Bochow, 1963, 1965). However, germination can be triggered by root exudates from other plants such as poppy (Papaver spp.), strawberry (Fragaria spp.), and ryegrass (Lolium spp.) (Craig, 1989). The resting spores germinate into a primary zoospore which has two flagella, and it enters the host via root hairs (Kageyama and Asano, 2009). This is the only stage of P. brassicae that is considered unprotected (without spore walls) (Burnett et al., 2019). The conducive environment for the primary zoospores to survive and enter the hosts are acidic pH (<6.8), low calcium, nitrogen in the form of ammonia and water logged soil with a temperature of greater than 15°C (AHDB, 2020). The primary zoospores colonize the root hair and produce primary plasmodia inside the host. Secondary zoospores are then released into the soil.
Secondary zoospores are the starting point for secondary infection, they form secondary plasmodium in host cells and neighboring cells undergo extreme hyperplasia (cell division) and hypertrophy (cell expansion), resulting in the formation of galls. The gall formation affects the vascular tissues in the root which limits the transport of nutrients and water to aerial parts of the infected plants causing yellowing and stunting (Ludwig-Müller et al., 2009). These symptoms only appear at the later stage of infection. Disintegration of the galls releases resting spores into the soil to start the infection cycle again.
3.3. Diagnostics
The greatest challenge of managing clubroot disease in the field is the lack of rapid and reliable detection methods and/or tools. Historically, clubroot infection was detected by the “plant bioassay method,” where bait plants are grown in suspect soil for 5–6weeks and subsequently examined for root galls (Dixon, 2009). Although this method is reliable and inexpensive, it is extremely time-consuming, labor intensive, and the results are highly influenced by environmental factors (Dixon, 2009). Alternative diagnostic methods include microscopic observation of host tissues, fluorochrome staining of P. brassicae’s resting spores in the soil (Takahashi and Yamaguchi, 1988, 1989) and the application of monoclonal antibodies in serological methods (Dixon, 2009). PCR can also be used to check seeds and tubers for P. brassicae (Rennie et al., 2011). These methods are reliable and sensitive, however, they are lab-based, require expensive equipment and technically skilled persons.
Global Positioning System (GPS), and Geographic Information System (GIS) have been used to help visualize and track the spread of P. brassicae around infected regions (Saharan et al., 2021). CLIMEX is a forecasting tool for clubroot distribution and disease severity and was developed in Canada (Howard et al., 2010). It uses temperature and moisture content data but requires further refinement to include various other soil parameters, e.g., nutrient content presences of alternative host species, P. brassicae pathotypes, crop cultivar and geographical region to be an effective tool to control clubroot disease. A major hinderance in developing forecast tools is the lack of previous data, farmers are often aware of the factors that influence clubroot disease but most of them fail to record these (Saharan et al., 2021). Further development of geographic specific modeling tools is required to effectively control clubroot disease in brassicas.
3.4. Prevention and management
3.4.1. Farm practices
Clubroot disease can be controlled by clean farm practice. Any activity that moves the soil or plants from an infected field to another has the potential to spread P. brassicae. It is important to disinfect machinery, equipment, vehicles, tools, footwear, seeds, plant materials, water and/or soil to prevent the spread of clubroot. Seed contamination can cause long-distance dissemination of P. brassicae and so seed cleaning is particularly important. Hwang et al. (2008) showed that most commercial disinfectants except sodium hypochlorite solution (1–2%) were ineffective at deactivating P. brassicae spores after a 20–30 min contact time. The study showed that sanitization can limit the P. brassicae pathogen but not eradicate it. Timing of pre-and post-harvest weed management is also crucial for controlling clubroot disease because the cruciferous weeds could act as a potential host for P. brassicae (Friberg et al., 2005; Ahmed et al., 2011).
Soil solarization is one of the methods used in tropical regions to control clubroot disease (Jacobsohn et al., 1980). In this technique, the infected soil is covered by a polyethene sheet to trap the heat and inactive P. brassicae spores. This technique is efficient in sandy soil with low disease pressure but requires the addition of fumigation agents in clay soils and when the disease pressure is high (Donald and Porter, 2014). Acidic soil often favors clubroot development and hence soil liming is considered an effective practice to control the disease. In this method, the soil pH is maintained around 7.2 or higher by the addition of lime (Myers and Campbell, 1985; Rastas et al., 2012). The lime is available in slow-acting and fast-acting forms. The slow-acting forms of lime such as agricultural lime and dolomitic lime are applied during autumn at 5–7.5 t/ha to allow time for them to react and diffuse so that soil is prepared for spring planting (Hwang et al., 2008). Fast-acting lime such as hydrated lime and quick lime is applied before planting. An important aspect to consider in this method is that liming effect is greatly varied depending upon the soil type. This method is inapplicable in soil with high buffering capacities and in ‘lime non-responsive’ soil (Myers et al., 1981).
High soil moisture content at 25°C favors clubroot (Feng et al., 2010; Sharma et al., 2011) and so it is recommended where possible to avoid these condition at the early stage of seedling development, whereas older plants are less affected. Gossen et al. (2012) showed that early sowing of Brassica plants reduced the disease severity by 10–50% and increased the yield by 30–58%. Longer crop rotations of more than 3 years are recommended to reduce disease build-up in the field. Further intercropping with Yellow Sarson and flaxseed has also been shown to reduce clubroot severity by 30–50% (Poulton, 1990). The presence of hydrogen cyanide in root exudates is additionally hypothesized to inhibit the clubroot pathogen (Poulton, 1990). Various farm practices are recommended for clubroot disease however it is greatly dependent on the local environment.
3.4.2. Chemical control
Very few chemicals are known to exhibit consistent control of clubroot disease in Brassica. Among them, the most efficient and widely used chemical is mercurous chloride (Calomel™). However, it has been withdrawn from the market due to its mercury toxicity and persistence in the environment for long periods (Saharan et al., 2021). Currently, the most used chemicals to control clubroot disease are benzimidazoles and their precursors. Seed treatments have been shown to reduce the severity of the disease and increase yield (Hwang et al., 2011) but another study has shown that they only reduce the dissemination of P. brassicae via seed and give no protection in the field (Rod, 1992). The major limitation in chemical control is the product delivery to the infected parts and secondly, most of the chemicals are environmental pollutants. An effective way to reduce the P. brassicae inoculum in the field is to fumigate the soil with dithiocarbamates such as metham sodium and dazomet. When these products get into contact with moist soil, they are converted to volatile compounds that diffuse through the soil and act as a fungicide. The fumigation activity is greatly dependent on the moisture content (the optimal moisture content is around 10–30%) and the soil type (Donald and Porter, 2014). The downside of utilizing fumigants is that it causes localized air pollution.
The application of calcium cyanamide fertilizer plays an important role in IPM. The slow-release nature of the fertilizer reduces nitrate pollution and makes calcium readily available, which in turn encourages microbial diversity and suppresses soil-borne pathogens like P. brassicae (Dixon, 2009). Various researchers have identified calcium cyanamide as an effective control for soil-borne pathogens (Tremblay et al., 2005; Donald and Porter, 2014).
3.4.3. Biological control
The weakest link in the lifecycle of P. brassicae is the zoospore stage and so, many disease management strategies target this stage. Using bait crops in combination with liming could control clubroot disease (Harling and Kennedy, 1991). Bait crops, like radish stimulate the germination of resting spores to zoospores (Bhattacharya et al., 2014). However, this strategy is only advised when the disease severity is at a lower-moderate level. The greatest disadvantage of this method is that it is time-consuming, most bait crops are hoed in after 6weeks and during this time the land is out of production. The application of seaweed (Posidonia australis) has also been shown to stimulate the germination of P. brassicae resting spores and reduce the disease severity (Tilston et al., 2002). There are no commercial stimulants on the market that could be used for inducing resting spore germination.
Many common microorganisms residing in the rhizosphere and root cortex of common vegetables and field crops do not flourish in the presence of Brassica species (Usuki and Narisawa, 2007). This unique characteristic of Brassica crops limits the application of common biocontrol agents that are rhizosphere colonizers and producers of antimicrobial compounds (Saharan et al., 2021). The most common biocontrol agents for clubroot disease are Bacillus subtilis (Serenade®), Gliocladium catenulatum (syn. Clonostachys rosea f. catenulate) (Prestop®), Streptomyces griseoviridis (Mycostop®), S. lydicus (Actinovate®), and Trichoderma harzianum (RootShield®) (Sharma et al., 2011; Saharan et al., 2021). The formulation plays a crucial role in the survival and delivery of biocontrol agents. B. subtilis has an advantage over other biocontrol agents in respects to formulation because it is capable of producing spores under adverse conditions (Schisler et al., 2004). Serenade® and Prestop® are more effective under lower P. brassicae inoculum pressure, with 85–100% reduction in disease severity (Peng et al., 2011). However, Mycostop® was more effective than Serenade® and Prestop® at the higher disease pressure (Adhikari, 2010).
Plant bio-stimulants like algae extract, amino acids, and phosphonate are also used to suppress clubroot disease. Kammerich et al. (2014) showed that two commercial bio-stimulants Frutogard® (liquid) and PlasmaSoil® (granules) reduced disease severity and gall size on B. rapa (Chinese cabbage) and B. napus (oilseed rape). However, few studies have been conducted at the field level to test effectiveness of biocontrol agents against clubroot disease and most of the studies presented in this section were conducted in a controlled environment.
3.4.4. Resistant cultivars
Sources of resistance to P. brassicae have been identified in various parts of the world. Breeding is considered the most effective disease management strategy for clubroot. Resistance to P. brassicae can vary from broad-spectrum resistance, effective against several races and pathotypes to highly specific to one strain. Numerous resistance varieties have been developed around the world (Table 1) but their effectiveness against different P. brassicae races and pathotypes is not known and is limited in crop types like broccoli and savoy cabbage.
Genetic mapping has revealed several dominant loci, twelve in B. rapa, >22 QTLs in B. oleracea and > 19 QTLs in B. napus for clubroot resistance to different pathotypes of P. brassicae (Saharan et al., 2021). The first resistance cultivars were Mendel and Tosca. These cultivars are heterozygous hybrids with pathotype-specific resistance. Unfortunately, the Mendel host resistance was rapidly broken down in Germany, France and the UK as a result of P. brassicae quickly adapting to overcome the resistance (Saharan et al., 2021). This is an example of why resistance cultivars must be used with care; they should be used in combination with other resistances and management practises to minimize the risk of resistance breakdown. The Mendel and Tosca resistances have been shown to be highly variable, sometimes being in-effective in some regions and to some pathotypes of P. brassicae (Peng et al., 2013, 2014).
It is expected that the market will receive more resistance varieties in the next decade due to the development of molecular techniques (Peng et al., 2014; Saharan et al., 2021). However, these resistances may break down without sustainable agronomic practices and pathogen resistance management strategies.
4. Downy mildew
4.1. Host and impact
The term downy mildew was first used in the USA to describe fungus that appeared as white-colored outgrowths caused by the proliferation and fructification of mycelium on the surface of green and necrotic plant tissues (Saharan et al., 2017). Downy mildew is caused by Hyaloperonospora parasitica syn. Paronospora parasitica, an obligate biotrophic oomycete in the family Peronosporaceae, a distinct group of pathogens classified as Mastigomycotina in the order Peronosporales (Voglmayr, 2003; Göker et al., 2009). H. parasitica remains understudied because it is difficult to culture in artificial media and collect pathogen samples due to its obligate nature. It originated from northern parts of America, and quickly adapted to European weather. It is currently widespread around the world, with reports from 93 countries across 6 continents (CABI, 2022b).
The host range for downy mildew is vast, particularly in the Brassicaceae where it infects 50 genera and more than 100 species (Gaumann, 1923). Hyaloperonospora parasitica infects more than 20 economically important Brassica crops, with the greatest impact on mustard (B. nigra and B. juncea) and oil seed rape (B. napus) (Saharan et al., 2017). The pathogens broad host range make it difficult to control. The symptoms of downy mildew infection of brassica are usually seen first in the cotyledons, followed by the true leaves with the appearance of pale green-yellowish spots, which are angular in shape and bound to the leaf vein (Figure 5). Often these symptoms are misinterpreted as nutrient deficiencies. The yield losses due to downy mildew disease alone are difficult to quantify because most of the time it is accompanied by white rust disease and viral diseases (Saharan et al., 2017), the yield loss due to the combined infection were reported to be around 23–55% (Saharan et al., 2017). Meena et al. (2016) reported yield losses of 11% (143.2 kg/ha) in Indian mustard plants in 2014 due to downy mildew. Disease severity also varies with the host plant.
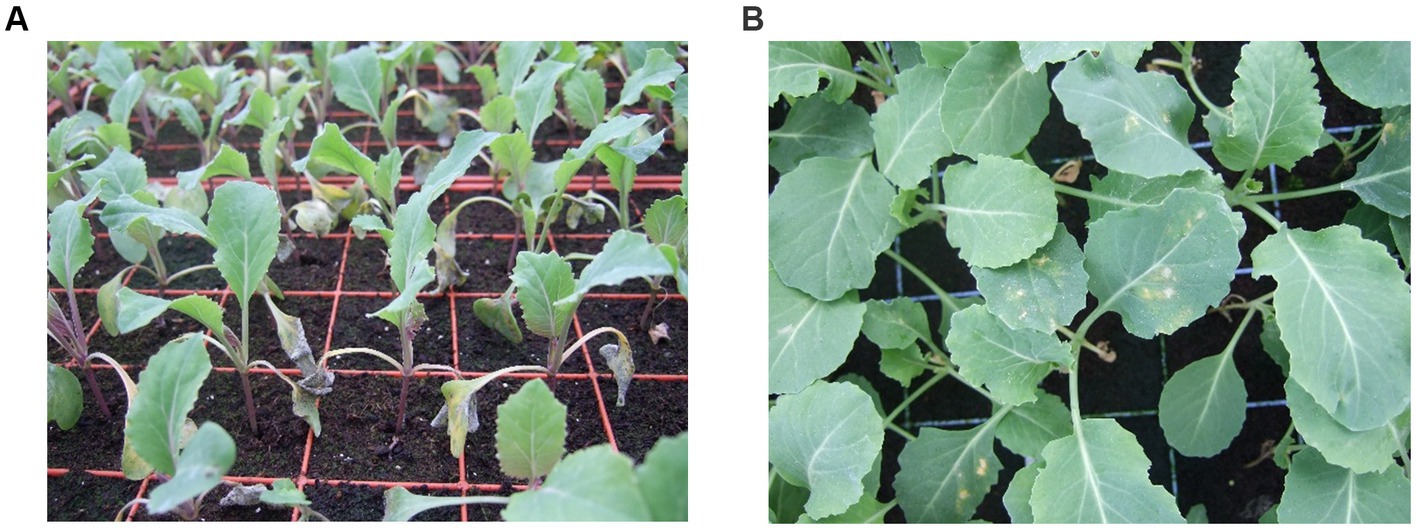
Figure 5. Symptoms of downy mildew infection caused by Hyaloperonospora parasitica on Brassica oleracea (photographs by Joana G. Vicente). (A) White powdery and wilted cotyledons of infected plants. (B) Yellow and white powdery lesions on the top side of older infected leaves associated with the veins.
4.2. Pathogen lifestyle and transmission
H. parastica is an obligate parasite of brassicas and has primary (sexual) and secondary (asexual) infection stages. Primary infection starts with the germination of sexually produced soilborne oospores in the presence of host plants and under conducive climatic conditions (Chang et al., 1963; Shiraushi et al., 1975). The oospores form germ tubes that penetrate the plant roots. Mycelium then form, growing intracellularly to produce haustoria that penetrate the cells for nutrient acquisition (Slusarenko and Schlaich, 2003). After 1–2 weeks, conidiophores grow out of stomata and release asexual conidiospores that are dispersed by wind and water splash to cause secondary infection of nearby hosts (Lucas et al., 1995). Conidiospores also produce germ tubes which penetrate the lower surface of the leaves (Chou, 1970; Achar, 1995) but sometimes infection can occur through the stomata. Concurrently, sexual oospores are produced in high number in the leaves, which then drop and infest the soil for further primary infection. As well as in the leaf, oospores have been found on the seed surface and in the pericarp and embryo. However, seed transmission has been shown to be extremely low 0.4–0.9% in rapeseed and mustard (Saharan et al., 2017) and so it is not considered an important route of transmission.
Generally, downy mildew on Brassica crops is more problematic when the temperature is between 10–15°C with high moisture conditions. However, the empirical data for disease development in the field is still lacking. Studies conducted in a controlled environment show that different developmental stages of the pathogen require different optima, conidial germination (8-12°C), cell penetration (16°C) and haustoria formation (20-24°C) (Chu, 1935; Felton and Walker, 1946). Oospores can survive for long periods (up to 5 years), without a host or during unfavorable conditions. Conversely, the survival as mycelium and conidial forms are greatly dependent on the environmental conditions (Saharan et al., 2017). Lin and Liang (1974) reported that conidia in the infected leaves of kohlrabi leaves survived for 10 days under warm field conditions and that the conidia could survive up to 110 days in a conducive environment. Marked reductions in the survival of conidia were observed in moist and dry soil in warm conditions (up to 22 days) (Krober, 1970).
4.3. Diagnostics
To date, diagnosing downy mildew mainly relies on observation and recognition of symptoms either by eye or using lenses (Slusarenko and Schlaich, 2003). The common serological methods for detection are ELISA and lateral flow assays. However, the major limitation of these methods is the cross-reactivity of antibodies with other oomycete species (Salcedo et al., 2021). While isoenzyme methodology can be used for diagnostics, this requires a large amount of pathogen material (Bonde et al., 1984). Moreover, these methods have been developed in other plant species and limited studies have been conducted in brassicas. The most reliable technique to detect H. parasitica is using molecular technologies. These methods detect the presence of the pathogen with a specific DNA marker, and includes approaches such as restriction fragment length polymorphism (RFLP), amplified fragment length polymorphism (AFLP), random amplified polymorphic DNA (RAPD), micro- and minisatellites (Schroeder et al., 2013; Wallace and Quesada-Ocampo, 2017). Although accurate, these are lab-based techniques, are relatively expensive requiring specific equipment and skilled personnel to interpret the results.
Recently, researchers have developed portable sequencers to enable in field detection of H. parasitica but they require further investigation to determine the sequencer’s sensitivity and reliability (DeShields et al., 2018; Salcedo et al., 2021). Additionally, high-resolution thermal and multi-spectral imagery has been used to detect downy mildew (Peronospora arborescens) in poppy fields (Landa et al., 2007). This technology could be translated to downy mildew detection in brassicas. However, these new diagnostic methods are only at the R&D level.
A huge knowledge gap exists in the epidemiology and forecasting of downy mildew. This is largely due to the complex life cycle of H. parasitica and that downy mildew is almost always present with other disease like white rust. Various forecasting models have been developed around the world but have not been robustly tested. For instance, a model developed in India gave a 21 and 36% deviation from predicted and actual downy mildew disease incidence in 1991–1992 and 1992–1993, respectively (Mehta and Saharan, 1999). The same model predicted a deviation of 66 and 16% in the same years for white rust. These large deviations for both diseases show that the model needs further improvement for best fit. Often a prediction model developed for one location might not fit another location. Hence, the model must be tested in multiple locations and for longer periods as well as consider microclimate, H. parasitica pathotype and crop cultivar to develop an effective tool (Mehta, 2014).
4.4. Prevention and management
4.4.1. Farm practices
One of the greatest challenges in controlling downy mildew is that it has a very short latent period. Raising disease-free seedlings is considered one of the crucial steps in downy mildew prevention and management. Good hygiene practices include sterilizing the glasshouse between each crop and raising plants in plastic or concrete platforms to avoid contact with the soil. To prevent secondary infection, it is recommended to remove cruciferous weeds from the field as they can act as an inoculum source. Infected plants and debris should also be removed quickly and destroyed to prevent contaminating the soil with long-lasting oospores which can lead to primary infections (RHS, 2023). In addition, it is recommended to avoid overhead irrigation systems, maintain well-drained soil, have long crop rotations (minimally two years), reduce planting density to reduce the relative humidity and water in the early morning to enable adequate time to dry to reduce the dispersal of spores (Sherf and MacNab, 1986). Koul and Singh (1999) showed that the removal of 50% of lower leaves within 60 days of the crop age reduced disease severity as it helped to reduce the relative humidity around the plants. They also showed that early sowing reduced disease severity in B. juncea grown in India because the plants matured before the pathogen could reach epidemic form.
4.4.2. Chemical control
Although not a fungus, downy mildew disease is primarily controlled by fungicide treatments like metalaxyl. Resistance to metalaxyl was reported in H. parasitica within 40 years of its introduction (Crute et al., 1985; Crute and Pink, 1996). Despite this, metalaxyl is still used for the control of downy mildew. Seed treatment with Apron (mixture of metalaxyl and captan) at the rate of 2 g/Kg has been shown to give 96% disease control in cauliflower and generally increased yield when used in combination with 1–2 foliar sprays (Verma and Thakur, 1989). White et al. (1984) showed that cauliflowers seed-treated with Apron did not develop disease symptoms, even after the artificial inoculation. However, metalaxyl is persistent and was present in cabbage leaves up to 4 weeks after sowing from treated seeds (Crute et al., 1985). An effective and economical schedule for the application of seed treatment and/or foliar sprays must be tailored for each local condition and crop. For instance, with mustard plants grown in India it is recommended to treat seed with Apron followed by three foliar sprays at 30 days intervals to control downy mildew (Koul and Singh, 1999). Since sporulation of the pathogen occurs mainly at the night and is disseminated during the morning, it is recommended to apply fungicides at the end of the day (AHDB, 2020).
Apart from seed treatment and foliar sprays, fungicides are also applied to the soil before sowing. The application of fungicides such as prothiocarb and fosetyl-aluminium as a soil drench with 50% seed emergence has controlled the disease progression in cauliflower and radish plants (Saharan et al., 2017). The application of granular metalaxyl pre-sowing also exhibited promising results on broccoli (0.56 a.i/ha) and cauliflower (0.28 a.i/ha) (Chiu, 1959). Compost treatment is also effective in controlling downy mildew disease, in the UK, module-raised cauliflower plants are grown in compost containing metalaxyl, milfuran with manganese zinc dithiocarbamate or propamocarb to control downy mildew. Although fungicides are effective at controlling downy mildew, they have their disadvantages (1) they persist in plants and will have associated toxicity (2) emergence of pathogen resistance (3) costly production and application processes as well as potential unknown environmental impacts.
Biochemicals such as salicylic acid and its derivatives can activate systemic acquired resistance in the host (Wang et al., 2007; Collier et al., 2011). Promising results were observed when S-methyl benzo [1,2,3] thiadiazole-7-carbothioate (a derivative of salicylic acid) was used to treat seeds of B. oleracea against H. parasitica (Molina et al., 1998). These biochemicals have a different mode of action compared to the conventional chemical control agents and when used as part of an integrated pest management strategy, would result in more durable control of downy mildew disease in Brassica crops (Jensen et al., 1998). However, further evaluation is required to determine the longevity and effectiveness of biochemicals for field use.
4.4.3. Biological control
Trichoderma harzianum and Pseudomonas spp. are the most common biocontrol agents for downy mildew in Brassica crops. Both have been shown to have a similar ability to reduce downy mildew disease severity in B. juncea when used as seed treatments and foliar sprays, singularly and in combination (Meena et al., 2013). In the same study, it was shown that a seed treatment with aqueous garlic extract followed by foliar sprays significantly reduced downy mildew disease incidence and severity (Meena et al., 2013). Garlic juice and aqueous extract of garlic have also been reported to inhibit H. parasitica in radish plants (Bedlan, 1987). Additionally, avirulent stains of H. parasitica have been shown to induce downy mildew resistance in broccoli and cauliflower (Singh et al., 2002). Although promising, further research is required to see how applicable avirulent strains would be in a field setting. One potential risk is that they may exchange genetic material (e.g., through horizontal gene transfer) with pre-existing isolates in the field and as a result become virulent, thus exacerbating the problem rather than remedying it.
4.4.4. Resistant cultivars
Compared to other diseases discussed in this review, downy mildew can sexually reproduce meaning that host resistance can be more easily overcome (Saharan et al., 2017). There are six pathotypes of H. parasitica which pose another challenge for resistance breeding (Coelho et al., 2012; Vicente et al., 2012). Fully and partially resistant varieties are available on the market or are under development (Ridout, 2018; Table 1), these are mainly broccoli types and there is limited or no resistance in Brussels sprouts, cauliflower and cabbage. Single gene resistance has been identified in various B. oleracea cvs. such as cauliflower, broccoli, kale and Brussels sprouts (Hoser-Krauze et al., 1984; Farnham et al., 2002; Carlsson et al., 2004) and also multi-gene resistances in in cauliflower, broccoli and cabbage (Jensen et al., 1999; Moss, 2002). However, they are race and geographic specific. Although downy mildew is considered one of the most devasting brassica diseases little research has been conducted to explore the development of resistance cultivars.
5. Turnip yellows virus
5.1. Host and impact
Turnip yellows virus (TuYV) syn. beet western yellows virus (BWYV) is a positive-sense, single-stranded RNA virus belonging to the genus Polerovirus in the family Solemoviridae (previously Luteoviridae, Scheets et al., 2020). BWYV was first reported in the USA in the 1950s as causing stunting and chlorosis of a broad range of plant species including sugar beet, brassicas and lettuce (Duffus, 1960). In the 1960s, BWYV-like isolates were reported in the UK that were serologically similar but not able to infect sugar beet (Russell and Duffus, 1970). These non-beet infecting isolates were reclassified as TuYV (Mayo, 2002). Subsequent molecular and sequencing analyses supports BWYV and TuYV as being different species (Hauser et al., 2000; Stevens et al., 2005).
TuYV has a broad host range infecting species from more than 16 plant families (Stevens et al., 2008). It is one of the main viruses infecting vegetable and oilseed brassicas and has been reported in 26 countries and 6 continents (Greer et al., 2021b; CABI, 2022a), but it is likely an underrepresentation and TuYV is probably present in other brassicas growing regions but not reported. TuYV infection can cause a range of non-specific symptoms in brassicas such as chlorosis, purpling and reddening of the leaf margins and stunting (Figure 6) but often infection can be symptomless. In oilseed brassicas, yield losses of 12–46% due to TuYV infection have been reported (Graichen and Schliephake, 1999; Jones et al., 2007; Nicholls and Stoddart, 2014) and in vegetable brassicas like Brussels sprouts, the virus can cause losses as high as 65% (Walsh et al., 2011). Furthermore, TuYV infection has been associated with tipburn, an internal disorder of stored white cabbage that can seriously impact post-harvest quality (Hunter et al., 2002).
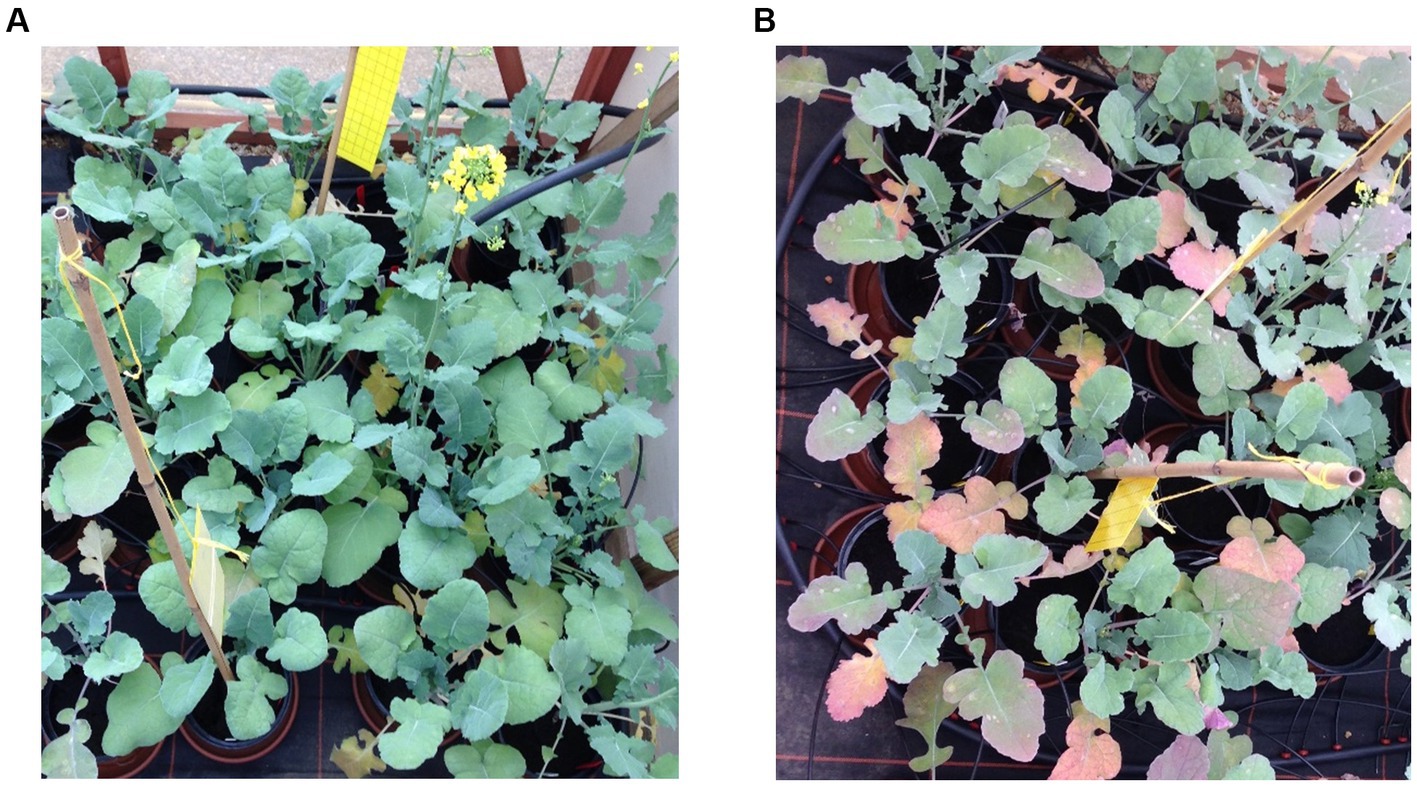
Figure 6. Symptoms of turnip yellows virus (TuYV) infection in oilseed rape (Brassica napus). (A) Healthy oilseed rape plants not infected with TuYV. (B) Oilseed rape plants infected with TuYV showing redding and purpling of the leaves.
5.2. Pathogen lifestyle and transmission
TuYV is transmitted by aphids in a persistent, circulative and non-propagative manner (Coleman, 2013). This means that once an aphid ingests TuYV from feeding on an infected plant, it carries and can transmit the virus for life, but the virus does not replicate within the aphid or pass to its progeny. Schliephake et al. (2000) showed that 17 species of aphid can transmit TuYV and that Myzus persicae (green peach-potato aphid) was the most efficient vector. In a study at Broom’s Barn Research Center, UK between 1994 and 2002, 72% of M. persicae caught in water traps tested positive for TuYV (Stevens et al. 2008). Furthermore, TuYV incidence in oilseed rape crops has been shown to be closely linked with the number of migrating M. persicae (Asare-Bediako et al., 2020). Thus, years with increased aphid numbers saw higher TuYV incidence, sometimes as high as 100%. There is also some evidence to suggest that TuYV infected plants release chemical volatiles that attract M. persciae to promote further transmission of the virus (Claudel et al., 2018).
Due to the highly polyphagous nature of M. persicae, TuYV can persist in weed species outside of the brassica growing season. However, Newbert (2016) showed that while some weed isolates were able to infect oilseed rape, others were unable to and were genetically distinct from crop isolates. TuYV is a phloem limited virus and relies on aphid vectors for transmission. It cannot be transmitted by contact, mechanically (through wounding) or through the seed like other plant viruses.
5.3. Diagnostics
Diagnosis of TuYV infection of brassicas by visual symptoms alone is challenging as they are not always apparent and when present are non-specific and can easily be mistaken for other phenotypes such as nutrient deficiency. Serological techniques such as ELISA using commercially available antibodies can be used to test plant tissue (D’Arcy et al., 1989; Greer et al., 2021a) and molecular techniques such as RT-PCR and LAMP can be used to detect TuYV in plants and aphids (Newbert, 2016; Congdon et al., 2019). Plant material is often not tested for TuYV as once the virus is present in the crop there is little that can be done to control/remove it. It is more informative to test aphids for TuYV, as these results can be fed into forecasting tools. The Department of Primary Industries and Regional Development, Government of Western Australia have developed such a tool that informs growers of aphid and TuYV pressure so that they can tailor their prevention and management practices accordingly (Congdon, 2021).
5.4. Prevention and management
5.4.1. Farm practices
Management of aphids is key for the control of TuYV. Monitoring tools can be used to assess aphid activity and help time the drilling and transplanting of brassicas to the field after the peak aphid migrations, where transmission of TuYV will be lower. This also minimizes early infection of the crop which is generally more detrimental than late infection (Congdon et al., 2020). Further, it is also important to remove weeds and manage green-bridges where aphids may find an alternative host and reservoir the virus between crop rotations (AHDB, 2023b). For similar reasons, brassicas should not be grown in fields near other brassica crops or alternative hosts.
5.4.2. Chemical control
Viruses cannot be directly targeted by chemical sprays or seed treatments like other phytopathogens (bacteria and fungi), instead chemicals target the viral vector. Unfortunately, the primary vector of TuYV, M. persicae has evolved resistance mechanisms to many of the available insecticide treatments (Bass et al., 2014). Most of the M. persicae population possess modified acetylcholinesterase (MACE), knockdown (kdr) or super-kdr resistance that render organophosphates, carbamates and pyrethroid insecticides ineffective (IRAG, 2021).
Insecticides belonging to the pyridine azomethines, sulfoximines and neonicotinoids still remain effective against M. persicae, although resistance to the latter has already been reported in Southern mainland Europe, North Africa and Australia (Slater et al., 2012; de Little et al., 2017; Charaabi et al., 2018). To maintain their effectiveness, it is imperative to rotate insecticides belonging to different chemical groups and apply in an informed, and restricted, manner. For example, using seed treatments and foliar sprays early in the growing period, where TuYV infection has its greatest yield impact (Congdon et al., 2020) could be the more effective and more resource efficient than applying treatments throughout the entire growing season. Furthermore, forecasting tools can be used to inform the timings of chemical applications to provide maximum protection, e.g., to coincide with high aphid numbers. It is important to note that some of the effective insecticides may not be approved for use in a given country and pose another hurdle for the control of TuYV. For example, neonicotinoids were banned for use in the EU in 2013 as they were found to be detrimental to pollinator species (The European Commission, 2013).
5.4.3. Biological control
Like chemical controls, biological controls target the aphid vector. There are over 150 natural enemies (pathogen, parasite or predator) of M. persicae but most are not used on brassica crops (CABI, 2022c). India uses several predatory species of hoverflies like Sphaerophoria indiana, Ischiodon scutellaris, Eupeodes latilunulatus and E. confrater to control M. persicae in mustard (B. nigra and B. juncea). However, these species are only found in India or countries of the Asia-Pacific (CABI, 2022c). Conversely, Lecanicillium muscarium and Beauveria bassiana are widespread entomopathogenic fungi. They are approved for use in Europe, Asia and America to control whitefly, thrips and aphids like M. persicae in vegetable crops (Lewis et al., 2016). Another recent study by Paliwal et al. (2022) identified entomopathogenic bacterial species that killed different clones of M. persicae but were non-pathogenic to plants. This is a promising finding but will require further research and development before it could be marketable.
RNA interference (RNAi) has the potential for the control of TuYV. RNAi is a natural gene regulatory mechanism in eukaryotes. It involves the sequence-specific recognition of single- and double-stranded RNA and either tags them for degradation or prevents their translation. The TuYV genome comprises of a single stranded RNA molecule, and it produces double-stranded RNA intermediates during its replication, making it a potential target for RNAi. This has been achieved for other viruses, Lindbo and Dougherty (1992) engineered tobacco plants to express a modified non-functional coat protein of the Tobacco Etch Virus which in turn induced resistance. In a similar way, resistance to M. persicae was achieved in tobacco by engineering it to express aphid gene fragments (Feng and Jander, 2022). However, RNAi would require approval as it is a genetic engineering/modification technique.
5.4.4. Resistant cultivars
Host resistance to TuYV is one of the only ways to directly control the virus. Several TuYV resistances have been identified, mainly in B. napus (Thomas et al., 1990; Coutts et al., 2010) but only one, the ‘R54’ resistance has been deployed commercially. There are numerous TuYV-resistant oilseed rape varieties but there are no vegetable brassica resistant varieties, although some claim generalized virus resistance (Table 1). The ‘R54’ resistance originated from B. napus and was first reported by Graichen (1994). It has subsequently been characterized and mapped to a single dominant QTL on chr. A04 (B. rapa A genome) (Dreyer et al., 2001; Juergens et al., 2010). Another resistance from oilseed rape line ‘Yudal’ was mapped to the same genetic locus as the ‘R54’ resistance, but further marker analyses suggested that they may be different in origin (Hackenberg et al., 2020). A study by Congdon et al. (2021) identified additional TuYV resistance source in B. napus, B. oleracea, and B. rapa and showed that at higher temperatures these resistance could breakdown. This is important to consider as some resistances may not be effective in countries with warmer climates or more temperate countries in the face of climate change. Further work is also needed to assess whether the identified resistances are effective against genetically diverse TuYV isolates (Newbert, 2016). Lastly, to ensure the durability of TuYV resistances they should be stacked to reduce the selection pressure for resistance-breaking isolates of TuYV. This was achieved by Greer et al. (2021a) who stacked TuYV resistances from B. rapa (genome AA) and B. oleracea (genome CC) in B. napus (genome AACC).
Another angle for the control of TuYV is the use of Brassica cultivars resistant to M. persicae but none have been reported. However, resistance to M. persicae has been reported in other hosts like peach and the genes responsible Rm1, Rm2, and Rm3 (Lambert and Pascal, 2011; Pascal et al., 2017; Niu et al., 2018) have been cloned and perhaps could be integrated in Brassica crops though genetic modification techniques. However, while some of the resistances are extreme it only takes one aphid and short feeding time of just 15 min to transmit TuYV (Stevens et al., 2008). Thus, host resistance to aphids alone may not provide adequate protection against TuYV in brassica.
6. Summary and future perspectives
Black rot (bacterial), clubroot (protist), downy mildew (oomycete) and aphid transmitted turnip yellows virus (aphid and viral) represent vastly different classes of pests and pathogens that cause considerable yield losses on brassicas worldwide. They have complex control strategies where no single preventative or management method discussed in this review is effective on its own. It is therefore important to develop IPMs that combine multiple control methods to reduce pests to tolerable levels with minimal effect on the environment and minimal costs to the pest manager. The five pillars of IPM are (1) identification of pest, host, and beneficial microbes (2) developing monitoring guidelines that are species specific (3) determine action thresholds for the pest (4) evaluate and implement control strategies and finally (5) documenting the results. Although, developing an IPM strategy is a long-term process that requires time, resource, and energy it is the most efficient strategy to control diseases of Brassica.
Many of the diagnostics identified in this review are implemented post-disease establishment and by this time, farmers and grower have identified the problem and do not need confirmational diagnostics. Many are also specialized and are lab-based (PCR, ELISA, FAME etc.). Diagnostics will be useful for testing farm-saved seed and identifying prevalent pathotypes/races of a pathogen for resistance cultivar deployment, but they need to be rapid and in-field. Another major limitation of diagnostics is that there is still a huge knowledge gap in pathogen biology/lifecycle, that in turn impedes disease detection and monitoring. Several IOT tool have been developed for disease forecasting, detection, monitoring and management but these are often generalist, e.g., for bacterial disease of brassicas. Specialist host-pathogen specific IOT tools need to be developed.
There are several practices, mainly cultural that are applicable for control of all the diseases discussed in this review (Table 3), but others are disease specific (chemical, biocontrol, host resistance). Cultural controls like seed sterilization and sanitation of field and glasshouse equipment are generally cheap and easy to implement. Removal of weeds could be helpful in some instances, but there is evidence to suggest that weed isolates can be distinct from those infecting crops and there is a balance between crop protection and maintaining biodiversity. Timing of drilling or transplanting of Brassica crops may be beneficial to prevent one disease but may exacerbate another. For example, early planting of brassicas reduces clubroot severity, but early sowing and infection increases yield losses to TuYV (Gossen et al., 2012; Congdon et al., 2020).
Chemical controls are damaging to the environment and generally not effective because of evolving resistance mechanisms brought about by over dependence and misuse/overuse of single products. Furthermore, the active chemistry (mode of action) used may not be specific to the pathogen and therefore have limited effectiveness, e.g., fungicides are used to control the clubroot and downy mildew pathogens which are protists and oomycetes, respectively. There are also no chemicals that directly target viruses. Forecasting can help inform precise chemical applications which will in turn maximize their effectiveness, reduce environmental impact and ensure their longevity.
Biocontrols are promising and are generally more precise than chemical applications which can kill beneficial microbiota. However, very few biocontrol agents are currently available for Brassica pests and diseases. Most are in the research stages and require considerably more development and time to deliver to market. Some may also meet consumer resistance and will be subject to local laws and regulations due to them being, gene-editing/−modification techniques or non-native species.
Numerous resistance sources to each of the diseases discussed here have been identified, characterized, and mapped in Brassica but very few have reached the stage where they have been deployed in the field. A limited resistance base causes strong selection pressure for resistance breaking isolates and so there is an increased risk of resistance break down. Ideally, multiple resistances for each pathogen, pathotype and race would be stacked, or rotated to prevent this. However, resistance often comes with a yield penalty of its own. Introgression of resistance into commercial crop types can take >10 years using traditional breeding methods for even simple single gene resistance. This can be accelerated using gene-editing/−modification techniques, although like biocontrol, this would be subject to local laws and regulations. Rapid in-field diagnostics could help identify pathotypes and races prevalent in the field which would help inform which resistances to deploy and help prevent resistance break down.
Although not discussed in depth in this review, it is also important to consider that disease severity and yield losses caused by disease can be heavily influenced by abiotic factors (weather, nutrient availability, soil pH etc.). For example, calcium and boron levels in the soil play a significant role in clubroot disease management (Saharan et al., 2021). How abiotic factors influence diseases of brassicas and their management is still not clear. Similarly, it is not clear how pathogens interact with other pathogens or beneficial microbes in a field setting as traditional plant pathology is focused on the study of single pathogen-plant host systems. There are still several knowledge gaps that need to be addressed for successful control of the Brassica diseases discussed in this review. These are outlined in Table 4 along with our future recommendations for disease prevention and management.
Author contributions
SG and AS: conceptualization, methodology, and writing—original draft. MG and RL: supervision, validation, and writing—reviewing and editing. All authors contributed to the article and approved the submitted version.
Funding
This work was funded by a grant from BBSRC, NERC, Defra, and the Scottish Government, under the Strategic Priorities Fund Plant Bacterial Diseases program under the project ‘Xanthomonas plants diseases: mitigating existing, emerging and future threats to UK agriculture’ (BB/T010924/1) and a grant from Innovate UK under the project ‘Insectrial Revolution’ (ISCF FFPS 47278). The Open Access publication costs were provided by the UKRI block grant to the University of Warwick.
Acknowledgments
The authors thank Srayan Ghosh, John Walsh, and Joana G. Vicente from the University of Warwick for the provision of photographs of disease symptoms for black rot, clubroot and downy mildew, respectively. The authors would also like to thank Angela Hambidge, University of Warwick for her assistance with Endnote.
Conflict of interest
The authors declare that the research was conducted in the absence of any commercial or financial relationships that could be construed as a potential conflict of interest.
Publisher’s note
All claims expressed in this article are solely those of the authors and do not necessarily represent those of their affiliated organizations, or those of the publisher, the editors and the reviewers. Any product that may be evaluated in this article, or claim that may be made by its manufacturer, is not guaranteed or endorsed by the publisher.
References
Achar, P. (1995). Tissue culture technique to determine the viability of Peronospora parasitica in Brassica oleracea. J. Phytopathol. 143, 647–649. doi: 10.1111/j.1439-0434.1995.tb00217.x
Adhikari, K. K. (2010). Effect of temperature, biofungicides and fungicides on clubroot of selected Brassica crops. Guelph: University of Guelph.
AHDB (2020). Brassica diseases [online]. Available at: https://projectbluearchive.blob.core.windows.net/media/Default/Horticulture/BrassicaDiseasesGuide1719_200428_WEB.pdf (Accessed April 19, 2023)
AHDB (2023a). Black rot of brassicas: Prevention and control [online]. Available at: https://horticulture.ahdb.org.uk/knowledge-library/black-rot-of-brassicas-prevention-and-control (Accessed March 08, 2023)
AHDB (2023b). Turnip yellow virus (TuYV) management in oilseed rape [online]. Available at: https://ahdb.org.uk/knowledge-library/turnip-yellow-virus-tuyv-management-in-oilseed-rape (Accessed April 03, 2023)
Ahmed, H. U., Hwang, S. F., Strelkov, S. E., Gossen, B. D., Peng, G., Howard, R. J., et al. (2011). Assessment of bait crops to reduce inoculum of clubroot (Plasmodiophora brassicae) of canola. Can. J. Plant Sci. 91, 545–551. doi: 10.4141/cjps10200
Alvarez, A. (1994). Serological, pathological, and genetic diversity among strains of Xanthomonas campestris infecting crucifers. Phytopathology 84, 1449–1457. doi: 10.1094/Phyto-84-1449
An, S. Q., Potnis, N., Dow, M., Vorhölter, F. J., He, Y. Q., Becker, A., et al. (2020). Mechanistic insights into host adaptation, virulence and epidemiology of the phytopathogen Xanthomonas. FEMS Microbiol. Rev. 44, 1–32. doi: 10.1093/femsre/fuz024
Asare-Bediako, E., Jones, J. E., Hambidge, A. J., Stevens, M., Mead, A., Jenner, C. E., et al. (2020). The incidence of turnip yellows virus in oilseed rape crops (Brassica napus L.) in three different regions of England over three consecutive growing seasons and the relationship with the abundance of flying Myzus persicae. Ann. Appl. Biol. 176, 130–137. doi: 10.1111/aab.12565
Bass, C., Puinean, A. M., Zimmer, C. T., Denholm, I., Field, L. M., Foster, S. P., et al. (2014). The evolution of insecticide resistance in the peach potato aphid, Myzus persicae. Insect Biochem. Mol. Biol. 51, 41–51. doi: 10.1016/j.ibmb.2014.05.003
Berg, T., Tesoriero, L. A., and Hailstones, D. (2005). PCR-based detection of Xanthomonas campestris pathovars in Brassica seed. Plant Pathol. 54, 416–427. doi: 10.1111/j.1365-3059.2005.01186.x
Bergey, D. H. F. C., Harrison, R. S., Breed, B. W., and Huntoon, F. M.. (1923). Manual of Determinative Bacteriology. 1st edition, Baltimore, The Williams & Wilkins Co.
Berry, P. M., and Spink, J. H. (2006). A physiological analysis of oilseed rape yields: past and future. J. Agric. Sci. 144, 381–392. doi: 10.1017/S0021859606006423
Bhat, N. A., and Masoodi, S. D. (2000). Efficacy of various antibiotics against Xanthomonas campestris pv. Campestris the casual pathogen of black rot of cabbage. Appl. Biol. Res. 2, 161–163.
Bhattacharya, I., Dutta, S., Mondal, S., and Mondal, B. (2014). Clubroot disease on Brassica crops in India. Can. J. Plant Pathol. 36, 154–160. doi: 10.1080/07060661.2013.875064
Bochow, H. (1963). Untersuchungen zur Ökologie und indirekten Bekämpfung von Plasmodiophora brassicae Wor: ein Beitrag zur Klärung von Fragen der Bodenhygiene. The University Rostock-thesis.
Bochow, H. (1965). The effect of root diffusates of host and non-host plants on the resting spore germination of Plasmodiophora brassicae Wor. Prague: Czechoslovak Academy of Sciences.
Bonde, M. R., Peterson, G. L., Dowler, W. M., and May, B. (1984). Isozyme analysis to differentiate species of Peronosclerospora causing downy mildews of maize. Phytopathology 74, 1278–1283. doi: 10.1094/Phyto-74-1278
Bonini, M., Maringoni, A., and Neto, J. (2007). Characterization of Xanthomonas spp. strains by bacteriocins. Summa Phytopathol. 33, 24–29. doi: 10.1590/S0100-54052007000100003
Brown, J. E., Lu, T. Y., Stevens, C., Khan, V. A., Lu, J. Y., Wilson, C. L., et al. (2001). The effect of low dose ultraviolet light-C seed treatment on induced resistance in cabbage to black rot (Xanthomonas campestris pv. Campestris). Crop Prot. 20, 873–883. doi: 10.1016/S0261-2194(01)00037-0
Burnett, F. J., Boor, T., Dussart, F., and Smith, J. (2019). Developing sustainable management methods for clubroot. Agriculture and Horticulture Development Board pr608 Final Project Report, 1–75.
CABI (2022a). Beet western yellows virus (turnip (mild) yellows). CABI Int. doi: 10.1079/pwkb.species.10259
CABI (2022b). Hyaloperonospora parasitica (downy mildew). CABI Compendium. doi: 10.1079/cabicompendium.39723
CABI (2022c). Myzus persicae (green peach aphid). CABI Compendium. doi: 10.1079/cabicompendium.35642
CABI (2022d). Xanthomonas campestris pv. Campestris (black rot). CABI Int. doi: 10.1079/pwkb.species.56919
Carlsson, M., Von Bothmer, R., and Merker, A. (2004). Screening and evaluation of resistance to downy mildew (Peronospora parasitica) and clubroot (Plasmodiophora brassicae) in genetic resources of Brassica oleracea. Hereditas 141, 293–300. doi: 10.1111/j.1601-5223.2004.01818.x
Chai, A. L., Xie, X. W., Shi, Y. X., and Li, B. J. (2014). Research status of clubroot (Plasmodiophora brassicae) on cruciferous crops in China. Can. J. Plant Pathol. 36, 142–153. doi: 10.1080/07060661.2013.868829
Chang, I. H., Xu, R. F., and Chiu, W. F. (1963). On the primary sources of infection of the downy mildew of Chinese cabbage caused by Peronospora parasitica (Pers.) Fr. And the limited systemic infection of seedlings. Acta Phytopathologica Sinica 6, 153–162.
Charaabi, K., Boukhris-Bouhachem, S., Makni, M., and Denholm, I. (2018). Occurrence of target-site resistance to neonicotinoids in the aphid Myzus persicae in Tunisia, and its status on different host plants. Pest Manag. Sci. 74, 1297–1301. doi: 10.1002/ps.4833
Chiu, W. F. (1959). On problems of three destructive diseases of the Chinese cabbage. Bullet. Agric. Sci. 9, 314–315.
Chou, C. K. (1970). An electron-microscope study of host penetration and early stages of haustorium formation of Peronospora parasitica (Fr.) Tul. on cabbage cotyledons. Ann. Bot. 34, 189–204. doi: 10.1093/oxfordjournals.aob.a084352
Chu, H. T. (1935). Notes on the penetration phenomena and haustorium formation in Peronospora brassicae Gäum. Japan J. Phytopathol. 5, 150–157. doi: 10.3186/jjphytopath.5.150
Chupp, C. (1934). A translation of Plasmodiophora brassicae–the cause of cabbage hernia by MS Woronin 1878, Phytopathological classics no. 4. St. Paul, Minnesota: American Phytopathological Society.
Claudel, P., Chesnais, Q., Fouche, Q., Krieger, C., Halter, D., Bogaert, F., et al. (2018). The aphid-transmitted turnip yellows virus differentially affects volatiles emission and subsequent vector behavior in two Brassicaceae plants. Int. J. Mol. Sci. 19:2316. doi: 10.3390/ijms19082316
Coelho, P. S., Vicente, J. G., Monteiro, A. A., and Holub, E. B. (2012). Pathotypic diversity of Hyaloperonospora brassicae collected from Brassica oleracea. Eur. J. Plant Pathol. 134, 763–771. doi: 10.1007/s10658-012-0052-z
Coleman, A. D. (2013). Control of turnip yellows virus: assessing impact on oilseed rape quality traits and dissecting circulative transmission by aphids. Res. Rev. 26, 1–50.
Collier, S. M., Hamel, L.-P., and Moffett, P. (2011). Cell death mediated by the N-terminal domains of a unique and highly conserved class of NB-LRR protein. Mol. Plant-Microbe Interact. 24, 918–931. doi: 10.1094/MPMI-03-11-0050
Congdon, B. (2021). Turnip yellows virus early warning system [online]. Available at: https://www.agric.wa.gov.au/canola/turnip-yellows-virus-early-warning-system#:~:text=DPIRD%20has%20developed%20an%20early%20warning%20system%20for,and%202021%20in%20Victoria%20and%20New%20South%20Wales
Congdon, B. S., Baulch, J. R., and Coutts, B. A. (2020). Impact of turnip yellows virus infection on seed yield of an open-pollinated and hybrid canola cultivar when inoculated at different growth stages. Virus Res. 277:197847. doi: 10.1016/j.virusres.2019.197847
Congdon, B. S., Baulch, J. R., and Coutts, B. A. (2021). Novel sources of turnip yellows virus resistance in Brassica and impacts of temperature on their durability. Plant Dis. 105, 2484–2493. doi: 10.1094/PDIS-10-20-2312-RE
Congdon, B. S., Kehoe, M. A., Filardo, F. F., and Coutts, B. A. (2019). In-field capable loop-mediated isothermal amplification detection of turnip yellows virus in plants and its principal aphid vector Myzus persicae. J. Virol. Methods 265, 15–21. doi: 10.1016/j.jviromet.2018.12.014
CornellCALS (2022). Disease resistant vegetable varieties [Online]. Available at: https://www.vegetables.cornell.edu/pest-management/disease-factsheets/disease-resistant-vegetable-varieties/ (Accessed August 16, 2023)
Coutts, B. A., Webster, C. G., and Jones, R. A. C. (2010). Control of beet western yellows virus in Brassica napus crops: infection resistance in Australian genotypes and effectiveness of imidacloprid seed dressing. Crop Pasture Sci. 61, 321–330. doi: 10.1071/CP09264
Craig, M. A. (1989). Resting spores of Plasmodiophora brassicae as affected by root exudates of hosts and nonhosts. Glasgow: University of Strathclyde.
Crute, I. R., Norwood, J. M., and Gordon, P. L. (1985). "Resistance to phenylamide fungicides in lettuce and brassica downy mildew", In: Proceedings of the Mixture Centenary Meeting, Bordeaux, British Crop Protection Council Monograph, 311–314.
Crute, I. R., and Pink, D. A. C. (1996). Genetics and utilization of pathogen resistance in plants. Plant Cell 8, 1747–1755. doi: 10.2307/3870227
D’arcy, C. J., Torrance, L., and Martin, R. R. (1989). Discrimination among luteoviruses and their strains by monoclonal antibodies and identification of common epitopes. Phytopathology 79, 869–873. doi: 10.1094/Phyto-79-869
Daw, M. A., and Falkiner, F. R. (1996). Bacteriocins: nature, function and structure. Micron 27, 467–479. doi: 10.1016/S0968-4328(96)00028-5
De Little, S. C., Edwards, O., Van Rooyen, A. R., Weeks, A., and Umina, P. A. (2017). Discovery of metabolic resistance to neonicotinoids in green peach aphids (Myzus persicae) in Australia. Pest Manag. Sci. 73, 1611–1617. doi: 10.1002/ps.4495
De Paiva, B. A. R., Wendland, A., Teixeira, N. C., and Ferreira, M. A. S. V. (2019). Rapid detection of Xanthomonas citri pv. Fuscans and Xanthomonas phaseoli pv. Phaseoli in common bean by loop-mediated isothermal amplification. Plant Dis. 104, 198–203. doi: 10.1094/PDIS-02-19-0325-RE
Deshields, J. B., Bomberger, R. A., Woodhall, J. W., Wheeler, D. L., Moroz, N., Johnson, D. A., et al. (2018). On-site molecular detection of soil-borne phytopathogens using a portable real-time PCR system. J. Vis. Exp. :e56891. doi: 10.3791/56891-v
Dixon, G. R. (2009). The occurrence and economic impact of Plasmodiophora brassicae and clubroot disease. J. Plant Growth Regul. 28, 194–202. doi: 10.1007/s00344-009-9090-y
Donald, E. C., and Porter, I. J. (2014). Clubroot in Australia: the history and impact of Plasmodiophora brassicae in Brassica crops and research efforts directed towards its control. Can. J. Plant Pathol. 36, 66–84. doi: 10.1080/07060661.2013.873482
Dong, H., Xiong, R., Liang, Y., Tang, G., Yang, J., Tang, J., et al. (2020). Development of glycine-copper(ii) hydroxide nanoparticles with improved biosafety for sustainable plant disease management. RSC Adv. 10, 21222–21227. doi: 10.1039/D0RA02050H
Dowson, W. J. (1939). “On the systematic position and generic names of the Gram-negative bacterial plant pathogens.” Zentralblatt für Bakteriologie, Parasitenkunde, Infektionskrankheiten und Hygiene, Abteilung II, 100, 177–193.
Dreyer, F., Graichen, K., and Jung, C. (2001). A major quantitative trait locus for resistance to turnip yellows virus (TuYV, syn. Beet western yellows virus, BWYV) in rapeseed. Plant Breed. 120, 457–462. doi: 10.1046/j.1439-0523.2001.00646.x
Duffus, J. E. (1960). Radish yellows, a disease of radish, sugar beet, and other crops. Phytopathology 50, 389–394.
FAO (2023a). FAOSTAT – worldwide production and yield of cabbage, cauliflower, broccoli and rape or colza seed [online]. Available at: https://www.fao.org/faostat/en/#data/QCL (Accessed March 07, 2023)
FAO (2023b). Crop information on cabbage. [online]. Available at: https://www.fao.org/land-water/databases-and-software/crop-information/cabbage/en/ (Accessed March 08, 2023)
FAO (2023c). FAOSTAT – worldwide production value of cabbage, cauliflower, broccoli and rape or colza seed [online]. Available at: https://www.fao.org/faostat/en/#data/QV (Accessed March 07, 2023)
Farnham, M. W., Wang, M., and Thomas, C. E. (2002). A single dominant gene for downy mildew resistance in broccoli. Euphytica 128, 405–407. doi: 10.1023/A:1021279426050
Felton, M. W., and Walker, J. C. (1946). Environal factors affecting downy mildew of cabbage. J. Agric. Res. 72, 69–81.
Feng, J., Hwang, R., Hwang, S. F., Strelkov, S. E., Gossen, B. D., Zhou, Q. X., et al. (2010). Molecular characterization of a serine protease Pro1 from Plasmodiophora brassicae that stimulates resting spore germination. Mol. Plant Pathol. 11, 503–512. doi: 10.1111/j.1364-3703.2010.00623.x
Feng, J., Hwang, S. F., and Strelkov, S. E. (2013). Studies into primary and secondary infection processes by Plasmodiophora brassicae on canola. Plant Pathol. 62, 177–183. doi: 10.1111/j.1365-3059.2012.02612.x
Feng, H., and Jander, G. (2022). “Rapid screening of Myzus persicae (green peach aphid) RNAi targets using tobacco rattle virus” in RNAi strategies for Pest management: Methods and protocols. ed. L. M. Vaschetto (New York, NY: Springer US), 105–117.
Fontenelle, A. D. B., Guzzo, S. D., Lucon, C. M. M., and Harakava, R. (2011). Growth promotion and induction of resistance in tomato plant against Xanthomonas euvesicatoria and Alternaria solani by Trichoderma spp. Crop Prot. 30, 1492–1500. doi: 10.1016/j.cropro.2011.07.019
Friberg, H., Lagerlöf, J., and Rämert, B. (2005). Germination of Plasmodiophora brassicae resting spores stimulated by a non-host plant. Eur. J. Plant Pathol. 113, 275–281. doi: 10.1007/s10658-005-2797-0
Garman, H. (1890). A bacterial disease of cabbage. Third annual report for year 1990. Kentucky Agric. Exp. Station 3, 43–46.
Gaumann, E. (1923). Contributions toward a monograph of the genus Peronospora corda. Beitrage zur kryptogamenflora de schweiz 5:360.
Gazdik, F., Magnus, S., Roberts, S. J., Baranski, R., Cechova, J., Pokluda, R., et al. (2021). Persistence of Xanthomonas campestris pv. Campestris in field soil in Central Europe. Microorganisms 9:591. doi: 10.3390/microorganisms9030591
Gibbs, J. G. (1931). Club-root in cruciferous crops. Investigation by plant Research Station. N. Z. J. Agric. 42, 1–17.
Göker, M., Voglmayr, H., Blázquez, G. G., and Oberwinkler, F. (2009). Species delimitation in downy mildews: the case of Hyaloperonospora in the light of nuclear ribosomal ITS and LSU sequences. Mycol. Res. 113, 308–325. doi: 10.1016/j.mycres.2008.11.006
Gossen, B. D., Adhikari, K. K. C., and Mcdonald, M. R. (2012). Effect of seeding date on development of clubroot in short-season Brassica crops. Can. J. Plant Pathol. 34, 516–523. doi: 10.1080/07060661.2012.722129
Graichen, K. (1994). Nachweis von resistenz gegenüber dem turnip yellows luteovirus (TuYV) in winterraps und verwandten arten. Vorträge für Pflanzenzüchtung 30, 132–143.
Graichen, K., and Schliephake, E. (1999). "Infestation of winter oilseed rape by turnip yellows luteovirus and its effect on yield in Germany", In: 10th International Rapeseed Conference: International Consultative Group for Research on Rapeseed, 43–44.
Greer, S. F., Hackenberg, D., Gegas, V., Mitrousia, G., Edwards, D., Batley, J., et al. (2021a). Quantitative trait locus mapping of resistance to turnip yellows virus in Brassica rapa and Brassica oleracea and introgression of these resistances by resynthesis into allotetraploid plants for deployment in Brassica napus. Front. Plant Sci. 12:2717. doi: 10.3389/fpls.2021.781385
Greer, S. F., Newbert, M. J., Rodrigues, L. K., Oliveira, A. M., Chaves, A. L. R., Calegario, R. F., et al. (2021b). First report of turnip yellows virus in Brazil. New Disease Reports 44:e12045. doi: 10.1002/ndr2.12045
Gupta, D. K. (1991). Studies on black rot of cabbage in Manipur. Indian J. Mycol. Plant Pathol. 21, 203–204.
Gupta, S. K. (2016). “Brassicas” in Breeding oilseed crops for sustainable production. ed. S. K. Gupta (San Diego: Academic Press), 33–53.
Hackenberg, D., Asare-Bediako, E., Baker, A., Walley, P., Jenner, C., Greer, S. F., et al. (2020). Identification and QTL mapping of resistance to turnip yellows virus (TuYV) in oilseed rape, Brassica napus. Theor. Appl. Genet. 133, 383–393. doi: 10.1007/s00122-019-03469-z
Harling, R., and Kennedy, S. H. (1991). Biological control of Plasmodiophora brassicae using a bait crop. Mededelingen van de Faculteit Landbouwwetenschappen, Rijksuniversiteit Gent 56, 159–170.
Hauser, S., Stevens, M., Mougel, C., Smith, H. G., Fritsch, C., Herrbach, E., et al. (2000). Biological, serological, and molecular variability suggest three distinct Polerovirus species infecting beet or rape. Phytopathology 90, 460–466. doi: 10.1094/PHYTO.2000.90.5.460
Haynes, E., Ramwell, C., Griffiths, T., Walker, D., and Smith, J. (2020). Review of antibiotic use in crops, associated risk of antimicrobial resistance and research gaps. Report to Department for Environment, Food and Rural Affairs (Defra) & The Food Standards Agency (FSA) FS301082, 1–83.
Hodgetts, J., Karamura, G., Johnson, G., Hall, J., Perkins, K., Beed, F., et al. (2015). Development of a lateral flow device for in-field detection and evaluation of PCR-based diagnostic methods for Xanthomonas campestris pv. Musacearum, the causal agent of banana xanthomonas wilt. Plant Pathol. 64, 559–567. doi: 10.1111/ppa.12289
Holtappels, D., Fortuna, K. J., Moons, L., Broeckaert, N., Bäcker, L. E., Venneman, S., et al. (2022). The potential of bacteriophages to control Xanthomonas campestris pv. Campestris at different stages of disease development. Microb. Biotechnol. 15, 1762–1782. doi: 10.1111/1751-7915.14004
Hoser-Krauze, J., Lakowska-Ryk, E., and Antosik, J. (1984). Resistance of cauliflower and broccoli (B. oleracea L. botrytis L.) seedlings to downy mildew, Peronospora parasitica. Cruciferae Newsletter 9, 92–93.
Howard, R. J., Strelkov, S. E., and Harding, M. W. (2010). Clubroot of cruciferous crops–new perspectives on an old disease. Can. J. Plant Pathol. 32, 43–57. doi: 10.1080/07060661003621761
Hunter, P. J., Jones, J. E., and Walsh, J. A. (2002). Involvement of beet western yellows virus, cauliflower mosaic virus, and turnip mosaic virus in internal disorders of stored white cabbage. Phytopathology 92, 816–826. doi: 10.1094/PHYTO.2002.92.8.816
Hwang, S. F., Ahmed, H. U., Zhou, Q., Strelkov, S. E., Gossen, B. D., Peng, G., et al. (2011). Influence of cultivar resistance and inoculum density on root hair infection of canola (Brassica napus) by Plasmodiophora brassicae. Plant Pathol. 60, 820–829. doi: 10.1111/j.1365-3059.2011.02457.x
Hwang, S. F., Strelkov, S. E., Turnbull, G. D., Manolii, V., Howard, R. J., Hartman, M., et al. (2008). Soil treatments and amendments for management of clubroot on canola in Alberta. Canadian J. Plant Sci. 91: 9991010. doi: 10.4141/cjps2011-028
Ignatov, A., Sechler, A., Schuenzel, E. L., Agarkova, I., Oliver, B., Vidaver, A. K., et al. (2007). Genetic diversity in populations of Xanthomonas campestris pv. Campestris in cruciferous weeds in central coastal California. Phytopathology 97, 803–812. doi: 10.1094/PHYTO-97-7-0803
International Seed Federation (2019). Detection of Xanthomonas pv. Campestris and pv. Raphani on Brassica spp. seed [online]. Available at: https://worldseed.org/wp-content/uploads/2019/10/Brassica_spp_Xcc-Xcr_v6.1_2019.pdf (Accessed March 06, 2023)
Jabeen, N. (2020). “Agricultural, economic and societal importance of Brassicaceae plants” in The plant family Brassicaceae: Biology and physiological responses to environmental stresses. ed. M. Hasanuzzaman (Singapore: Springer Singapore), 45–128.
Jacobsohn, R., Greenberger, A., Katan, J., Levi, M., and Alon, H. (1980). Control of Egyptian broomrape (Orobanche aegyptiaca) and other weeds by means of solar heating of the soil by polyethylene mulching. Weed Sci. 28, 312–316. doi: 10.1017/S0043174500055351
Jensen, B. D., Olumide Latunde-Dada, A., Hudson, D., and Lucas, J. A. (1998). Protection of Brassica seedlings against downy mildew and damping-off by seed treatment with CGA 245704, an activator of systemic acquired resistance. Pestic. Sci. 52, 63–69. doi: 10.1002/(SICI)1096-9063(199801)52:1<63::AID-PS660>3.0.CO;2-2
Jensen, B. D., Værbak, S., Munk, L., and Andersen, S. B. (1999). Characterization and inheritance of partial resistance to downy mildew, Peronospora parasitica, in breeding material of broccoli, Brassica oleracea convar botrytis var. italica. Plant Breeding 118, 549–554. doi: 10.1046/j.1439-0523.1999.00409.x
Jensen, B., Vicente, J. G., Manandhar, H., and Roberts, S. (2010). Occurrence and diversity of Xanthomonas campestris pv. Campestris in vegetable brassica fields in Nepal. Plant Dis. 94, 298–305. doi: 10.1094/PDIS-94-3-0298
Jeromela, A. M., Mikić, A. M., Vujić, S., Ćupina, B., Krstić, Đ., Dimitrijević, A., et al. (2017). Potential of legume–brassica intercrops for forage production and green manure: encouragements from a temperate southeast European environment. Front. Plant Sci. 8, 1–7. doi: 10.3389/fpls.2017.00312
Jones, R., Coutts, B., and Hawkes, J. (2007). Yield-limiting potential of beet western yellows virus in Brassica napus. Aust. J. Agric. Res. 58, 788–801. doi: 10.1071/AR06391
Juergens, M., Paetsch, C., Krämer, I., Zahn, M., Rabenstein, F., Schondelmaier, J., et al. (2010). Genetic analyses of the host-pathogen system turnip yellows virus (TuYV)—rapeseed (Brassica napus L.) and development of molecular markers for TuYV-resistance. Theor. Appl. Genet. 120, 735–744. doi: 10.1007/s00122-009-1194-z
Kageyama, K., and Asano, T. (2009). Life cycle of Plasmodiophora brassicae. J. Plant Growth Regul. 28, 203–211. doi: 10.1007/s00344-009-9101-z
Kammerich, J., Beckmann, S., Scharafat, I., and Ludwig-Müller, J. (2014). Suppression of the clubroot pathogen Plasmodiophora brassicae by plant growth promoting formulations in roots of two Brassica species. Plant Pathol. 63, 846–857. doi: 10.1111/ppa.12148
Koenraadt, H., Bilsen, J. G. P. M., and Roberts, S. (2005). Comparative test of four semi-selective agar media for the detection of Xanthomonas campestris pv. Campestris in brassica seeds. Seed Sci. Technol. 33, 115–125. doi: 10.15258/sst.2005.33.1.12
Korbas, M., Jędryczka, M., Kasprzyk, I., Jajor, E., and Kaczmarek, J. (2014). Infestation of polish agricultural soils by Plasmodiophora brassicae along the polish-Ukrainian border. J. Plant Protect. Res. 54, 238–241. doi: 10.2478/jppr-2014-0036
Koul, T. K., and Singh, S. K. (1999). Cultural management of major foliar diseases in Brassica juncea. J. Mycol. Plant Pathol. 29: 87–90.
Krauthausen, H. J., Hörner, G., Zimmermann, S., Voegele, R. T., and Brändle, F. (2018). Competence of Xanthomonas campestris from cruciferous weeds and wallflower (Erysimum cheiri) to induce black rot in cabbage. Eur. J. Plant Pathol. 151, 275–289. doi: 10.1007/s10658-017-1371-x
Krauthausen, H. J., Laun, N., and Wohanka, W. (2011). Methods to reduce the spread of the black rot pathogen, Xanthomonas campestris pv. Campestris, in brassica transplants. J. Plant Dis. Protect. 118, 7–16. doi: 10.1007/BF03356375
Krober, H. (1970). Longevity of conidia of several P. spp. under different environmental conditions. Phytopathol. Z. 69, 64–70.
Kůdela, V., Pokorný, R., and Lebeda, A. (2018). Potential impact of climate change on geographic distribution of plant pathogenic bacteria in Central Europe. Plant Prot. Sci. 45, S27–S32. doi: 10.17221/2832-PPS
Labana, K. S., and Gupta, M. L. (1993). “Importance and origin” in Breeding oilseed brassicas. eds. K. S. Labana, S. S. Banga, and S. K. Banga (Berlin, Heidelberg: Springer Berlin Heidelberg), 1–7.
Lambert, P., and Pascal, T. (2011). Mapping Rm2 gene conferring resistance to the green peach aphid (Myzus persicae Sulzer) in the peach cultivar “Rubira®”. Tree Genet. Genomes 7, 1057–1068. doi: 10.1007/s11295-011-0394-2
Landa, B. B., Montes-Borrego, M., Muñoz-Ledesma, F. J., and Jiménez-Díaz, R. M. (2007). Phylogenetic analysis of downy mildew pathogens of opium poppy and PCR-based in planta and seed detection of Peronospora arborescens. Phytopathology 97, 1380–1390. doi: 10.1094/PHYTO-97-11-1380
Lange, H. W., Tancos, M. A., Carlson, M. O., and Smart, C. D. (2015). Diversity of Xanthomonas campestris isolates from symptomatic crucifers in New York state. Phytopathology 106, 113–122. doi: 10.1094/PHYTO-06-15-0134-R
Langston, D. B., and Cummings, M. P. (2003). Evaluation of bactericides and plant defense activators for control of black rot of cabbage. Plant Pathol. 1, 2594–2595.
Lenka, S., and Ram, S. (1997). A note on the efficacy in vivo of various antibiotics and fungicide chemicals against Xanthomonas campestris pv. Campestris causing black rot of cauliflower. Orissa J. Hortic. 25, 90–92.
Lewis, K. A., Tzilivakis, J., Warner, D., and Green, A. (2016). An international database for pesticide risk assessments and management. Hum. Ecol. Risk Assess. Int. J. 22, 1050–1064. doi: 10.1080/10807039.2015.1133242
Lin, C. Y., and Liang, T. C. (1974). The discharge of conidia of Peronospora brassicae from kohlrabi and the polyethylene cover as a protection to seedlings against infection of this pathogen. Bullet. Agric. Res. 3, 41–48.
Lindbo, J. A., and Dougherty, W. G. (1992). Pathogen-derived resistance to a potyvirus: immune and resistant phenotypes in transgenic tobacco expressing altered forms of a potyvirus coat protein nucleotide sequence. Mol. Plant Microbe Int. 5, 144–153. doi: 10.1094/MPMI-5-144
López-Soriano, P., Noguera, P., Gorris, M. T., Puchades, R., Maquieira, Á., Marco-Noales, E., et al. (2017). Lateral flow immunoassay for on-site detection of Xanthomonas arboricola pv. Pruni in symptomatic field samples. PLoS One 12:e0176201. doi: 10.1371/journal.pone.0176201
Lucas, J. A., Hayter, J. B. R., and Crute, I. R. (1995). The downy mildews: host specificity and pathogenesis. Pathogenesis Host Specificity Plant Dis. 2, 217–238.
Ludwig-Müller, J., Prinsen, E., Rolfe, S. A., and Scholes, J. D. (2009). Metabolism and plant hormone action during clubroot disease. J. Plant Growth Regul. 28, 229–244. doi: 10.1007/s00344-009-9089-4
Marutani-Hert, M., Hert, A., Tudor-Nelson, S., Preston, J., Minsavage, G., Stall, R., et al. (2020). Characterization of three novel genetic loci encoding bacteriocins associated with Xanthomonas perforans. PLoS One 15:e0233301. doi: 10.1371/journal.pone.0233301
Massomo, S. M. S., Nielsen, H., Mabagala, R. B., Mansfeld-Giese, K., Hockenhull, J., and Mortensen, C. N. (2003). Identification and characterisation of Xanthomonas campestris pv. Campestris strains from Tanzania by pathogenicity tests, biolog, rep-PCR and fatty acid methyl ester analysis. Eur. J. Plant Pathol. 109, 775–789. doi: 10.1023/A:1026194402701
Mayo, M. A. (2002). Virology division news: ICTV at the Paris ICV: results of the plenary session and the binomial ballot. Arch. Virol. 147, 2254–2260. doi: 10.1007/s007050200052
McGrann, G. R. D., Gladders, P., Smith, J. A., and Burnett, F. (2016). Control of clubroot (Plasmodiophora brassicae) in oilseed rape using varietal resistance and soil amendments. Field Crop Res. 186, 146–156. doi: 10.1016/j.fcr.2015.11.013
McKeen, W. E. (2009). Black rot of rutabaga in Ontario and its control. Can. J. Plant Pathol. 3, 244–246. doi: 10.1080/07060668109501358
Meena, P. D., Gour, R. B., Gupta, J. C., Singh, H. K., Awasthi, R. P., Netam, R. S., et al. (2013). Non-chemical agents provide tenable, eco-friendly alternatives for the management of the major diseases devastating Indian mustard (Brassica juncea) in India. Crop Prot. 53, 169–174. doi: 10.1016/j.cropro.2013.07.006
Meena, P. D., Thomas, L., and Singh, D. (2016). Assessment of yield losses in Brassica juncea due to downy mildew (Hyaloperonospora brassicae). J. Oilseed Brassica 1, 73–77.
Mehta, N. (2014). Epidemiology and forecasting for the management of rapeseed-mustard diseases. J. Mycol. Plant Pathol. 44:131.
Mehta, N., and Saharan, G. S. (1999). Effect of planting dates on infection and development of white rust and downy mildew disease complex in mustard. J. Mycol. Plant Pathol. 28, 259–265.
Molina, A., Hunt, M. D., and Ryals, J. A. (1998). Impaired fungicide activity in plants blocked in disease resistance signal transduction. Plant Cell 10, 1903–1914. doi: 10.1105/tpc.10.11.1903
Moss, A. (2002). The effects of long-term feeding of extracted rapeseed meal and whole rapeseed on the physical and financial performance, health and welfare of high yielding cows. Report OS59e, Home Grown Cereals Authority, London, UK 1–42.
Moxham, S. E., and Buczacki, S. T. (1983). Chemical composition of the resting spore wall of Plasmodiophora brassicae. Trans. Br. Mycol. Soc. 80, 297–304. doi: 10.1016/S0007-1536(83)80013-8
Mulema, J., Vicente, J. G., Pink, D., Jackson, A., Chacha, D., Wasilwa, L., et al. (2012). Characterisation of isolates that cause black rot of crucifers in East Africa. Eur. J. Plant Pathol. 133, 427–438. doi: 10.1007/s10658-011-9916-x
Myers, D. F., and Campbell, R. N. (1985). Lime and the control of clubroot of crucifers: effects of pH, calcium, magnesium, and their interactions. Phytopathology 75, 670–673. doi: 10.1094/Phyto-75-670
Myers, D. F., Campbell, R. N., and Greathead, A. S. (1981). “Clubroot of crucifers in California-soils respond differentially to lime for clubroot control” in Phytopathology (St Paul, MN: American Phytopathological Society), 1005–1006.
Nagaharu, U. (1935). Genome analysis in Brassica with special reference to the experimental formation of B. napus and peculiar mode of fertilzation. Japan. J. Botany 7, 389–452.
Newbert, M. (2016). The genetic diversity of turnip yellows virus in oilseed rape (Brassica napus) in Europe, pathogenic determinants, new sources of resistance and host range. PhD Thesis for Doctor of Philosophy. Coventry: The University of Warwick.
Nicholls, C., and Stoddart, H. (2014). Restriction of neonics; restriction on crop protection? [online]. Available at: http://cereals.ahdb.org.uk/media/677181/AAB-Pollinators-in-agriculture-conference-neonics-abstract-April-2014.pdf (Accessed May 10, 2016)
Niu, L., Pan, L., Zeng, W., Lu, Z., Cui, G., Fan, M., et al. (2018). Dynamic transcriptomes of resistant and susceptible peach lines after infestation by green peach aphids (Myzus persicae Sülzer) reveal defence responses controlled by the Rm3 locus. BMC Genomics 19:846. doi: 10.1186/s12864-018-5215-7
Nuñez, A. M. P., Rodríguez, G. A. A., Monteiro, F. P., Faria, A. F., Silva, J. C. P., Monteiro, A. C. A., et al. (2018). Bio-based products control black rot (Xanthomonas campestris pv. Campestris) and increase the nutraceutical and antioxidant components in kale. Sci. Rep. 8:10199. doi: 10.1038/s41598-018-28086-6
Orynbayev, A., Miroshnikov, K., Ignatov, A., and Dzhalilov, F. (2020). “Evaluation of effectiveness of bacteriophage agent for cabbage black rot control” in Растениеводство и луговодство. doi: 10.26897/978-5-9675-1762-4-2020-113
Orynbayev, A., Кабанова, А., Образцова, Е., Ignatov, A., Miroshnikov, K., and Dzhalilov, F. (2019). “Isolation of bacteriophages specific for Xanthomonas campestris pv. Campestris and biocontrol of black rot disease of cabbage” in Bacterial diseases of potato and vegetables: Diagnostics and control. doi: 10.34677/0021-342X-2019-2-35-48
Paliwal, D., Hamilton, A. J., Barrett, G. A., Alberti, F., Van Emden, H., Monteil, C. L., et al. (2022). Identification of novel aphid-killing bacteria to protect plants. Microb. Biotechnol. 15, 1203–1220. doi: 10.1111/1751-7915.13902
Pammel, L. H. (1895). Bacteriosis of rutabaga (Bacillus campestris n. sp). Iowa Agric. Exp. Station Bullet. 27, 130–134.
Papaianni, M., Ricciardelli, A., Fulgione, A., D’errico, G., Zoina, A., Lorito, M., et al. (2020). Antibiofilm activity of a Trichoderma metabolite against Xanthomonas campestris pv. Campestris, alone and in association with a phage. Microorganisms 8:620. doi: 10.3390/microorganisms8050620
Pascal, T., Aberlenc, R., Confolent, C., Hoerter, M., Lecerf, E., Tuéro, C., et al. (2017). Mapping of new resistance (Vr2, Rm1) and ornamental (Di2, pl) Mendelian trait loci in peach. Euphytica 213:132. doi: 10.1007/s10681-017-1921-5
Patikarnmonthon, N., Nawapan, S., Buranajitpakorn, S., Charoenlap, N., Mongkolsuk, S., and Vattanaviboon, P. (2010). Copper ions potentiate organic hydroperoxide and hydrogen peroxide toxicity through different mechanisms in Xanthomonas campestris pv. Campestris. FEMS Microbiol. Lett. 313, 75–80. doi: 10.1111/j.1574-6968.2010.02124.x
Peng, G., Lahlali, R., Hwang, S.-F., Pageau, D., Hynes, R. K., Mcdonald, M. R., et al. (2014). Crop rotation, cultivar resistance, and fungicides/biofungicides for managing clubroot (Plasmodiophora brassicae) on canola. Can. J. Plant Pathol. 36, 99–112. doi: 10.1080/07060661.2013.860398
Peng, G., Mcgregor, L., Lahlali, R., Gossen, B. D., Hwang, S. F., Adhikari, K. K., et al. (2011). Potential biological control of clubroot on canola and crucifer vegetable crops. Plant Pathol. 60, 566–574. doi: 10.1111/j.1365-3059.2010.02400.x
Peng, S. S., Ren, Z. H., Huang, X. L., Liu, M. J., Sun, L. F., and Liu, E. M. (2013). Physiological race identification on clubroot of cruciferous crops caused by Plasmodiophora brassicae in Hunan. J. Changjiang Veg. 6, 46–49.
Pham, H. T., Riu, K. Z., Jang, K. M., Cho, S. K., and Cho, M. (2004). Bactericidal activity of glycinecin a, a bacteriocin derived from Xanthomonas campestris pv. Glycines, on phytopathogenic Xanthomonas campestris pv. Vesicatoria cells. Appl. Environ. Microbiol. 70, 4486–4490. doi: 10.1128/AEM.70.8.4486-4490.2004
Popović, T., Balaž, J., Starović, M., Trkulja, N., Ivanović, Ž., Ignjatov, M., et al. (2013). First report of Xanthomonas campestris pv. Campestris as the causal agent of black rot on oilseed rape (Brassica napus) in Serbia. Plant Dis. 97:418. doi: 10.1094/PDIS-05-12-0506-PDN
Popović, T., Mitrović, P., Jelušić, A., Dimkić, I., Marjanović-Jeromela, A., Nikolić, I., et al. (2019). Genetic diversity and virulence of Xanthomonas campestris pv. Campestris isolates from Brassica napus and six Brassica oleracea crops in Serbia. Plant Pathol. 68, 1448–1457. doi: 10.1111/ppa.13064
Rastas, M., Latvala, S., and Hannukkala, A. (2012). Occurrence of Plasmodiophora brassicae in Finnish turnip rape and oilseed rape fields. Agric. Food Sci. 21, 141–158. doi: 10.23986/afsci.6249
Ren, L., Xu, L., Liu, F., Chen, K., Sun, C., Li, J., et al. (2016). Host range of Plasmodiophora brassicae on cruciferous crops and weeds in China. Plant Dis. 100, 933–939. doi: 10.1094/PDIS-09-15-1082-RE
Rennie, D. C., Manolii, V. P., Cao, T., Hwang, S. F., Howard, R. J., and Strelkov, S. E. (2011). Direct evidence of surface infestation of seeds and tubers by Plasmodiophora brassicae and quantification of spore loads. Plant Pathol. 60, 811–819. doi: 10.1111/j.1365-3059.2011.02449.x
RHS (2023). Brassica downy mildew [online]. Available at: https://www.rhs.org.uk/disease/brassica-downy-mildew (Accessed April 05, 2023)
Řičařová, V., Kazda, J., Singh, K., and Ryšánek, P. (2016). Clubroot caused by Plasmodiophora brassicae Wor.: a review of emerging serious disease of oilseed rape in the Czech Republic. Plant Prot. Sci. 52, 71–86. doi: 10.17221/87/2015-PPS
Ridout, C. (2018). Creating a baby-leaf kale resistant to downy mildew [online]. Available at: https://www.jic.ac.uk/blog/baby-leaf-kale-resistant-to-downy-mildew-disease-created-in-collaboration-between-industry-and-science/ (Accessed April 18, 2023)
Roberts, S. J., Branfield, L., and Payne, S. R. (2022). Improved management of bacterial diseases of horticultural crops. Agriculture and Horticulture Development Board CP191 Final Report, 1–64.
Rod, J. (1992). The effect of seed treatment on club-root (Plasmodiophora brassicae) incidence. Ochrana Rostlin-UVTIZ (CSFR), 28, 1–7.
Russell, G. E., and Duffus, J. E. (1970). An aphid-transmitted yellowing virus disease of lettuce in England. Plant Pathol. 19, 148–149.
Saharan, G. S., Mehta, N., and Meena, P. D. (2017). Downy mildew disease of crucifers: Biology, ecology and disease management. Singapore: Springer Singapore.
Saharan, G. S., Mehta, N. K., and Meena, P. D. (2021). “Clubroot perspective” in Clubroot disease of crucifers: Biology, ecology and disease management (Singapore: Springer Singapore), 1–45.
Sain, S. K., Gour, H. N., and Sharma, P. (2007). Evaluation of botanicals and PGPRs against Xanthomonas campestris pv. Campestris, an incitant of black rot of cauliflower. J. Eco Friend. Agric. 2, 178–182.
Salcedo, A. F., Purayannur, S., Standish, J. R., Miles, T., Thiessen, L., and Quesada-Ocampo, L. M. (2021). Fantastic downy mildew pathogens and how to find them: advances in detection and diagnostics. Plan. Theory 10:435. doi: 10.3390/plants10030435
Sanna, M., Gilardi, G., Gullino, M. L., and Mezzalama, M. (2022). Evaluation of physical and chemical disinfection methods of Brassica oleracea seeds naturally contaminated with Xanthomonas campestris pv. Campestris. J. Plant Dis. Protect. 129, 1145–1152. doi: 10.1007/s41348-022-00635-2
Savary, S., Ficke, A., Aubertot, J.-N., and Hollier, C. (2012). Crop losses due to diseases and their implications for global food production losses and food security. Food Security 4, 519–537. doi: 10.1007/s12571-012-0200-5
Scheets, K., Miller, W. A., and Somera, M. (2020). Abolish the family Luteoviridae (Tolivirales) and move its genera to the familes Tombusviridae (Tolivirales) and Solemoviridae (Sobelivirales). Int. Committee Tax. Viruses 2020, 1–10.
Schisler, D. A., Slininger, P. J., Behle, R. W., and Jackson, M. A. (2004). Formulation of Bacillus spp. for biological control of plant diseases. Phytopathology 94, 1267–1271. doi: 10.1094/PHYTO.2004.94.11.1267
Schliephake, E., Graichen, K., and Rabenstein, F. (2000). Investigations on the vector transmission of the beet mild yellowing virus (BMYV) and the turnip yellows virus (TuYV). J. Plant Dis. Protect. 107, 81–87.
Schroeder, K. L., Martin, F. N., De Cock, A. W. A. M., Lévesque, C. A., Spies, C. F. J., Okubara, P. A., et al. (2013). Molecular detection and quantification of Pythium species: evolving taxonomy, new tools, and challenges. Plant Dis. 97, 4–20. doi: 10.1094/PDIS-03-12-0243-FE
Sharma, K., Gossen, B. D., and Mcdonald, M. R. (2011). Effect of temperature on primary infection by Plasmodiophora brassicae and initiation of clubroot symptoms. Plant Pathol. 60, 830–838. doi: 10.1111/j.1365-3059.2011.02458.x
Shaw, R. K., Shen, Y., Wang, J., Sheng, X., Zhao, Z., Yu, H., et al. (2021). Advances in multi-omics approaches for molecular breeding of black rot resistance in Brassica oleracea L. Front. Plant Sci. 12:742553. doi: 10.3389/fpls.2021.742553
Sherf, A. F., and Macnab, A. A. (1986). Vegetable diseases and their control. Hoboken, NJ: John Wiley & Sons.
Shiraushi, M., Sakamoto, K., Asada, Y., Nagatani, T., and Hidaka, H. (1975). A scanning electron microscopic observation on the surface of Japanese radish leaves infected by Peronospora parasitica (Fr.) Fr. Japan. J. Phytopathol. 41, 24–32. doi: 10.3186/jjphytopath.41.24
Silva Júnior, T. A. F., Silva, J. C., Gonçalves, R. M., Soman, J. M., Passos, J. R. S., and Maringoni, A. C. (2020). Survival of Xanthomonas campestris pv. Campestris associated with soil and cauliflower crop debris under Brazilian conditions. Eur. J. Plant Pathol. 156, 399–411. doi: 10.1007/s10658-019-01888-8
Singh, S., Dey, S. S., Bhatia, R., Batley, J., and Kumar, R. (2018). Molecular breeding for resistance to black rot [Xanthomonas campestris pv. Campestris (Pammel) Dowson] in brassicas: recent advances. Euphytica 214:196. doi: 10.1007/s10681-018-2275-3
Singh, U. S., Nashaat, N. I., Doughty, K. J., and Awasthi, R. P. (2002). Altered phenotypic response to Peronospora parasitica in Brassica juncea seedlings following prior inoculation with an avirulent or virulent isolate of Albugo candida. Eur. J. Plant Pathol. 108, 555–564. doi: 10.1023/A:1019937115378
Singh, D., Rathaur, P. S., and Vicente, J. G. (2016). Characterization, genetic diversity and distribution of Xanthomonas campestris pv. Campestris races causing black rot disease in cruciferous crops of India. Plant Pathol. 65, 1411–1418. doi: 10.1111/ppa.12508
Slater, R., Paul, V., Andrews, M., Garbay, M., and Camblin, P. (2012). Identifying the presence of neonicotinoid resistant peach-potato aphid (Myzus persicae) in the peach-growing regions of southern France and northern Spain. Pest Manag. Sci. 68, 634–638. doi: 10.1002/ps.2307
Slusarenko, A. J., and Schlaich, N. L. (2003). Downy mildew of Arabidopsis thaliana caused by Hyaloperonospora parasitica (formerly Peronospora parasitica). Mol. Plant Pathol. 4, 159–170. doi: 10.1046/j.1364-3703.2003.00166.x
SmartProtect (2023). SmartProtect platform – monitoring techniques for bacteria [online]. Available at: https://platform.smartprotect-h2020.eu/en (Accessed May 12, 2023)
Smith, E. F. (1898). “The black rot of the cabbage” in Farmers’ bulletin (United States Department of Agriculture) no. 68 (Washington: US Dept. of Agriculture, Government Printing Office) 1–20.
Stavridou, E., Kristensen, H. L., Krumbein, A., Schreiner, M., and Thorup-Kristensen, K. (2012). Effect of differential N and S competition in inter- and sole cropping of Brassica species and lettuce on glucosinolate concentration. J. Agric. Food Chem. 60, 6268–6278. doi: 10.1021/jf300091e
Stevens, M., Freeman, B., Liu, H.-Y., Herrbach, E., and Lemaire, O. (2005). Beet poleroviruses: close friends or distant relatives? Mol. Plant Pathol. 6, 1–9. doi: 10.1111/j.1364-3703.2004.00258.x
Stevens, M., Mcgrann, G., and Clark, B. (2008). Turnip yellows virus (syn. Beet western yellows virus): an emerging threat to European oilseed rape production? Agric. Hortic. Dev. Res. Rev. 69, 1–32.
Takahashi, K., and Yamaguchi, T. (1988). A method for assessing the pathogenic activity of resting spores of Plasmodiophora brassicae by fluorescence microscopy. Japan. J. Phytopathol. 54, 466–475. doi: 10.3186/jjphytopath.54.466
Takahashi, K., and Yamaguchi, T. (1989). Assessment of pathogenicity of resting spores of Plasmodiophora brassicae in soil by fluorescence microscopy. Japan. J. Phytopathol. 55, 621–628. doi: 10.3186/jjphytopath.55.621
Taylor, J. D., Conway, J., Roberts, S. J., Astley, D., and Vicente, J. G. (2002). Sources and origin of resistance to Xanthomonas campestris pv. Campestris in Brassica genomes. Phytopathology 92, 105–111. doi: 10.1094/PHYTO.2002.92.1.105
The European Commission (2013). Commission implementing regulation (EU) no 485/2013 of 24 May 2013. Amending implementing regulation (EU) no 540/2011, as regards the conditions of approval of the active substances clothianidin, thiamethoxam and imidacloprid, and prohibiting the use and sale of seeds treated with plant protection products containing those active substances. OJEU 139, 12–26.
Thomas, P. E., Evans, D. W., Fox, L., and Biever, K. D. (1990). Resistance to beet western yellows virus among forage brasssicas. Plant Dis. 74, 327–330. doi: 10.1094/PD-74-0327
Tilston, E. L., Pitt, D., and Groenhof, A. C. (2002). Composted recycled organic matter suppresses soil-borne diseases of field crops. New Phytol. 154, 731–740. doi: 10.1046/j.1469-8137.2002.00411.x
Timila, R. D., Correll, J. C., and Duwadi, V. R. (2008). Severe and widespread clubroot epidemics in Nepal. Plant Dis. 92:317. doi: 10.1094/PDIS-92-2-0317B
Tremblay, N., Belec, C., Coulombe, J., and Godin, C. (2005). Evaluation of calcium cyanamide and liming for control of clubroot disease in cauliflower. Crop Prot. 24, 798–803. doi: 10.1016/j.cropro.2004.12.013
UKRI (2022). Strategic guidance for applicants to call for crop pests and diseases business collaboration awards [online]. Available at: https://www.ukri.org/wp-content/uploads/2022/06/BBSRC-010622-StrategicGuidanceforApplicants.pdf#:~:text=Strategic%20Guidance%20for%20Applicants%20to%20call%20for%20crop,and%20control%20of%20priority%20crop%20pests%20and%20diseases (Accessed April 18, 2023)
USDA (2020). Oilseeds: world markets and trade. Foreign Agricultural Service. United States Department of Agriculture October 2020 report, 1–41.
Usuki, F., and Narisawa, K. (2007). A mutualistic symbiosis between a dark septate endophytic fungus, Heteroconium chaetospira, and a nonmycorrhizal plant, Chinese cabbage. Mycologia 99, 175–184. doi: 10.1080/15572536.2007.11832577
Verma, T. S., and Thakur, P. C. (1989). Comparative field resistance of cabbage collection to downy mildew at seedling stage. Short communication. Indian J. Plant Protect. 17:79–80.
Vicente, J. G., Conway, J., Roberts, S. J., and Taylor, J. D. (2001). Identification and origin of Xanthomonas campestris pv. Campestris races and related pathovars. Phytopathology 91, 492–499. doi: 10.1094/PHYTO.2001.91.5.492
Vicente, J. G., Gunn, N. D., Bailey, L., Pink, D. A. C., and Holub, E. B. (2012). Genetics of resistance to downy mildew in Brassica oleracea and breeding towards durable disease control for UK vegetable production. Plant Pathol. 61, 600–609. doi: 10.1111/j.1365-3059.2011.02539.x
Vicente, J. G., and Holub, E. B. (2013). Xanthomonas campestris pv. Campestris (cause of black rot of crucifers) in the genomic era is still a worldwide threat to brassica crops. Mol. Plant Pathol. 14, 2–18. doi: 10.1111/j.1364-3703.2012.00833.x
Voglmayr, H. (2003). Phylogenetic relationships of Peronospora and related genera based on nuclear ribosomal ITS sequences. Mycol. Res. 107, 1132–1142. doi: 10.1017/S0953756203008438
Wallace, E. C., and Quesada-Ocampo, L. M. (2017). Analysis of microsatellites from the transcriptome of downy mildew pathogens and their application for characterization of Pseudoperonospora populations. PeerJ 5:e3266. doi: 10.7717/peerj.3266
Wallenhammar, A.-C., Almquist, C., Schwelm, A., Roos, J., Marzec-Schmidt, K., Jonsson, A., et al. (2014). Clubroot, a persistent threat to Swedish oilseed rape production. Can. J. Plant Pathol. 36, 135–141. doi: 10.1080/07060661.2013.870606
Walsh, J. A., Richards, A., Sharpe, C., Kevitt-Jack, J., Jones, J. D. G., and Mead, A. (2011). The incidence of turnip yellows virus (TuYV) in overwintered cauliflower and Brussels sprout and the effect of the virus on yield quality and storage. Agriculture and Horticulture Development Board Final report – FV 365.
Wang, W., Devoto, A., Turner, J. G., and Xiao, S. (2007). Expression of the membrane-associated resistance protein RPW8 enhances basal defense against biotrophic pathogens. Mol. Plant Microbe Interact. 20, 966–976. doi: 10.1094/MPMI-20-8-0966
Keywords: Brassica oleracea, integrated pest management, black rot, clubroot, downy mildew, turnip yellows virus, aphids, plant disease
Citation: Greer SF, Surendran A, Grant M and Lillywhite R (2023) The current status, challenges, and future perspectives for managing diseases of brassicas. Front. Microbiol. 14:1209258. doi: 10.3389/fmicb.2023.1209258
Edited by:
Yasmeen Siddiqui, Universiti Putra Malaysia, MalaysiaReviewed by:
Khamis Youssef, Agricultural Research Center, EgyptAjit Singh, University of Nottingham Malaysia Campus, Malaysia
Copyright © 2023 Greer, Surendran, Grant and Lillywhite. This is an open-access article distributed under the terms of the Creative Commons Attribution License (CC BY). The use, distribution or reproduction in other forums is permitted, provided the original author(s) and the copyright owner(s) are credited and that the original publication in this journal is cited, in accordance with accepted academic practice. No use, distribution or reproduction is permitted which does not comply with these terms.
*Correspondence: Shannon F. Greer, S.F.Easterlow@warwick.ac.uk; Arthy Surendran, Arthy.Surendran@sruc.ac.uk
†These authors have contributed equally to this work and share first authorship