- 1Department of Plant Protection, Rural Engineering and Soils, School of Engineering, São Paulo State University - UNESP-FEIS, Ilha Solteira, São Paulo, Brazil
- 2Department of Biology and Zootechnics, Lab of Plant Morphology and Anatomy/Lab Plant Metabolism and Physiology, School of Engineering, São Paulo State University - UNESP-FEIS, Ilha Solteira, São Paulo, Brazil
- 3Department of Crop Science, State University of Mato Grosso do Sul – UEMS, Mundo Novo, Mato Grosso do Sul, Brazil
- 4Department of Plant and Environmental Sciences, Faculty of Science, University of Copenhagen, Copenhagen, Denmark
- 5Botany and Microbiology Department, College of Science, King Saud University, Riyadh, Saudi Arabia
- 6Laboratory for Molecular Plant Physiology and Biotechnology, Department of Biology, University of Antwerp, Antwerp, Belgium
Inoculation with Bacillus subtilis is a promising approach to increase plant yield and nutrient acquisition. In this context, this study aimed to estimate the B. subtilis concentration that increases yield, gas exchange, and nutrition of lettuce plants in a hydroponic system. The research was carried out in a greenhouse in Ilha Solteira, Brazil. A randomized block design with five replications was adopted. The treatments consisted of B. subtilis concentrations in nutrient solution [0 mL “non-inoculated”, 7.8 × 103, 15.6 × 103, 31.2 × 103, and 62.4 × 103 colony forming units (CFU) mL−1 of nutrient solution]. There was an increase of 20% and 19% in number of leaves and 22% and 25% in shoot fresh mass with B. subtilis concentrations of 15.6 × 103 and 31.2 × 103 CFU mL−1 as compared to the non-inoculated plants, respectively. Also, B. subtilis concentration at 31.2 × 103 CFU mL−1 increased net photosynthesis rate by 95%, intercellular CO2 concentration by 30%, and water use efficiency by 67% as compared to the non-inoculated treatments. The concentration of 7.8 × 103 CFU mL−1 improved shoot accumulation of Ca, Mg, and S by 109%, 74%, and 69%, when compared with non-inoculated plants, respectively. Inoculation with B. subtilis at 15.6 × 103 CFU mL−1 provided the highest fresh leaves yield while inoculation at 15.6 × 103 and 31.2 × 103 CFU mL−1 increased shoot fresh mass and number of leaves. Concentrations of 7.8 × 103 and 15.6 × 103 increased shoot K accumulation. The concentrations of 7.8 × 103, 15.6 × 103, and 31.2 × 103 CFU mL−1 increased shoot N accumulation in hydroponic lettuce plants.
1 Introduction
Lettuce, a low-calorie, low-fat, and low-sodium salad vegetable, is an abundant source of natural health-promoting phytochemicals. It is a substance-rich source of vitamins, including glycosylated flavonoids, hydroxycinnamic acids, sesquiterpene lactones (e.g., lactucin), carotenoids, B-group vitamins, vitamin C, tocopherol, and ascorbic acid. It is also rich in iron (Fe). Secondary metabolites of lettuce are potentially associated with many beneficial health properties, including anti-free radicals, anti-cardiovascular, anti-inflammatory, anti-cystic, and anti-diabetic effects (Kim et al., 2016; Yang et al., 2022).
Lettuce production is increasing worldwide, and the possibility of its production throughout the year has been a problem, especially when cultivated in the soil. In addition, root–leaf diseases during prolonged rain conditions are another factor affecting lettuce production (Opatovsky et al., 2019). The nutrient solution recirculation system in the Nutrient Film Technique (NFT) in hydroponics provides more efficient disease management in lettuce cultivation, increases plant precocity and yield, and reduces water consumption compared to the production of leafy vegetables in soil (Dalastra et al., 2020). Greater efficiency in water use is essential for the global water crisis (Kappel et al., 2021), in addition to increasing the absorption and concentration of nutrients in lettuce plants (Aini et al., 2019).
Plant growth-promoting bacteria that improve growth, nutrient acquisition, water use efficiency, and yield gains have been extensively studied in production systems under field conditions (Fukami et al., 2018). Inoculation with Bacillus subtilis has previously been associated with several mechanisms that promote plant growth, such as producing phytohormones and siderophores and increasing nutrient acquisition (Kang et al., 2019). In a study with the inoculation of Bacillus spp. in an aquaponic system with lettuce, it was possible to verify an increase in shoot and root growth, acquisition of N and P, and even improved plant quality for human consumption (Kasozi et al., 2021).
The use of B. subtilis in the nutrient solution (inoculation) to improve the cultivation of lettuce in hydroponics with the intent to produce healthy and quality foods, mitigate the consumption of fertilizers, and increase the yield of lettuce in hydroponic cultivation can be a valuable alternative. Owing to the scarcity of studies related to inoculation in hydroponic systems, a study to find the ideal concentration of inoculant in the nutrient solution is indispensable. In this context, this study aimed to estimate the B. subtilis concentration that promotes increases in yield, gas exchange, and nutrition of lettuce plants in a hydroponic system.
2 Materials and methods
2.1 Environmental characterization
The experiment was conducted in an NFT hydroponic lettuce cultivation system in a greenhouse with 30% shading at São Paulo State University - (UNESP), Ilha Solteira - SP, Brazil (20°25′07″ S, 51°20′31″ W, and an altitude of 376 m). Weather data were collected outside the greenhouse, in the UNESP weather station, between 2 September and 4 October 2021 (Figure 1).
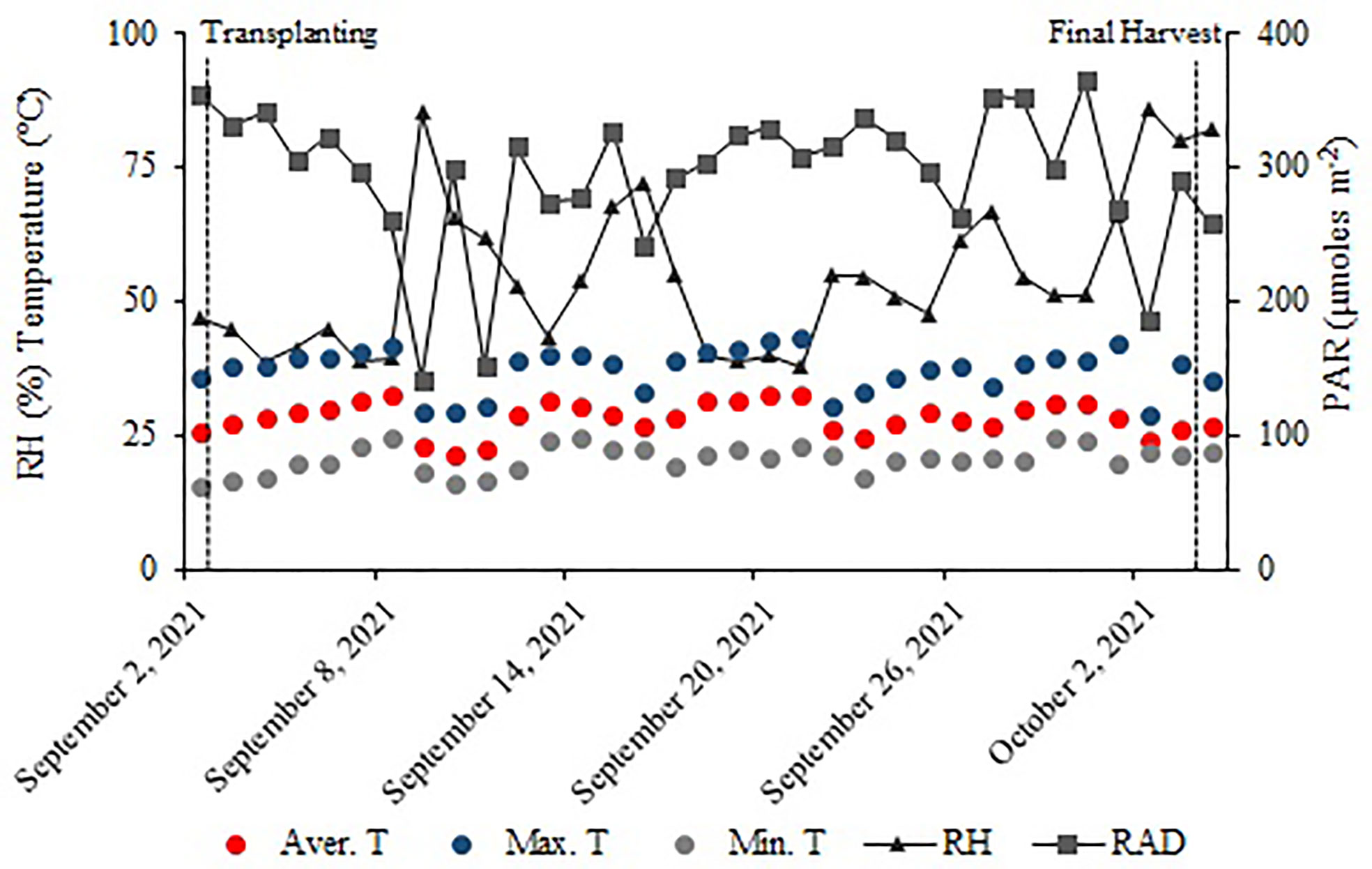
Figure 1 Relative air humidity (RH); maximum (Max. T), average (Avg. T), and minimum (Min. T) temperatures; and PAR radiation (RAD) during the experiment conduction.
2.2 Experimental design
The randomized block design with five replications was adopted. Each experimental unit consisted of four lettuce plants randomly collected on the bench in the four central channels in the hydroponic. The treatments comprised five concentrations of B. subtilis, strain CCTB04, in the nutrient solution, which were obtained by diluting a liquid inoculant containing 1 × 108 colony forming units (CFU) mL−1 in the nutrient solution of the reservoirs of the hydroponic system. The following doses of inoculant were diluted in 100 L of nutrient solution: 0.0 (non-inoculated), 7.8, 15.6, 31.2, and 62.4 mL, obtaining the following concentrations of B. subtilis: 0.0, 7.8 × 103, 15.6 × 103, 31.2 × 103, and 62.4 × 103 CFU mL−1 of nutrient solution. The inoculant doses were applied in the nutrient solution only on the day of transplanting the lettuce seedlings. The isolates of Bacillus subtilis, strain CCTB04 were obtained from the collection of the Brazilian Agricultural Research Corporation (EMBRAPA). This isolate was sent to the UNESP microbiology laboratory for multiplication. The multiplication of bacteria was carried out in Starch Casein Agar medium (10 g of starch, 0.3 g of casein, 2 g of KNO3, 2 g of NaCl, 2 g of K2HPO, 0.05 g of MgSO4.7H2O, 0.01 g of FeSO4.7H2O, 16 g of Agar, and 1,000 mL of distilled water) and incubated at 25°C for 7 days under constant light (Bettiol et al., 2005). The colony count of the liquid inoculant was carried out using a digital colony counter (CP 600 Plus, Phoenix Ind. Com. Equipamentos Científicos Ltda, Araraquara, SP, Brazil). The colony-forming units per milliliter of the B. subtilis, liquid inoculant, was calculated at 1 × 108 CFU mL−1.
2.3 Experimental characterization
The experiment was set up in a Nutrient Film Technique (NFT) system in individual benches of 6 m in length and 10% slope. The cultivation channels were made of PVC with a rectangular section 8 cm wide and 4 cm high and upper perforations to accommodate plants every 25 cm (Supplementary Figure 2). Each bench consisted of six cultivation channels 20 cm apart with an individual pumping system and a 300-L reservoir with a flow rate of 1 L per minute and continuous flow of the nutrient solution.
Iceberg lettuce, Angelina cultivar, was used. This cultivar shows vigorous growth with closed, compact, and uniform heads under cultivation in soil and hydroponic systems, moderate levels of resistance to bacteriosis, intense and bright green leaves, and safety of planting in periods of climatic oscillations (high adaptation to tropical growing conditions).
The seedlings were developed in phenolic foam for 15 days and later transplanted to the benches of the NTF system, where they remained for 31 days until harvest. The nutrient solution composed of concentrated fertilizers from Hidrogood Fert was used at a concentration of (0.666 g L−1) indicated for all stages of crop development, with the following nutrient concentrations: 10% N, 9% phosphorus (P), 28% potassium (K), 4.3% sulfur (S), 3.3% magnesium (Mg), 0.06% boron (B), 0.01% copper (Cu), 0.05% manganese (Mn), 0.07% molybdenum (Mo), and 0.02% zinc (Zn). Also, calcium nitrate (15.5% N and 26.5% calcium) at 0.495 g L−1 and Hidrogood Fert Iron EDDHA (6% iron) at 0.020 g L−1 were used.
Electrical conductivity (EC) and pH were measured and adjusted daily in the morning. On this occasion, the EC was readjusted to the EC determined for each cultivation bench with the replacement of fertilizers if necessary. This EC readjustment was conducted on the 1st, 11th, and 21st day after transplantation (DAT). The initial EC was 1.3 dS m−1; these values were increased according to the stage of cultivation and its response to fertilization. At the 11th DAT, the EC was increased to 1.5 dS m−1, and at the 21st DAT, it was raised to 1.7 dS m−1 and remained until harvest. Weekly supplementation with 20 mg L−1 of K2O (KCl) was carried out in all cultivation benches to improve the water status of the plants due to the effect of the high temperatures in the region (a fact observed in previous studies since non-supplementation caused wilting in the plants in the period of higher daily temperature). To maintain the pH between 6.0 and 6.5, sulfuric acid (25%) was used when pH was above 6.5, and sodium hydroxide (25%) when pH was below 6.0.
The EC and pH adjustment were described according to fertilizer uses (Supplementary Figures 3A–E). The effect of inoculation in the nutrient solution caused an increase in EC without adding nutrients and thus avoided the replacement of nutrients for a longer period. A hypothesis for the EC increase without adding nutrients is the occurrence of biological nitrogen fixation (BNF) due to the presence of Bacillus subtilis in the nutrient solution. The daily elevations of EC by inoculation were described (Supplementary Figure 3F).
2.4 Biometric and yield assessments
The evaluations were carried out 31 DAT the lettuce seedlings (harvest). Eight plants were collected and separated to quantify the number of leaves of each plant and the fresh matter of the root system and the shoot (in grams) using an analytical scale (0.001 kg). Then, the plants were dried in an air-forced circulation oven at 60°C for 72 h to obtain the dry matter of the root system and shoot (in grams) using a precision scale (0.001 g). Lettuce yield was estimated according to Equation 1.
LY—Lettuce yield (kg m−2);
SFM—Shoot fresh matter (kg plant−1);
PP—Plant population equal to 19.5 plants m−2.
2.5 Nutritional assessments
After drying, weighing, and grinding the plants in a Wiley-type mill, the concentrations of N, P, K, S, Ca, and Mg in the shoots and roots of lettuce at harvest (31 DAT) were determined according to the methodology of Malavolta et al. (1997). The accumulation of nutrients in the shoots and roots of the plants was calculated based on the respective dry matter and nutrient concentrations obtained. The accumulation of nutrients was estimated according to Equation 2.
NA—Nutrient accumulation (g m−2);
DM—Dry matter (shoot or root) (kg m−2);
NC—Nutrient concentration of N, P, K, Ca, Mg, and S (g kg−1).
2.6 Physiological assessments
The evaluation of gas exchange was carried out in eight plants per plot at the time of harvest, between 9 a.m. and 11 a.m., in fully expanded leaves located in the middle part of the plant, using an infrared gas analyzer (Infra-Red Gas Analyzer—IRGA, model CRS300). The intercellular CO2 concentration (Ci—μmol CO2 mol−1 air−1), stomatal conductance (gs—mmol of H2O m−2 s−1), transpiration (E—mmol of H2O m−2 s−1), net photosynthesis rate (A—μmol CO2 m−2 s−1), and water use efficiency (WUE—mmol CO2 mol−1 H2O−1) were evaluated.
The third fully expanded leaf from the apex was collected between 9 a.m. and 10 a.m. to avoid large interferences of light intensity on the activity of the enzyme and leaf chlorophyll. Fresh leaf tissue samples were collected, stored in plastic bags, transported to the laboratory with ice, and washed with deionized water.
The total chlorophyll content (ChlT) was determined using the DMSO extracting agent. Leaf tissue (50 mg) was cut into 1-mm fragments and incubated in 7 mL of DMSO in the dark in a water bath at 65°C for 30 min, according to Hiscox and Israelstam (1979). After readings in the spectrophotometer, the contents of the photosynthetic pigments were calculated and expressed in mg g−1 fresh weight (FW). ChlT = (20.20 × ABS663) + (8.02 × ABS663).
2.7 Data analysis
The data of all variables presented normal distribution and homogeneous variances (Shapiro–Wilk test). The data were submitted for analysis of variance, and the significance of the mean squares obtained was tested by the F-test at a 5% probability level. The means relative to concentrations of B. subtilis were compared by the Tukey test at 5% probability.
3 Results
3.1 Yield components
There was a significant effect of B. subtilis on the fresh and dry matter of shoot and roots, the number of leaves, and the leaf yield of lettuce in the hydroponic system (Supplementary Table 1). The highest number of leaves were 18.8 and 18.6 leaves plant−1 at B. subtilis concentrations of 15.6 × 103 and 31.2 × 103 CFU mL−1 and the highest shoot fresh matter were 358, 382, and 394 g plant−1 at B. subtilis concentrations of 7.8 × 103, 15.6 × 103, and 31.2 × 103 CFU mL−1, respectively. The higher number of leaves results in greater use of the plant to prepare salads (Figures 2A, C). The highest leaf yield (7.56 and 7.14 kg m−2 of fresh leaves of lettuce) occurred with the inoculation with B. subtilis at 15.6 × 103 and 31.2 × 103 CFU mL−1, respectively (Figure 2B). There was an increase of 25% and 18% in leaf yield with B. subtilis at 15.6 × 103 and 31.2 × 103 CFU mL−1 compared to non-inoculated plants, respectively. There was also an increase of 20% and 19% in the number of leaves at 15.6 × 103 and 31.2 × 103 CFU mL−1 compared to non-inoculated plants, respectively. A 15%, 22%, and 25% increase in shoot fresh matter with inoculation at 7.8 × 103, 15.6 × 103, and 31.2 × 103 CFU mL−1 compared to non-inoculated treatment was found. Both traits are closely related to improving yield efficiency (Figures 2A–C).
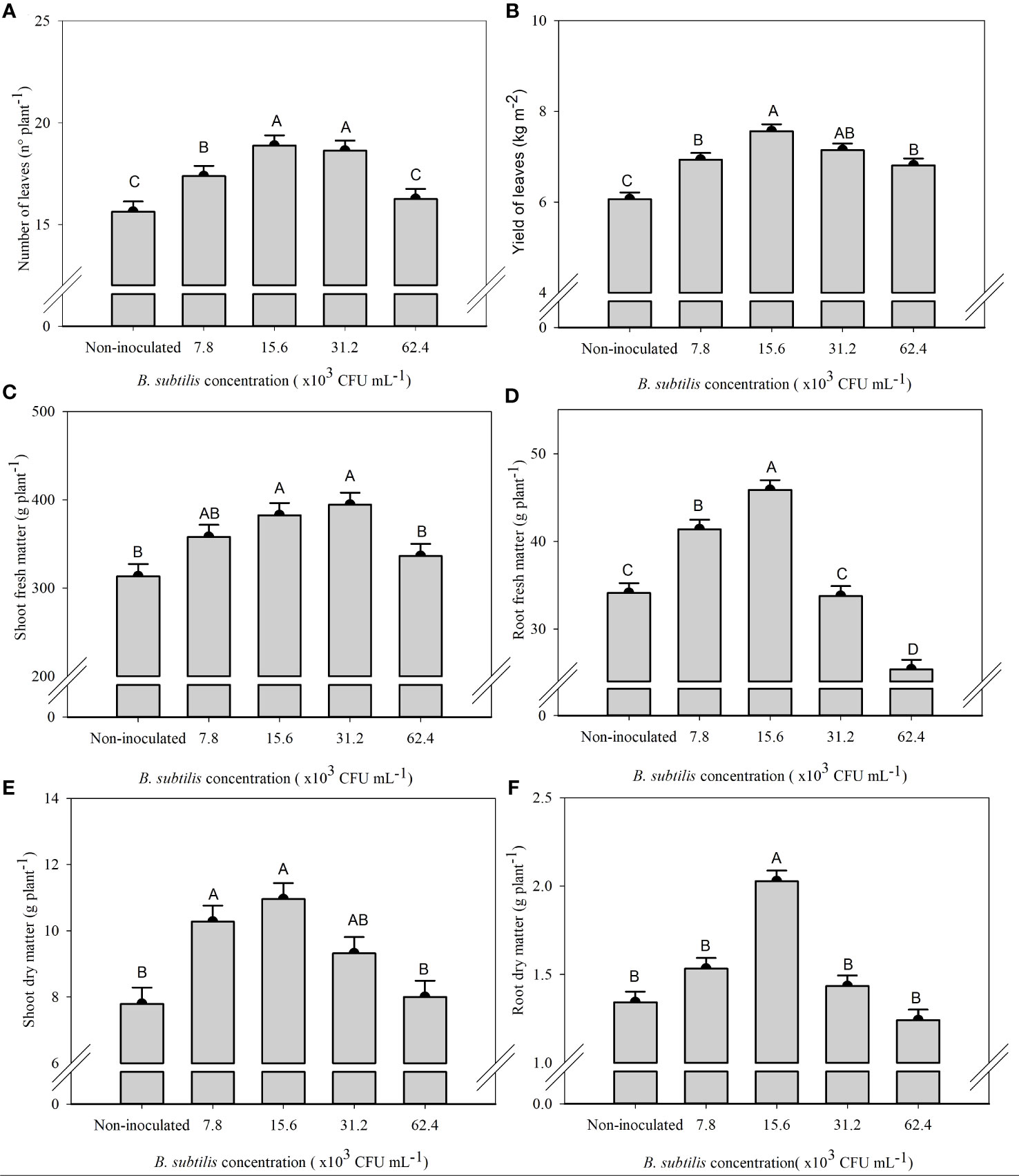
Figure 2 Number of leaves (A), yield of fresh leaves (B), fresh mass of shoot (C) and roots (D), and dry mass of shoot (E) and roots (F) of lettuce plants in the hydroponic NFT system according to the concentrations of Bacillus subtilis in the nutrient solution. Different capital letters in bars are statistically different by Tukey’s test at probability ofp ≤ 0.05. Error bars indicate the standard deviation of the mean (n = 5).
The highest root fresh and dry matter (45.87 and 2,02 g plant−1) occurred with the inoculation with B. subtilis at 15.6 × 103 CFU mL−1, which corresponded to an increase of 34% and 51% compared to non-inoculated treatment, respectively (Figures 2D, F). The highest shoot dry matter occurred at 7.8 × 103, 15.6 × 103, and 31.2 × 103 CFU mL−1 with an accumulation of 10.27, 10.95, and 9.32 g plant−1, approximately 31%, 40%, and 20% higher than non-inoculated plants, respectively (Figure 2E).
3.2 Plant physiology
There was a significant effect of B. subtilis on the intercellular CO2 concentration (Ci), stomatal conductance (gs), net photosynthesis rate (A), transpiration (E), water use efficiency (WUE), and total chlorophyll content (ChlT) (Supplementary Table 2).
The highest means of A, Ci, and WUE were 16.42 µmol CO2 m−2 s−1, 388.25 µmol CO2 mol−1 air−1, and 1.61 mmol CO2 mol−1 H2O−1 at a B. subtilis concentration of 31.2 × 103 CFU mL−1, with an increase of 95% in A, 30% in Ci, and 67% in WUE compared to the non-inoculated treatment, respectively (Figures 3A, D, E). The highest means of gs, 669.62, 738.50, and 747.62 mmol H2O m−2 s−1, were observed at 7.8 × 103, 15.6 × 103, and 31.2 × 103 CFU mL−1, with an increase of 33%, 46%, and 48% of gs compared to non-inoculated plants, respectively (Figure 3C). The highest E was 10.04, 10.60, and 10.26 mmol of H2O m−2 s−1, obtained at B. subtilis concentrations of 7.8 × 103, 15.6 × 103, and 31.2 × 103 CFU mL−1, equivalent to an increase of 14%, 20%, and 17% compared to non-inoculated treatment, respectively (Figure 3B). The highest total chlorophyll concentration (ChlT) was 1.47 mg g−1 FW, obtained at 15.6 × 103 CFU mL−1, approximately 54% higher than non-inoculated plants (Figure 3F).
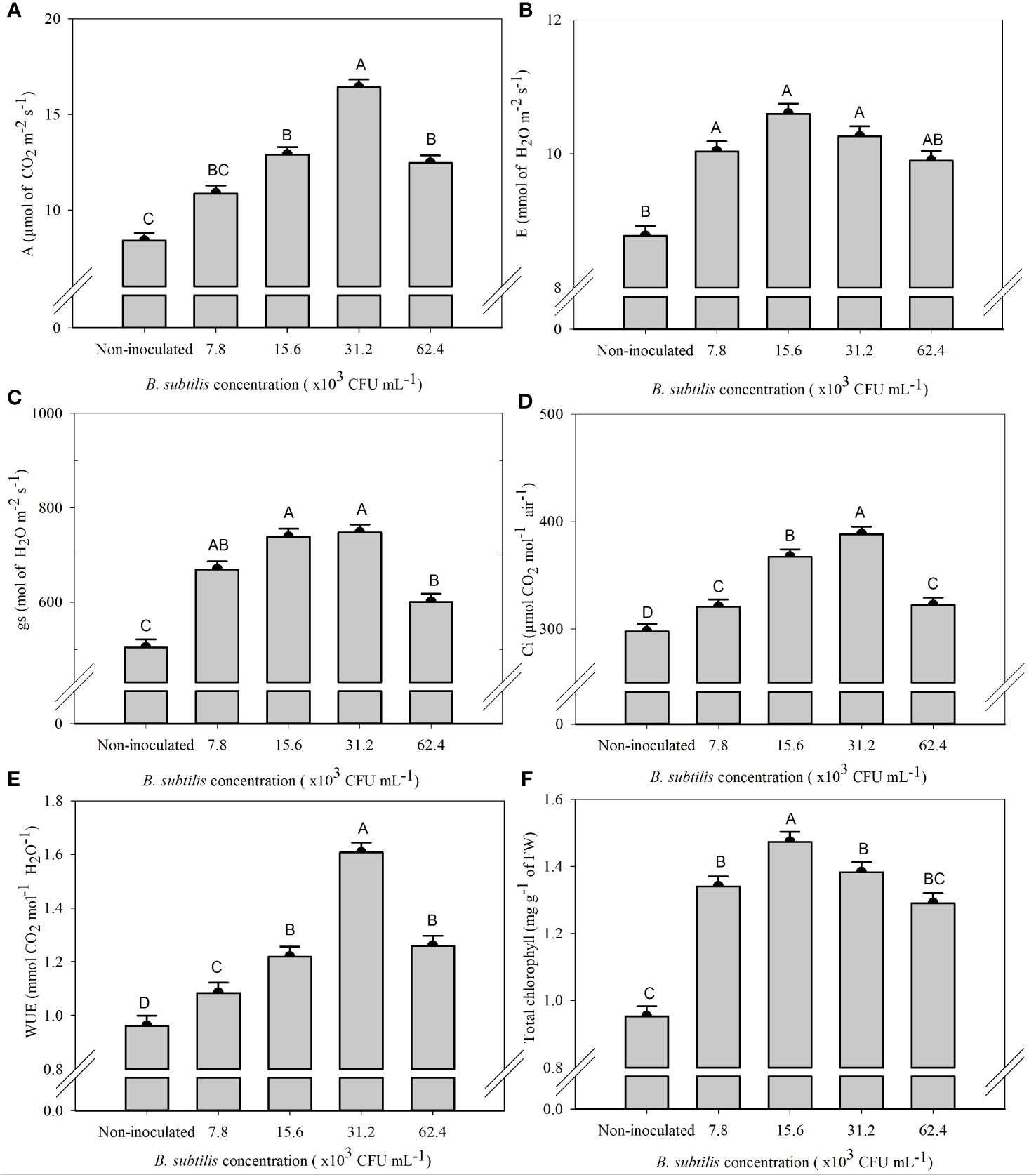
Figure 3 Net photosynthesis rate—A (A), transpiration—E (B), stomatal conductance—gs (C), intercellular CO2 concentration—Ci (D), water use efficiency—WUE (E), and total chlorophyll content—ChlT (F) of lettuce plants in the hydroponic NFT system according to the concentrations of Bacillus subtilis in the nutrient solution. Different capital letters in bars are statistically different by Tukey’s test at probability ofp ≤ 0.05. Error bars indicate the standard deviation of the mean (n = 5).
3.3 Nutrient accumulation in lettuce plants
The supply of N in the treatment without inoculation was greater than the accumulation of N in all plants cultivated in this treatment, demonstrating the low use efficiency of nitrogen fertilizers. On the other hand, under the inoculation with B. subtilis, there was an increase in the acquisition of N by plants of 25% and 36% at B. subtilis concentrations of 7.8 × 103 and 15.6 × 103 CFU mL−1 compared to the non-inoculated treatment, respectively (Supplementary Figure 1). The increment of B. subtilis concentrations significantly affected the accumulation of N, P, K, Ca, Mg, and S in the shoot and roots (Supplementary Table 3).
The highest N accumulation in the shoot was 8.18, 8.60, and 8.20 g m−2 at 7.8 × 103, 15.6 × 103, and 31.2 × 103 CFU mL−1, with an increase of 24%, 30%, and 24% compared to non-inoculated plants, respectively (Figure 4A). P accumulation in the shoot was the highest at 7.8 × 103, 15.6 × 103, and 31.2 × 103 CFU mL−1, with 1.91, 1.81, and 1.70 g m−2 of P, equivalent to an increase of 40%, 33%, and 25% compared to the non-inoculated treatment, respectively (Figure 4B). The concentration of 7.8 × 103 CFU mL−1 provided the highest accumulation of Ca (5.45 g m−2) and Mg (1.33 g m−2), approximately 109% and 74% higher than non-inoculated treatment, respectively (Figures 4D, E). The concentrations of 7.8 × 103 and 15.6 × 103 CFU mL−1 provided the highest accumulation of K (12.01 and 11.29 g m−2), approximately 53% and 44% higher than the non-inoculated treatment, and S (0.85 and 0.77 g m−2), approximately 73% and 47% higher than the non-inoculated treatment, respectively (Figures 4C, F).
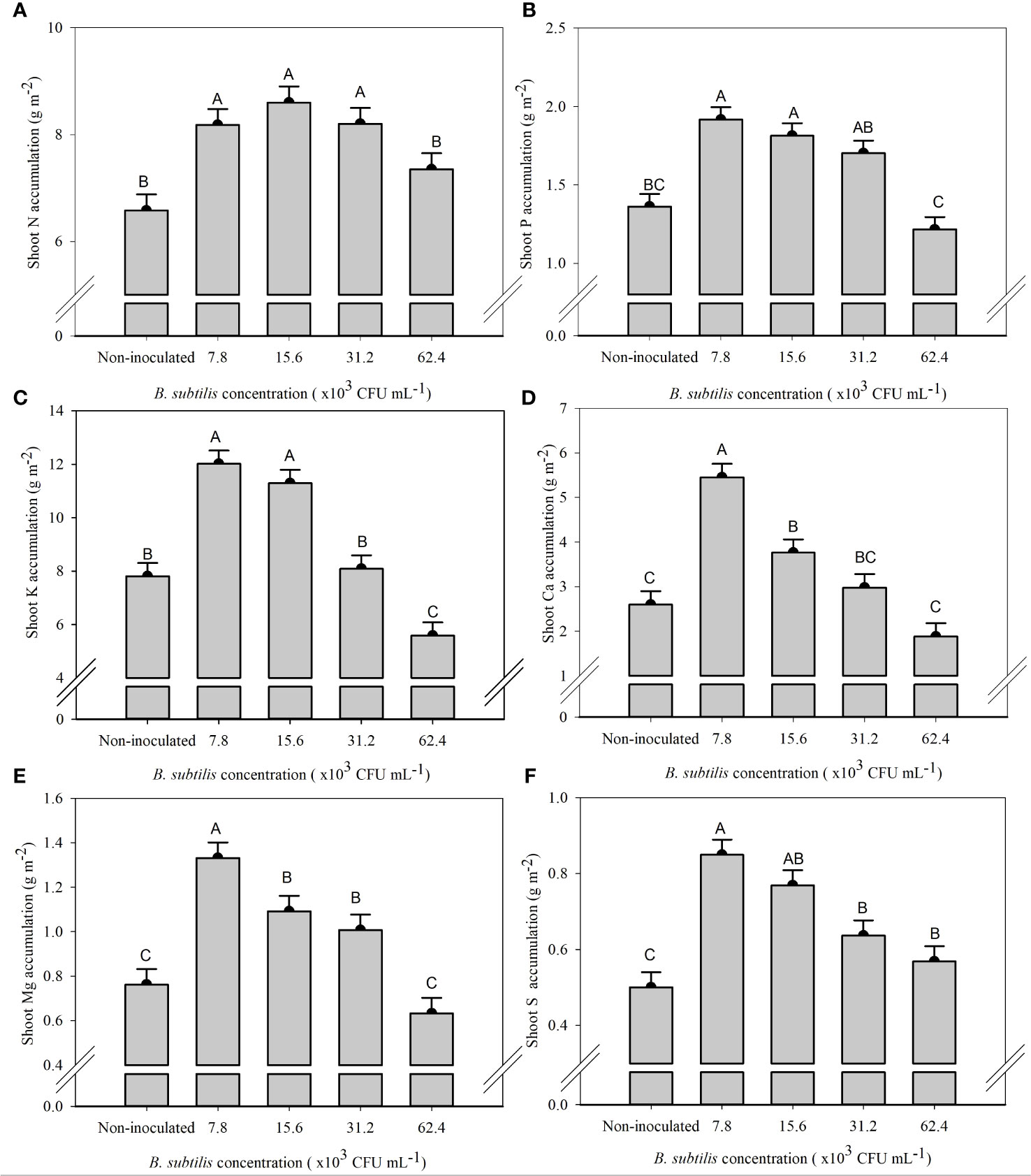
Figure 4 Accumulations of nitrogen (A), phosphorus (B), potassium (C), calcium (D), magnesium (E), and sulfur (F) in the shoot of lettuce plants in the hydroponic NFT system according to the concentrations of Bacillus subtilis in the nutrient solution. Different capital letters in bars are statistically different by Tukey’s test at probability ofp ≤ 0.05. Error bars indicate the standard deviation of the mean (n = 5).
The B. subtilis concentration of 15.6 × 103 CFU mL−1 provided the highest accumulation of N, P, K, Mg, and S in the roots, with means of 1.15, 0.37, 1.11, 0.36, and 0.37 g m−2, and an increase of 89%, 34%, 77%, 60%, and 87% compared to non-inoculated treatment, respectively (Figures 5A–C, E, F). The Ca accumulation in the roots was 0.43 and 0.47 g m−2 at 7.8 × 103 and 15.6 × 103 CFU mL−1, with an increase of 31% and 44% in Ca accumulation of root compared to the non-inoculated (Figure 5D).
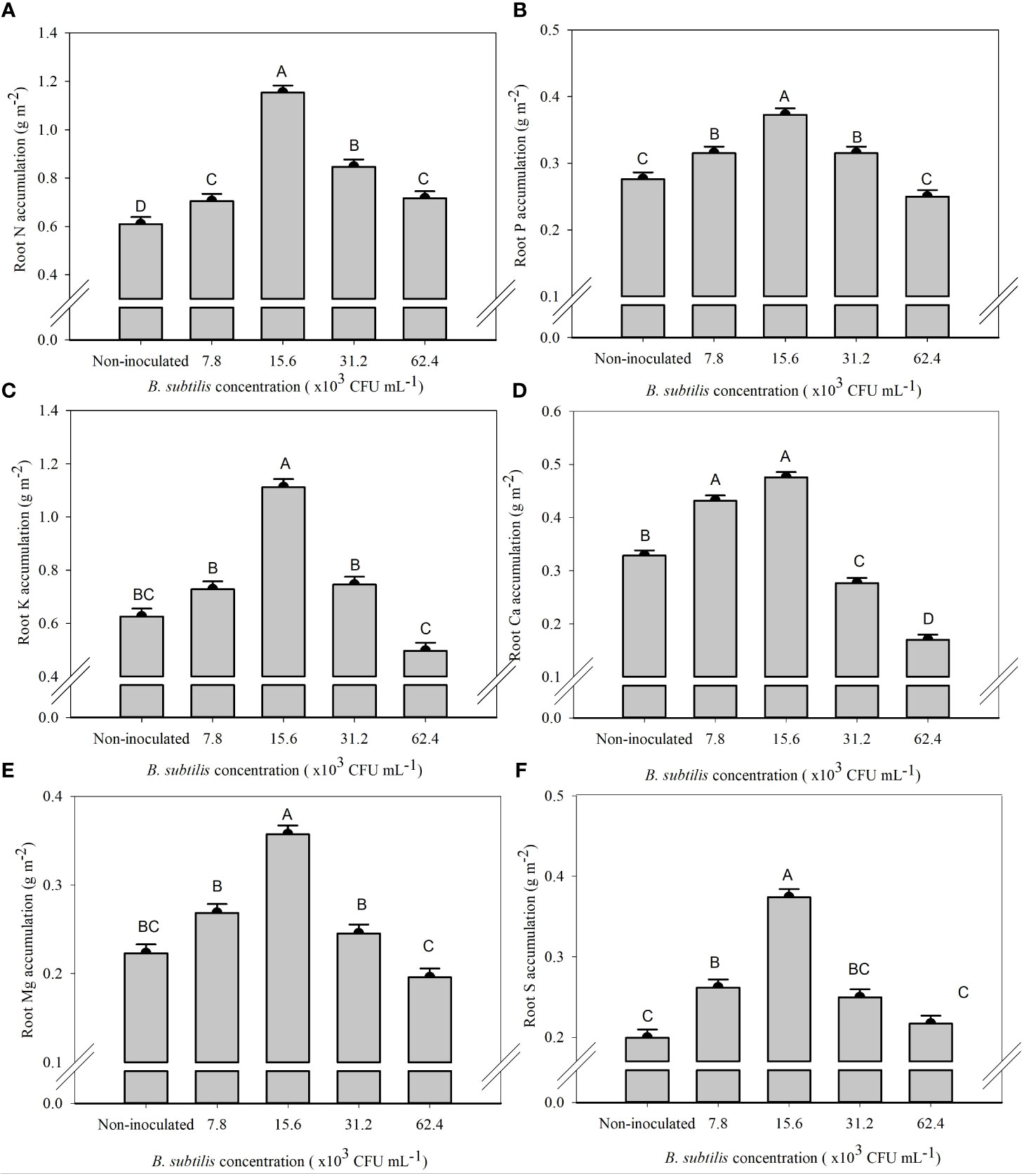
Figure 5 Accumulations of nitrogen (A), phosphorus (B), potassium (C), calcium (D), magnesium (E), and sulfur (F) in the roots of lettuce plants in the hydroponic NFT system according to the concentrations of Bacillus subtilis in the nutrient solution. Different capital letters in bars are statistically different by Tukey’s test at probability ofp ≤ 0.05. Error bars indicate the standard deviation of the mean (n = 5).
4 Discussion
The increase in root growth due to inoculation with the genus Bacillus in soil cultivation may be explained by several mechanisms including an increase in the biosynthesis of phytohormones and organic compounds, increased availability of nutrients, and the ability to colonize the root system of the plant (Tsotetsi et al., 2022). In our studies, in a hydroponic system, these mechanisms may explain the increase in fresh and dry matter of roots at a B. subtilis concentration of 15.6 × 103 CFU mL−1 compared to the non-inoculated plants, respectively (Figures 2D, F). Similar results were verified in arugula plants inoculated with 10 mL 100 L−1 of B. subtilis in a hydroponic system, with a 31% increase in fresh and dry matter of arugula plants (Oliveira et al., 2023b). The greater root growth with inoculation of B. subtilis occurred by stimulating the increase in the production of phytohormones such as indole-3-acetic acid (IAA) by the plants, which act mainly as stimulators of root growth and, consequently, improve the exploitation of the environment of cultivation and absorption of water and nutrients (Kumari et al., 2018).
The highest shoot fresh mass, number of leaves, and fresh leaf yield of lettuce plants were noted at B. subtilis concentrations of 15.6 × 103 and 31.2 × 103 CFU mL−1 (Figures 2A–C). It has been reported that fresh mass of lettuce is one of the most important marketable traits that increase profitability (Dalastra et al., 2020; Oliveira et al., 2023c). In other studies, inoculation via nutrient solution at 10 mL 100 L−1 of A. brasilense provided an increase of 12% and 20% in the number of leaves and 23% and 32% in the leaf yield of lettuce and arugula plants, respectively (Oliveira et al., 2023a). Similar results were verified in arugula plants inoculated with 10 mL 100 L−1 of B. subtilis in a hydroponic system (Oliveira et al., 2023b). Inoculation with B. subtilis favors the increase in the shoot fresh and dry matter by increasing photosynthetic efficiency, carbon assimilation, and nutrient acquisition by the roots (Fonseca et al., 2022).
Leaf growth occurs with higher net assimilation of CO2 in the photosynthetic process that allows the formation of new leaves and an increase in plant mass, resulting in higher yield (Fonseca et al., 2022). The present results stated that inoculation with B. subtilis at a concentration of 31.2 × 103 CFU mL−1 increased A, Ci, and WUE, while ChlT of lettuce leaves increased at a concentration of 15.6 × 103 CFU mL−1 (Figures 3A, D–F). The higher water use efficiency is related to the greater integrity of leaf cell membranes, which is related to the ability of plants to keep their metabolic and photosynthetic activities in operation even in periods with high temperatures, as in the case of the present study (Figure 1). The greater water use efficiency provides greater turgor in the guard cells, keeping the stomata open. The gs was higher at B. subtilis concentrations of 15.6 × 103 and 31.2 × 103 CFU mL−1 compared to non-inoculated plants (Figure 3C). The suitable water status of plant leaves can increase stomatal opening periods, which favors photosynthetic activity and net CO2 assimilation by plants, and can improve the efficiency of water use and plant growth (Buckley, 2019).
An adequate supply of B subtilis led to increased growth of lettuce shoots and roots via enhanced nutrient uptake. At inoculation concentrations ranging from 7.6 to 31.2 × 103 CFU mL−1, increased accumulation of N, P, Ca, Mg, K, and S (depending on the individual nutrient) was observed in the shoot and roots of lettuce as compared to non-inoculated plants (Supplementary Table 3; Figures 4, 5).
The increase in the efficiency of nutrient uptake and transport by plants inoculated with B. subtilis is mainly related to increased root growth, production of siderophores, and production of secondary compounds and phytohormones in the plant (Qiao et al., 2017; Fonseca et al., 2022). Root growth also favors the increased absorption efficiency of N, P, K, Ca, Mg, and S, and the gibberellin production as previously reported in Chinese cabbage plants under inoculation with B. subtilis compared to non-inoculated plants (Kang et al., 2019). The increase in the accumulation of these nutrients was also observed in sugarcane under inoculation with B. subtilis (Fonseca et al., 2022). Other research with foliar inoculation with T. harzianum and A. brasilense in lettuce and arugula in the hydroponic system provided greater root growth and, consequently, greater acquisition of nutrients by the plants, in addition to providing less use of fertilizers (Moreira et al., 2022; Oliveira et al., 2022; Gato et al., 2023). Inoculation with B. subtilis via nutrient solution in arugula plants provided an increase of 6%, 43%, 36%, 52%, and 45% in the accumulation of N, K, Ca, Mg, and S in the shoots, respectively, and provided an increase of 32%, 107%, 6%, 65%, and 19% in the accumulation of N, P, K, Ca, and S in the roots, respectively (Oliveira et al., 2023b).
There was increase in ChlT content with the inoculation of B. subtilis at 15.6 × 103 CFU mL−1 (Figure 3F). Photosynthetic CO2 assimilation rates are favored by adequate K and Mg nutrition, increasing intercellular CO2 levels and leaf photosynthetic activity (Singh and Reddy, 2017). Because of the osmotic role of K in increasing leaf stomatal conductance (acting in guard cell regulation), lower K uptake impairs photosynthesis (Jákli et al., 2017). Plant growth and metabolism require the translocation of carbohydrates from photosynthetically active tissues; sucrose loading is the main form of carbohydrate transport in plants, and K and Mg deficiency in the phloem can impair the efficient transport of carbon to within the plant, and adequate supply of these two nutrients enhances photoprotection (Tränkner et al., 2018). Ca2+ binding proteins are chloroplast residents, but some are located in the chloroplast membrane, such as the transporter-like S-adenosylmethionine (Stael et al., 2011). Ca2+ acts as a cofactor for the activation of redox enzymes related to photosystem II membranes; Ca2+ also regulates the enzymes fructose-1,6-bisphosphatase (FBPase) and sedoheptulose-1,7-bisphosphatase (SBPase) that are fundamental in the Calvin cycle in the carbon assimilation pathway that occurs in the chloroplast stroma (Wang et al., 2019).
The use of inoculation with B. subtilis has been highlighted as promoting plant growth, increasing crop yield, and increasing efficiency in nitrogen acquisition. The main mechanisms related to greater nitrogen acquisition caused by B. subtilis are greater root growth (increases soil exploitation by roots), growth promotion (increased production of secondary compounds and phytohormones), and biological nitrogen fixation (increases nitrogen metabolism activity and yield by increasing carbon accumulation) (Hashem et al., 2019). At B. subtilis concentrations of 7.8 × 103 and 15.6 × 103 CFU mL−1, there was higher N accumulation than in the non-inoculated treatment (Supplementary Figure 1), which may occur due to the effect of biological N fixation by B. subtilis since the only nitrogen source available for the roots in the hydroponic system is from the nutrient solution. Inoculation with B. subtilis in the nutrient solution may allow a symbiotic interaction with the plant, permitting B. subtilis to sequester N from the atmosphere via nitrogen fixation and provide it to the plant. In studies with several species of Bacillus, the presence of the nif and fixam genes, responsible for the ability to perform nitrogen fixation, were confirmed by genome sequencing. In addition, these Bacillus species increased biological N fixation in a tropical region (Yousuf et al., 2017). B. subtilis provides greater nitrogen use efficiency and mitigation of ammonia emission to the environment due to the ease of acquisition of ammonium molecules derived by bacteria during the nitrogen fixation process and its transport to plant tissues (Sun et al., 2020). In addition, according to Gunka and Commichau (2012), inoculation with B. subtilis increased the nrgA gene expression related to the uptake of N by plants in the ammoniacal form, inducing a greater amount of ammonium available through the process of biological N fixation.
Inoculation with B. subtilis increased plant mass accumulation, nutrient accumulation, and photosynthetic and water use efficiency. The main mechanisms of B. subtilis to improve the growth, nutrition, and leaf gas exchange of hydroponic lettuce plants are related to increased efficiency in nutrient uptake and photosynthesis. In addition, it was possible to verify alterations in the nutrient solution, raising the hypothesis that there is an interaction between B. subtilis and roots in the hydroponic system, resulting in biological nitrogen fixation. This hypothesis will require further research to confirm the presence of nitrogen fixation genes in this B. subtilis strain and their expression under hydroponic growth conditions with lettuce. However, increasing lettuce leaf yield with B. subtilis inoculation is a low-cost technology that reduces fertilizer consumption and increases farmer profitability.
5 Conclusions
The B. subtilis concentration at 15.6 × 103 and 31.2 × 103 CFU mL−1 in the nutrient solution is indicated to achieve the highest yield efficiency in the hydroponic system since it provided the highest lettuce fresh leaf yield, shoot fresh matter, and number of leaves. B. subtilis inoculation at 15.6 × 103 CFU mL−1 increased root fresh and dry mass in lettuce plants grown in the hydroponic system.
Inoculation with B. subtilis at 7.8 × 103 CFU mL−1 in the nutrient solution increased the accumulation of Ca, Mg, and S in the shoot, and at 7.8 × 103 and 15.6 × 103 CFU mL−1, it increased K accumulation in the shoot. B. subtilis at 7.8 × 103, 15.6 × 103, and 31.2 × 103 CFU mL−1 increased N accumulation in the shoot in the hydroponic system. In addition, the inoculation with B. subtilis at 15.6 × 103 CFU mL−1 increased the accumulation of N, P, K, Mg, and S in the roots of lettuce plants.
B. subtilis at 31.2 × 103 CFU mL−1 increased water use efficiency, intercellular CO2 concentration, and net photosynthesis rate of lettuce plants in the hydroponic system. B. subtilis at 15.6 × 103 and 31.2 × 103 CFU mL−1 increased stomatal conductance. Also, the highest total chlorophyll content was found at 15.6 × 103 CFU mL−1 in the lettuce plants grown in a hydroponic system.
Data availability statement
The original contributions presented in the study are included in the article/Supplementary Materials. Further inquiries can be directed to the corresponding author.
Author contributions
CO and MT conceived and designed the research. CEO and JA carried out the experiment. CO and MT analyzed the data. CO wrote the manuscript. BG, MA-M, KA, and HA contributed to formal analysis and data curation. AJ, LC, and MT reviewed and translated the manuscript. All authors contributed to the article and approved the submitted version.
Funding
The author(s) declare financial support was received for the research, authorship, and/or publication of this article. This research was funded by the Fundação de Amparo à Pesquisa do Estado de São Paulo (FAPESP, grant number: 2020/11621-0). The Conselho Nacional de Desenvolvimento Científico e Tecnológico (CNPq) granted the doctoral fellowship for the first author (CNPq/TWAS grant number: 166331/2018-0) and the research productivity fellowship (award number: 311308/2020-1) for the corresponding author. This study was supported by the ECOTWINS [Research Capacity Building and Upskilling and Upgrading the Research Team in NUBiP (Ukraine) on Agroecological Intensification for Crop Production] project. ECOTWINS has received funding from the Horizon Europe Framework Programme (HORIZON) under grant agreement number 101079308 and Researchers Supporting Project number (RSPD2023R725) King Saud University, Riyadh, Saud Arabia.
Acknowledgments
The authors acknowledge the São Paulo Research Foundation, FAPESP, process number 2020/11621-0, Coordination for the Improvement of Higher Education Personnel Foundation (CAPES), and Horizon Europe Framework Programme (HORIZON) under grant agreement number 101079308, and CNPq productivity research grant (award number 311308/2020-1) of the last author. The authors thank the laboratories of plant nutrition (UNESP/FEIS), plant morphology and anatomy (UNESP/FEIS), and plant metabolism and physiology (UNESP/FEIS). The authors also acknowledge the seedling nursery AGROMUDAS for the seedlings provided. The authors extend their appreciation to the Researchers Supporting Project number (RSPD2023R725) King Saud University, Riyadh, Saud Arabia.
Conflict of interest
The authors declare that the research was conducted in the absence of any commercial or financial relationships that could be construed as a potential conflict of interest.
Publisher’s note
All claims expressed in this article are solely those of the authors and do not necessarily represent those of their affiliated organizations, or those of the publisher, the editors and the reviewers. Any product that may be evaluated in this article, or claim that may be made by its manufacturer, is not guaranteed or endorsed by the publisher.
Supplementary material
The Supplementary Material for this article can be found online at: https://www.frontiersin.org/articles/10.3389/fpls.2023.1248044/full#supplementary-material
References
Aini, N., Yamika, W. S. D., Ulum, B. (2019). Effect of nutrient concentration, PGPR and AMF on plant growth, yield and nutrient uptake of hydroponic lettuce. Intl. J. Agric. Biol. 21, 175–183. doi: 10.17957/IJAB/15.0879
Bettiol, W., Kupper, K. C., Goes, A., Moretto, C., Correa, E. B. (2005). Mass production of Bacillus subtilis and Trichoderma viride for the control of Phyllosticta citricarpa (teleomorph: Guignardia citricarpa). Summa. Phytopatol. 31, 276–278.
Buckley, T. N. (2019). How do stomata respond to water status? New Phytol. 224, 21–36. doi: 10.1111/nph.15899
Dalastra, C., Teixeira Filho, M. C. M., Silva, M. R., Nogueira, T. A. R., Fernandes, G. C. (2020). Head lettuce production and nutrition in relation to nutrient solution flow. Hortic. Bras. 38, 21–26. doi: 10.1590/S0102-053620200103
Fonseca, M. C., Bossolani, J. W., de Oliveira, S. L., Moretti, L. G., Portugal, J. R., Scudeletti, D., et al. (2022). Bacillus subtilis inoculation improves nutrient uptake and physiological activity in sugarcane under drought stress. Microorganisms 10, 809. doi: 10.3390/microorganisms10040809
Fukami, J., Cerezini, P., Hungria, M. (2018). Azospirillum: benefits that go far beyond biological nitrogen fixation. AMB Expr. 8, 73. doi: 10.1186/s13568-018-0608-1
Gato, I. M. B., Oliveira, C. E. S., Oliveira, T. J. S. S., Jalal, A., Moreira, V. A., Giolo, V. M., et al. (2023). Nutrition and yield of hydroponic arugula under inoculation of beneficial microorganisms. Hortic. Environ. Biotechnol. 63, 1–12. doi: 10.1007/s13580-022-00476-w
Gunka, K., Commichau, F. M. (2012). Control of glutamate homeostasis in Bacillus subtilis: a complex interplay between ammonium assimilation, glutamate biosynthesis and degradation. Molec. Microbiol. 85, 213–224. doi: 10.1111/j.1365-2958.2012.08105.x
Hashem, A., Tabassum, B., Abd Allah, F. E. (2019). Bacillus subtilis: A plant-growth promoting rhizobacterium that also impacts biotic stress. Saudi J. Biol. Sci. 26, 1291–1297. doi: 10.1016/j.sjbs.2019.05.004
Hiscox, J., Israelstam, G. F. (1979). A method for the extraction of chlorophyll from leaf tissue without maceration. Can. J. Bot. 57, 1332–1334. doi: 10.1139/b79-163
Jákli, B., Tavakol, E., Tränkner, M., Senbayram, M., Dittert, K. (2017). Quantitative limitations to photosynthesis in K deficient sunflower and their implications on water-use efficiency. J. Plant Physiol. 209, 20–30. doi: 10.1016/j.jplph.2016.11.010
Kang, S. M., Hamayun, M., Khan, M. A., Iqbal, A., Lee, I. J. (2019). Bacillus subtilis JW1 enhances plant growth and nutrient uptake of Chinese cabbage through gibberellins secretion. J. Appl. Bot. Food Qual. 92, 172–178. doi: 10.5073/JABFQ.2019.092.023
Kappel, N., Boros, I. F., Ravelombola, F. S., Sipos, L. (2021). EC sensitivity of hydroponically grown lettuce (Lactuca sativa L.) types in terms of nitrate accumulation. Agriculture 11, 315. doi: 10.3390/agriculture11040315
Kasozi, N., Kaiser, H., Wilhelmi, B. (2021). Effect of bacillus spp. on lettuce growth and root associated bacterial community in a small-scale aquaponics system. Agronomy 11, 947. doi: 10.3390/agronomy11050947
Kim, M. J., Moon, Y., Tou, J. C., Mou, B., Waterland, N. L. (2016). Nutritional value, bioactive compounds and health benefits of lettuce (Lactuca sativa L.). J. Food Composition Anal. 49, 19–34. doi: 10.1016/j.jfca.2016.03.004
Kumari, S., Prabha, C., Singh, A., Kumari, S., Kiran, S. (2018). Optimization of Indole-3-Acetic Acid Production by Diazotrophic B. subtilis DR2 (KP455653), Isolated from Rhizosphere of Eragrostis cynosuroides. Int. J. Pharma Med. Biol. Sci. 7, 20–27. doi: 10.18178/ijpmbs.7.2.20-27
Malavolta, E., Vitti, G. C., Oliveira, S. A. (1997). Assessment of the nutritional status of plants: principles and applications. 2nd edn (Piracicaba: Brazilian Association for the Research of Potash and Phosphate).
Moreira, V. D. A., Oliveira, C. E. D. S., Jalal, A., Gato, I. M. B., Oliveira, T. J. S. S., Boleta, G. H. M., et al. (2022). Inoculation with Trichoderma harzianum and Azospirillum brasilense increases nutrition and yield of hydroponic lettuce. Arch. Microbiol. 204, e440. doi: 10.1007/s00203-022-03047-w
Oliveira, C. E. S., Gato, I. M. B., Moreira, V. A., Jalal, A., Oliveira, T. J. S. S., Oliveira, J. R., et al. (2023a). Inoculation methods of Azospirillum brasilense in lettuce and arugula in the hydroponic system. Rev. Bras. Eng. Agr. Amb. 27, 653–662. doi: 10.1590/1807-1929/agriambi.v27n9p653-662
Oliveira, C. E. S., Jalal, A., Oliveira, J. R., Tamburi, K. V., Teixeira Filho, M. C. M. (2022). Leaf inoculation of Azospirillum brasilense and Trichoderma harzianum in hydroponic arugula improve productive components and plant nutrition and reduce leaf nitrate. Pesquisa Agropecuária Trop. 52, e72755, 1–11. doi: 10.1590/1983-40632022v5272755
Oliveira, C. E. S., Jalal, A., Vitória, L. S., Giolo, V. M., Oliveira, T. J. S. S., Aguilar, J. V., et al. (2023c). Inoculation with Azospirillum brasilense Strains AbV5 and AbV6 increases nutrition, chlorophyll, and leaf yield of hydroponic lettuce. Plants 12, 3107. doi: 10.3390/plants12173107
Oliveira, T. J. S. S., Oliveira, C. E. S., Jalal, A., Gato, I. M. B., Rauf, K., Moreira, V. A., et al. (2023b). Inoculation reduces nitrate accumulation and increases growth and nutrient accumulation in hydroponic arugula. Sci. Hortic. 320, 112213. doi: 10.1016/j.scienta.2023.112213
Opatovsky, I., Elbaz, M., Dori, I., Avraham, L., Mordechai-Lebiush, S., Dombrovsky, A., et al. (2019). Control of lettuce big-vein disease by application of fungicides and crop covers. Plant Pathol. 68, 790–795. doi: 10.1111/ppa.12990
Qiao, J., Yu, X., Liang, X., Liu, Y., Borriss, R., Liu, Y. (2017). Addition of plant-growth-promoting Bacillus subtilis PTS-394 on tomato rhizosphere has no durable impact on composition of root microbiome. BMC Microbiol. 17, 131. doi: 10.1186/s12866-017-1039-x
Singh, S. K., Reddy, V. R. (2017). Potassium starvation limits soybean growth more than the photosynthetic processes across CO2 levels. Front. Plant Sci. 8. doi: 10.3389/fpls.2017.00991
Stael, S., Rocha, A. G., Robinson, A. J., Kmiecik, P., Vothknecht, U. C., Teige, M. (2011). Arabidopsis calcium-binding mitochondrial carrier proteins as potential facilitators of mitochondrial ATP-import and plastid SAM-import. FEBS Lett. 585, 3935. doi: 10.1016/j.febslet.2011.10.039
Sun, B., Bai, Z., Bao, L., Xue, L., Zhang, S., Wei, Y., et al. (2020). Bacillus subtilis biofertilizer mitigating agricultural ammonia emission and shifting soil nitrogen cycling microbiomes. Environ. Int. 144, 105989. doi: 10.1016/j.envint.2020.105989
Tränkner, M., Tavakol, E., Jákli, B. (2018). Functioning of potassium and magnesium in photosynthesis, photosynthate translocation and photoprotection. Physiol. Plant 163, 414–431. doi: 10.1111/ppl.12747
Tsotetsi, T., Nephali, L., Malebe, M., Tugizimana, F. (2022). Bacillus for plant growth promotion and stress resilience: What have we learned? Plants 11, 2482. doi: 10.3390/plants11192482
Wang, Q., Yang, S., Wan, S., Li, X. (2019). The significance of calcium in photosynthesis. Int. J. Mol. Sci. 20, 1353. doi: 10.3390/ijms20061353
Yang, X., Gil, M. I., Yang, Q., Tomás-Barberán, F. A. (2022). Bioactive compounds in lettuce: Highlighting the benefits to human health and impacts of preharvest and postharvest practices. Compr. Rev. Food. Sci. Food Saf. 21, 4–45. doi: 10.1111/1541-4337.12877
Keywords: biological nitrogen fixation, growth-promoting bacteria, Lactuca sativa L., photosynthetic efficiency, water use efficiency
Citation: Oliveira CEdS, Jalal A, Aguilar JV, de Camargos LS, Zoz T, Ghaley BB, Abdel-Maksoud MA, Alarjani KM, AbdElgawad H and Teixeira Filho MCM (2023) Yield, nutrition, and leaf gas exchange of lettuce plants in a hydroponic system in response to Bacillus subtilis inoculation. Front. Plant Sci. 14:1248044. doi: 10.3389/fpls.2023.1248044
Received: 26 June 2023; Accepted: 06 October 2023;
Published: 25 October 2023.
Edited by:
Louise Mary Nelson, University of British Columbia, CanadaReviewed by:
Roxana Vidican, University of Agricultural Sciences and Veterinary Medicine of Cluj-Napoca, RomaniaLuz E. Bashan, Bashan Institute of Science, United States
Copyright © 2023 Oliveira, Jalal, Aguilar, de Camargos, Zoz, Ghaley, Abdel-Maksoud, Alarjani, AbdElgawad and Teixeira Filho. This is an open-access article distributed under the terms of the Creative Commons Attribution License (CC BY). The use, distribution or reproduction in other forums is permitted, provided the original author(s) and the copyright owner(s) are credited and that the original publication in this journal is cited, in accordance with accepted academic practice. No use, distribution or reproduction is permitted which does not comply with these terms.
*Correspondence: Marcelo Carvalho Minhoto Teixeira Filho, mcm.teixeira-filho@unesp.br
†ORCID: Carlos Eduardo da Silva Oliveira, orcid.org/0000-0002-3894-9559
Arshad Jalal, orcid.org/0000-0002-9451-0508
Jailson Vieira Aguilar, orcid.org/0000-0003-3684-9180
Liliane Santos de Camargos, orcid.org/0000-0002-0979-4447
Tiago Zoz, orcid.org/0000-0003-2991-5485
Marcelo Carvalho Minhoto Teixeira Filho, orcid.org/0000-0003-2303-3465