- 1Key Laboratory of Plant Resource Conservation and Germplasm Innovation in Mountainous Region (Ministry of Education), School of Liquor and Food Engineering, Guizhou University, Guiyang, China
- 2National & Local Joint Engineering Research Center for the Exploitation of Homology Resources of Southwest Medicine and Food, Guizhou University, Guiyang, China
- 3First Affiliated Hospital of Guizhou University of Traditional Chinese Medicine, Guiyang, China
Alpinia coriandriodora, also known as sweet ginger, is a medicinal and edible plant. A. coriandriodora rhizome is popularly utilized in traditional Chinese medicine and as flavouring spices, but there are few reports on its constituents and bioactivities. This study analyzed the phytochemical components of A. coriandriodora rhizome by GC-MS and UHPLC-Q-Orbitrap-MS and evaluated its antioxidant, antimicrobial, and anti-enzymatic properties. According to the GC-FID/MS data, its rhizome essential oil (EO) consisted mainly of (E)-2-decenal (53.8%), (E)-2-decenyl acetate (24.4%), (Z)-3-dodecenyl acetate (3.5%), and (E)-2-octenal (3.5%). Its water extract (WE) and 70% ethanol extract (EE) showed high total phenolic content (TPC, 52.99–60.49 mg GAEs/g extract) and total flavonoid content (TFC, 260.69–286.42 mg REs/g extract). In addition, the phytochemicals of WE and EE were further characterized using UHPLC-Q-Orbitrap-MS, and a total of sixty-three compounds were identified, including fourteen phenolic components and twenty-three flavonoid compounds. In the antioxidant assay, WE and EE revealed a potent scavenging effect on DPPH (IC50: 6.59 ± 0.88 mg/mL and 17.70 ± 1.15 mg/mL, respectively), surpassing the BHT (IC50: 21.83 ± 0.89 mg/mL). For the antimicrobial activities, EO displayed excellent antibacterial capabilities against Proteus vulgaris, Enterococcus faecalis, Bacillus subtilis, Escherichia coli, and Staphylococcus aureus with DIZ (12.60–22.17 mm), MIC (0.78–1.56 mg/mL), and MBC (3.13 mg/mL) and significantly inhibited Aspergillus flavus growth (MIC = 0.313 mg/mL, MFC = 0.625 mg/mL, respectively). In addition to weak tyrosinase and cholinesterase inhibition, EE and WE had a prominent inhibitory effect against α-glucosidase (IC50: 0.013 ± 0.001 mg/mL and 0.017 ± 0.002 mg/mL), which was significantly higher than acarbose (IC50: 0.22 ± 0.01 mg/mL). Hence, the rhizome of A. coriandriodora has excellent potential for utilization in the pharmaceutical and food fields as a source of bioactive substances.
1 Introduction
Alpinia (Zingiberaceae) has approximately 250 species and is mostly distributed in subtropical and tropical Asia (WFO, 2023). Alpinia is widely cultivated for its rhizome, which is consumed in fields of folk medicine, condiment, perfume, and food (Yang and Eilerman, 1999; Victório, 2011; Ibrahim et al., 2014). Alpinia plants are abundant in essential oil (EO) and are frequently used to treat cancer, ulcer, neural disorders, diabetes, and Alzheimer’s disease (Ghosh and Rangan, 2013; Rajasekar et al., 2014; Shi et al., 2015). Numerous bioactivities of the Alpinia plant’s essential oils and extract have been reported, including anti-inflammatory, antimicrobial, insecticidal, neuroprotective, antioxidant, antifungal, and analgesic activities (Ghosh and Rangan, 2013; Van et al., 2021).
Alpinia coriandriodora D. Fang., also known as sweet ginger, is a perennial plant with a distinctive coriander fragrance that is extensively cultivated in China due to its medicinal and edible value (Wu and Larsen, 2000; Cheng et al., 2021). A. coriandriodora rhizome is used as traditional Chinese medicine for treating fever, asthma, cold, stomachache, and indigestion (CHMC-Chinese Herbal Medicine Company, 1994; Zhang and Li, 2015; Wu et al., 2016). Moreover, its rhizome was utilized as spices in the preparation of soups and stews. Interestingly, wrapping zongzi with its leaves is a specialty of residents in Guangxi, China. In previous studies on A. coriandriodora rhizome, several diarylheptanoids and flavonoids were isolated, and these compounds had intracellular antioxidant and anti-inflammatory actions by inhibiting NO release (Cheng et al., 2021). A. coriandriodora is rich in essential oil, and its EO has been used in traditional medicine (Tran et al., 2022). Previous research indicated that A. coriandriodora rhizome EO was mainly composed of (E)-2-decenal (60.4–56.3%) and (E)-2-decenyl acetate (19.6–4.3%) and possessed potent anticancer and anti-malassezia effects (Hong et al., 2022; Tran et al., 2022).
Despite its high edible and medicinal value, there are few reports on the phytochemistry and biological activities of A. coriandriodora rhizome, which may hinder its utilization. Thus, this study analyzed the phytochemical constitution of A. coriandriodora rhizome using GC-FID/MS and UHPLC-Q-Orbitrap-MS and evaluated its antioxidant, antimicrobial, and anti-enzymatic properties.
2 Materials and methods
2.1 Chemical and reagents
Streptomycin, Folin-Ciocalteu reagent, resazurin, galanthamine, kojic acid, acarbose, rutin, and gallic acid were from Solarbio Life Sciences (Beijing, China). Sigma-Aldrich (Germany) provided BTCl (butyrylthiocholine chloride), ATCI (acetylthiocholine iodide), L-tyrosine, p-NPG (p-nitrophenyl-α-D-glucopyranoside), BChE (butyrylcholinesterase), AChE (acetylcholinesterase), tyrosinase, α-glucosidase, ABTS (2,2-azino-bis-3-ethylbenzthiazoline-6-sulphonic acid), DPPH (1,1-diphenyl-2-picrylhydrazyl), BHT (butylated hydroxytoluene), and ascorbic acid. Merck (Darmstadt, Germany) supplied a standard n-alkane (C7–C30) in the GC-MS test and formic acid and acetonitrile (LC/MS-grade) in the UHPLC-Q-Orbitrap-MS test. Aladdin (Shanghai, China) offered other solvents and chemicals in analytical purity.
2.2 Plant material
Alpinia coriandriodora D. Fang was cultivated at Guigang City, Guangxi Province, China (latitude: 23°80′89.74′′N and longitude: 110°18′64.68″E). The plant samples were harvested in August 2020 and authenticated by Professor Guoxiong Hu, a plant taxonomist. A voucher specimen has been deposited at the Herbarium of the College of Life Sciences, Guizhou University, China (Voucher No: AC2020803).
2.3 Extraction of EO, WE, and EE
Hydro-distillation was employed to prepare the EO of A. coriandriodora rhizome. In brief, the fresh rhizome (2 kg) was washed using distilled water, crushed, and hydrodistilled (4 h) in a Clevenger’s apparatus. After drying with anhydrous Na2SO4, EO was stored at 4°C until further use.
Reflux extraction was used to produce the WE and EE of the A. coriandriodora rhizome. The crushed plant material (0.5 kg) and 70% ethanol or distilled water (2 L) were added to a round-bottomed flask (5 L). Afterward, the mixture was reflux-extracted twice (2 h/reflux). The extraction solution was mixed, filtered, condensed using a rotary evaporator, and dried using a freeze drier. Then, WE and EE were weighed and stored in a brown glass bottle at 4°C until usage.
2.4 EO’s composition analysis using GC-FID/MS
Quantitative analysis of phytochemical components was performed on GC-FID (Agilent Technologies, 6890N) equipped with an HP-5MS column (60 m × 0.25 mm, 0.25 mm film thickness). The helium was used as a carrier gas (1 mL/min). The injection volumes were 1 µL (split ratio 1:20). The injector temperature was 250°C. The oven temperature was programmed as follows: kept at 70°C (2 min), raised to 180°C (2°C/min), increased to 310°C (10°C/min), and held at 310°C for 14 min (running time: 84 min). Agilent 6890/5975C GC-MS was employed for qualitative analysis, and its GC settings were the same as those of GC-FID. The following conditions were applied in MS: ion source (EI, 70 eV); ion source temperature (230°C); interface temperature (280°C); scan range from 29 to 500 m/z. The peak area revealed the relative percentage of different chemical constituents. A series of n-alkanes (C8–C16) were used to determine the retention index (RI). Matching the RI and MS information in Wiley 275 and NIST 2020 databases was done to identify the chemical constituents.
2.5 Determination of total phenolic and flavonoid contents
2.5.1 TPC analysis
The TPC in WE and EE were quantified using the Folin-Ciocalteu method (Tian et al., 2020). Folin-Ciocalteu reagent (2.5 mL) and sample solution (0.5 mL) were combined and kept at 25°C for 5 min. The 7.5% Na2CO3 solution (4 mL) was subsequently incorporated and reacted for 1 h at 25°C. The absorbance at 760 nm was measured, and gallic acid equivalents (mg GAEs/g extract) were used to express TPC.
2.5.2 TFC analysis
The TFC in WE and EE were quantified using NaNO2-Al(NO3)3-NaOH colorimetry with minor modifications (China Pharmacopoeia Committee, 2020). The sample solution (5 mL) and NaNO2 (0.4 mL, 5%) were blended and kept at 25°C for 5 min, which was reacted with 10% Al(NO3)3 solution (0.4 mL) for 5 min. Subsequently, 4 mL NaOH solution (4%) and distilled water were added to gain a final volume of 10 mL and reacted 15 min at 25°C. The absorbance at 510 nm was measured, and rutin equivalents (mg REs/g extract) were applied to represent TFC.
2.6 Identification of phytochemicals in WE and EE by UHPLC-Q-Orbitrap-MS
UHPLC-Q-Orbitrap-MS (ultra-high-performance liquid chromatography coupled with quadrupole orbitrap mass spectrometer) was employed for the phytochemical analysis of WE and EE. Dionex Ultimate 3000 UHPLC system settings were as follows: Hypersil Gold C18 column (2.1 mm × 100 mm, 1.9 μm), injection volume (5 μL), column temperature (40°C), flow rate (0.3 mL/min), and mobile phases (A: 0.1% formic acid water solution and B: 0.1% formic acid acetonitrile). The gradient elution protocol was as follows: 5% B (0–2 min), 5–95% B (2–42 min), 95% B (42–47 min), 5% B (47.1 min), and 5% B (47.1–50 min).
The MS data collection was performed using Q-Orbitrap-MS (Q Exactive Focus hybrid quadrupole-Orbitrap high-resolution mass spectrometry, Thermo Fisher Scientific) with HESI-II (heated electrospray ionization). The HESI-II settings were as follows: spray voltages –2.5/+ 3.0 kV, sheath gas 35 arb, auxiliary gas 10 arb, sweep gas 0 arb, RF lens amplitude 60, vaporizer temperature 350°C, and capillary temperature 320°C. The mode of full mass/ddMS2 mode was used, and its parameters were as follows: full scan range (m/z 100 to 1,500); resolution MS1 (70,000) and MS2 (17,500); maximum injection time 100 ms (MS1) and 50 ms (MS2); stepped normalized collision energy (20/40/60 eV); automatic gain control (AGC) target values 1e6 (MS1) and 2e5 (MS2). Thermo Fisher Scientific’s Xcalibur 4.1 was used to process the mass spectrum data of the chemical composition. The identification of phytochemical constituents was determined by comparing mzVault and mzCloud databases and references within a 10 ppm threshold.
2.7 Antioxidant properties
The radical scavenging capacities on DPPH (1,1-diphenyl-2-picrylhydrazyl) and ABTS (2,2-azino-bis-3-ethylbenzthiazoline-6-sulphonic acid) were assessed according to the method described in the literature (Tian et al., 2020). Butylated hydroxytoluene (BHT) was used as a positive control.
2.7.1 DPPH assay
Equal amounts of DPPH solution (0.08 mM) and sample solution (100 μL) were mixed, and the reaction was carried out at 25°C for 30 min away from light. Optical density at 517 nm was measured, and IC50 values and BHT equivalents (mg BEs/g sample) were calculated for demonstrating the scavenging effect of DPPH.
2.7.2 ABTS assay
In order to produce the ABTS•+ solution, 50 mL of ABTS solution (0.7 mM) was reacted with an equal amount of K2S2O8 solution (2.45 mM) for 12 h at 25°C without light. In addition, it was further diluted with ethanol prior to use to yield an absorbance of 0.70 ± 0.02 at 734 nm. Then, 0.4 mL of sample solution reacted with 4 mL of diluted ABTS•+ solution for 10 min at 25°C away from light. Optical density at 734 nm was gained, and IC50 values and the equivalents of BHT (mg BEs/g sample) were expressed in the results.
2.8 Antimicrobial properties
2.8.1 Bacterial strains
The antibacterial properties of EO, WE, and EE was assessed against Gram-negative bacterias (Escherichia coli CICC 10389, Enterococcus faecalis ATCC 19433, and Proteus vulgaris ACCC 11002) and Gram-positive bacterias (Pseudomonas aeruginosa ATCC 9027, Bacillus subtilis ATCC 6633, and Staphylococcus aureus ATCC 6538P).
2.8.2 Agar well diffusion assay
The agar well diffusion method assayed the diameter of the inhibition zone (DIZ) (Zhang et al., 2017). EO was dissolved in DMSO and diluted with sterile distilled water (DMSO content was less than 0.5%). Sterile distilled water was used to dissolve the WE and EE. Three sample solutions were brought to a concentration of 100 mg/mL. Streptomycin was dissolved in distilled water to reach a 100 μg/mL concentration, which was used as a positive control. The Mueller-Hinton agar medium surface was spread with 100 μL of the bacterial suspension (1 × 107 CFU/mL) and was covered with filter paper discs of 6 mm diameter (containing 20 μL sample solutions). The DIZ was determined after 24 h of incubation at 37°C.
2.8.3 Determination of MIC and MBC
In a 96-well plate, 100 μL of bacterial suspensions (1 × 106 CFU/mL) was added to an equal amount of sample solution. After 24 h of incubation at 37°C, each well was supplied with 20 μL of resazurin aqueous solution (100 μg/mL), which was cultured for 2 h at 37°C without light. The minimal sample concentration without color change was determined as MIC (minimal inhibitory concentration) (Tian et al., 2020). The mixture (10 μL) from the wells that did not change color was subcultured in a Mueller Hinton agar plate for 24 h at 37°C, the least sample concentration at which no bacterial growth was determined as MBC (minimal bactericidal concentration) (Eloff, 1998).
2.8.4 Antifungal capacity
The anti-Aspergillus flavus activity of EO was tested by a previously published method with slight modification (da Silva et al., 2012) using amphotericin B as a positive control. The EO solution (3.125 mg/mL–100 mg/mL) was mixed with Potato Dextrose Agar (PDA) medium (2:18, v:v). Subsequently, the mixture (15 mL) was added to Petri dishes (diameter 90 mm) and solidified. The A. flavus spore suspension (1 × 103 CFU/mL, 100 μL) was inoculated into wells (6 mm in diameter) of the culture medium and incubated at 28°C for 36 h. The MIC value was recorded as EO concentrations that inhibited the visible growth of A. flavus, and the diameter of colony growth zone was less than 12 mm (including well diameter of 6 mm) (Yadav et al., 2005). The minimum fungicidal concentration (MFC) was the minimum sample concentration showing no A. flavus growth on the PDA medium.
2.9 Enzyme inhibitory properties
The enzyme inhibitory properties of A. coriandriodora rhizome WE, EE, and EO on cholinesterases (AChE and BChE), tyrosinase, and α-glucosidase were tested, and galanthamine, arbutin, and acarbose were used as positive controls, respectively (Tian et al., 2020).
2.9.1 Cholinesterases inhibition
In a 96-well plate, 10 μL of AChE or BuChE solution (pH 8.0, 0.5 U/mL) and 50 μL of sample solution were mixed and incubated for 5 min at 4°C. Subsequently, each well was injected with 20 μL of ATCI (acetylthiocholine iodide) or BTCl (butyrylthiocholine chloride) solution (2 mM) and 20 μL of color-developing agent 5,5’-dithiobis-(2-nitrobenzoic acid) solution (2 mM). After 30 min of reaction at 37°C, the absorbance at 405 nm was read. The galanthamine equivalents (mg GALAEs/g sample) and IC50 values expressed cholinesterase inhibition.
2.9.2 Tyrosinase inhibition
In a 96-well plate, 100 μL of tyrosinase solution (100 U/mL) was mixed with 70 μL of sample solution and incubated for 5 min at 37°C. Then, 80 μL of L-tyrosine solution (5.5 mM) was injected into each well. After 30 min of reaction at 37°C, the absorbance at 492 nm was measured. Data were presented as arbutin equivalents (mg AREs/g sample) and IC50 values.
2.9.3 α-Glucosidase inhibition
In a 96-well plate, 10 μL of α-glucosidase solution was reacted with 90 μL sample solution for 15 min at 37°C. Next, 10 μL of p-NPG (p-Nitrophenyl-α-D-glucopyranoside) solution (1 mM) was injected into each well and maintained at 37°C or 15 min. Lastly, 80 μL of Na2CO3 (0.2 M) was added for stopping the reaction. After the absorbance detection at 405 nm, α-glucosidase inhibition was represented as acarbose equivalents (mmoL ACEs/g sample) and IC50 values.
2.10 Statistical analysis
All data were collected from three independently performed experiments and were expressed as mean ± standard deviation (SD). In SPSS (version 25) software, the two-tailed unpaired t-test or one-way analysis of variance (ANOVA) and Fischer’s LSD post hoc test (p < 0.05) were utilized to compare the significant difference between the two groups.
3 Results and discussion
3.1 Chemical composition of EO
Based on the fresh weight of A. coriandriodora rhizome, the extraction rate of EO was 0.56% (w/w). As shown in Table 1, a total of 27 chemical compositions, representing 97.0% of the total EO, were identified by GC-MS/FID. As shown in Figure 1, it was mainly composed of (E)-2-decenal (53.8%), (E)-2-decenyl acetate (24.4%), (Z)-3-dodecenyl acetate (3.5%), and (E)-2-octenal (3.5%). According to our previous study, EO’s main compounds from A. coriandriodora rhizome harvested at the same location (Guangxi, China) in July 2019 were (E)-2-decenal (56.3%), (E)-2-decenyl acetate (19.6%), (Z)-3-dodecenyl acetate (3.8%), and (E)-2-octenal (3.6%) (Hong et al., 2022). The main chemical components of A.coriandriodora essential oils from July and August are the same, and the difference in their content may be caused by different harvesting times.
3.2 Chemical composition of WE and EE
Based on the fresh weight of A. coriandriodora rhizome, the yield of WE and EE were 2.04% (w/w) and 1.37% (w/w), respectively. As shown in Figure 2, A. coriandriodora rhizome WE (60.49 ± 0.24 mg GAEs/g extract) exhibited more TPC compared to EE (52.99 ± 0.16 mg GAEs/g extract) (p < 0.01). The TFC in WE (286.42 ± 2.21 mg REs/g extract) was notably greater than that in EE (260.69 ± 0.44 mg REs/g extract) (p < 0.01).
The phytochemical components of WE and EE were further analyzed using UHPLC-Q-Orbitrap-MS. Sixty-three chemicals were identified based on comparisons of primary and secondary mass spectrometry with the mzVault and mzCloud databases and references (Table 2). As illustrated in Figure S1 (Supplementary Material), WE and EE displayed variations in phytochemical constituents. Thirty-seven and fifty-three components were identified from WE and EE, respectively. WE and EE had high TPC and TFC values, and fourteen identified phenolic compounds and twenty-three flavonoid compounds were identified. The fourteen identified phenolic compounds included hordenine (5), protocatechuic acid (8), 3,4-dihydroxyphenylethanol (9), methyl gallate (10), vanillic acid (11), protocatechualdehyde (12), 4-methoxysalicylic acid (13), orsellinic acid (15), 2-hydroxy-4-methoxybenzaldehyde (19), p-coumaric acid (21), ethyl 3,4-dihydroxybenzoate (33), ferulaldehyde (34), tetrahydrocurcumin (36), and arctigenin (39). The twenty-three identified flavonoid compounds consisted of epicatechin (14), procyanidin B1 (16), astilbin (17), cianidanol (18), (+)-catechin hydrate (20), procyanidin A2 (23), hyperoside (24), quercetin (26), isoquercitrin (27), quercetin 3-O-β-D-Glucuronide (29), tetrahydroxyxanthone (31), morin (35), pectolinarigenin (40), lysionotin (41), isorhamnetin (43), isoxanthohumol (44), gardenin B (46), hydroxygenkwanin (47), 6-demethoxytangeretin (48), jaceosidin (49), eupatilin (50), 4’,7-di-O-methylnaringenin (53), and acacetin (57). All sixty-three constituents were first identified from A. coriandriodora. These findings suggested that A. coriandriodora rhizome can serve as a rich source of flavonoids and phenolic compounds.
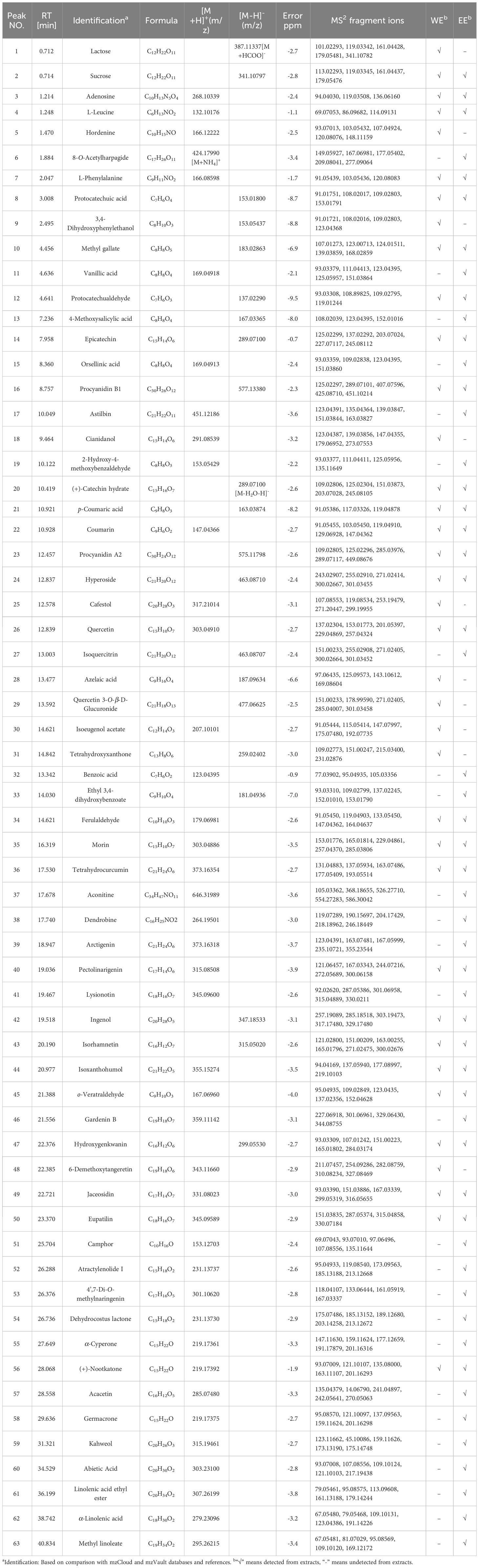
Table 2 Phytochemical compounds of A. coriandriodora rhizome WE and EE detected and characterized using UHPLC-Q-Orbitrap-MS in positive and negative ionization modes.
3.3 Antioxidant capacity
In the antioxidant capacity of A. coriandriodora rhizome (Table 3), the scavenging effect of EO on DPPH and ABTS was weak. WE (IC50: 6.59 ± 0.88 μg/mL) and EE (IC50: 17.70 ± 1.15 μg/mL) possessed potent DPPH scavenging ability, which was superior to that of BHT (IC50: 21.83 ± 0.89 μg/mL) (p < 0.05). In the ABTS analysis, WE and EE exhibited significant ABTS radical scavenging activity, with IC50 values of 20.48 ± 0.44 μg/mL and 18.27 ± 0.55 μg/mL, respectively. Excessive production of reactive oxygen species triggers oxidative stress, which could induce or aggravate many chronic diseases, such as neurodegenerative disorders, cardiovascular disease, asthma, and cancer (López-Alarcón and Denicola, 2013; Pisoschi and Pop, 2015). Past studies have revealed that various phenolic and flavonoid compounds from Alpinia genus plants were natural antioxidants that are effective in scavenging free radicals (Honmore et al., 2016; Tang et al., 2018; da Cruz et al., 2020). Based on the results of UHPLC-Q-Orbitrap-MS, a variety of identified phenolic and flavonoid compounds, such as protocatechuic acid (8), methyl gallate (10), vanillic acid (11), and epicatechin (14), has been proved to have DPPH and ABTS scavenging effects (Kakkar and Bais, 2014; Li et al., 2014; Bernal-Mercado et al., 2018; Ryu and Kim, 2022). Hence, the antioxidant activity of A. coriandriodora rhizome WE and EE can be attributed to their richness in phenolics and flavonoids, which can serve as a natural source of antioxidants.
3.4 Antibacterial properties
The antibacterial properties of EO, WE, and EE were determined qualitatively using the DIZ and assessed quantitatively using the MIC and MBC. Streptomycin was used as the control drug (Table 4). The DIZ of WE and EE (100 mg/mL) were not observed, and the MIC and MBC of WE and EE were not listed as they were not detected at 25 mg/mL. EO exhibited broad-spectrum antibacterial activity with DIZ values ranging from 12.60 to 22.17 mm. Previous studies suggest that MIC values less than 5 mg/mL have potent antibacterial properties (Monteiro et al., 2021). Hence, the EO displayed a strong antibacterial effect against P. vulgaris (MIC = 1.56 mg/mL, MBC = 3.13 mg/mL), E. faecalis (MIC = 0.78 mg/mL, MBC = 3.13 mg/mL), B. subtilis (MIC = 0.78 mg/mL, MBC = 3.13 mg/mL), E. coli (MIC = 0.78 mg/mL, MBC = 6.25 mg/mL) and S. aureus (MIC = 0.78 mg/mL, MBC = 3.13 mg/mL). In addition, it revealed moderate antibacterial activity against P. aeruginosa (MIC = 6.25 mg/mL, MBC = 6.25 mg/mL). (E)-2-Decenal, as the predominant component, has been proven to possess broad-spectrum antibacterial action, with MIC values ranging from 7.8 to 500 μg/mL against E. coli, S. aureus, S. epidermidis, Salmonella typhi, S. enteritidis, Bacillus cereus, Moraxella catarrhalis, Haemophilus influenzae, Listeria monocytogenes, Streptococcus pneumoniae, and S. pyogenes (Bisignano et al., 2001; Trombetta et al., 2002). (E)-2-Octenal was a potential antibacterial agent that inhibited the growth of a variety of bacteria, including S. aureus, E. coli, P. vulgaris, P. aeruginosa, Enterobacter aerogenes, Propionibacterium acnes, Streptococcus mutans, Brevibacterium ammoniagenes, and Bacillus subtilis (Kubo and Kubo, 1995; Sagun et al., 2016). Thus, the antibacterial action of A. coriandriodora EO can be attributed to these main components. Bacterial infections and the emergence of bacterial resistance have threatened human public health around the world (Fernandes et al., 2022). Therefore, EO with antibacterial properties has gained great attention. Our results suggested that A. coriandriodora EO may be used in the food and pharmaceutical industries as a natural antibacterial agent.
3.5 Antifungal capacity
The anti-Aspergillus flavus properties of A. coriandriodora rhizome EO are shown in Figure 3 and Table 5. The EO exhibited anti-Aspergillus flavus effects in a dose-dependent manner. The growth of A. flavus on the PDA medium was obviously inhibited when the EO concentration reached 0.625 mg/mL (MIC). Besides, EO completely inhibited A. flavus growth at the concentration of 1.250 mg/mL (MFC). (E)-2-Decenal is a potent antifungal agent against several fungi, including Trichophyton mentagrophytes, Penicillium chrysogenum, Pityrosprum ovale, Candida utilis, Saccharomyces cerevisiae, Purpureocillium lilacinum, Isaria fumosorosea, Metarhizium flavoviride, Paecilomyces suffultus, Beauveria bassiana, and Akanthomyces lecanii (Kubo and Kubo, 1995; Bojke et al., 2020). (E)-2-Octenal has been proven to be a substitute for chemical fungicides with antifungal activity against Trichophyton mentagrophytes, Penicillium italicum, Sclerotium rolfsii, Aspergillus niger, Penicillium digitatum, Alternaria alternate, Microsporum canis, Botrytis cinerea, etc. (Battinelli et al., 2006; Liarzi et al., 2016; Liarzi et al., 2020). In particular, (E)-2-octenal exerted a potent inhibitory effect on the growth of A. flavus and the production of aflatoxin B1 (Cleveland et al., 2009). Hence, these compounds could explain the remarkable anti-Aspergillus flavus activity of A. coriandriodora rhizome EO. A. flavus is known to cause spoilage of vegetables, grains, fruits, and nuts, which results in huge economic losses worldwide (Hashem et al., 2022). In addition, aflatoxin produced by A. flavus poses a great threat to human health due to its immunosuppressive, genotoxic, and carcinogenic effects (Lasram et al., 2019; Yadav et al., 2019). Recent studies have suggested that essential oils can be used as a greener alternative to protect foods from Aspergillus flavus contamination (Dutta et al., 2020; Kundu et al., 2022). Hence, A. coriandriodora rhizome EO has the exploitation potential as an anti-Aspergillus flavus agent in the food field.
3.6 Enzyme inhibitory properties
The enzyme inhibitory properties of A. coriandriodora rhizome WE, EE, and EO on cholinesterases (AChE and BChE), tyrosinase, and α-glucosidase were tested, and the results are shown in Table 6.
AD (Alzheimer’s disease) is a chronic disease with memory loss and cognitive impairment caused by central nervous system degeneration, and low levels of cholinergic energy in the human brain play an essential role in the pathogenesis of AD (Sharma, 2019). As shown in Table 6, compared with the positive control galanthamine, EO, WE, and EE of A. coriandriodora rhizome showed weak AChE (IC50: 5.84 ± 0.48 mg/mL, 6.67 ± 0.04 mg/mL, and 1.59 ± 0.09 mg/mL, respectively) and BChE (IC50: 5.73 ± 0.33 mg/mL, 3.61 ± 0.89 mg/mL, and 0.38 ± 0.02 mg/mL, respectively) inhibitory activity.
In mammals, tyrosinase is a crucial enzyme in the production of melanin, which protects against UV damage, but excessive melanin can lead to many skin problems, such as malignant melanoma, freckles, and age spots (Brenner and Hearing, 2008; Sun et al., 2017). Tyrosinase inhibitors have been extensively applied in cosmetic and medicine industries to solve these problems. According to the results presented in Table 6, EO (IC50: 1.01 ± 0.08 mg/mL, 253.12 ± 9.13 mg AREs/g sample) had the most strong tyrosinase inhibition, followed by WE (IC50: 8.91 ± 1.69 mg/mL, 29.78 ± 5.61 mg AREs/g sample) and EE (IC50: 19.85 ± 3.74 mg/mL, 13.21 ± 2.71 mg AREs/g sample). (E)-2-octenal, as the main components of A. coriandriodora rhizome EO, have been demonstrated to possess tyrosinase inhibition (Kubo and Kinst-Hori, 1999). Plant-derived flavonoids and phenolic compounds can serve as natural inhibitors of tyrosinase (Goncçalves and Romano, 2017). A variety of phenolic and flavonoid compounds identified from WE and EE, such as orsellinic acid, arctigenin, and hordenine, have been confirmed to possess tyrosinase inhibitory activity (Kim et al., 2013; Park et al., 2013; Lopes et al., 2018). Thus, the tyrosinase inhibition of A. coriandriodora rhizome can be attributed to the presence of these active ingredients.
The α-glucosidase inhibitors can decrease the intake of postprandial carbohydrates by reducing the digestion of carbohydrates and are an effective means of treating type II diabetes (Gao et al., 2022). Nevertheless, synthetic α-glucosidase inhibitors, such as voglibose, emiglitate, miglitol, and acarbose, have unacceptable consequences, including flatulence, nausea, vomiting, diarrhea, stomach pain, and allergic reactions (Liu et al., 2015; Hossain et al., 2020). Hence, there is an urgent need to find more effective and safer natural products as α-glucosidase inhibitors. As illustrated in Table 6, the inhibitory effects of A. coriandriodora rhizome on α-glucosidase were as follows: EE (IC50: 0.013 ± 0.001 mg/mL) > WE (IC50: 0.017 ± 0.002 mg/mL) > acarbose (IC50: 0.22 ± 0.01 mg/mL) > EO (IC50: 3.30 ± 0.17 mg/mL) (p < 0.05). Notably, the α-glucosidase inhibitory capacity of WE (19.92 ± 1.19 mmoL ACEs/g sample) and EE (25.87 ± 2.32 mmoL ACEs/g sample) was significantly higher than acarbose (p < 0.05).
Previous studies have indicated that phenolic compounds and flavonoids can act as potent α-glucosidase inhibitors (Goncçalves and Romano, 2017). Various phenolic and flavonoid compounds identified from A. coriandriodora rhizome, such as hordenine (Su et al., 2018), p-coumaric acid (Amalan et al., 2016), procyanidin A2 (Sheikh et al., 2019), eupatilin (Kang et al., 2008), and acacetin (Wang et al., 2023) were obviously lowered fasting blood glucose level of diabetic mice. In addition, protocatechualdehyde (Ji et al., 2021; Luo et al., 2021), hyperoside (Ku et al., 2014; Luo et al., 2021), quercetin (Vessal et al., 2003; Nurul Islam et al., 2013), and isoquercitrin (Zhang et al., 2018; Luo et al., 2021) displayed significant anti-diabetic properties in vivo and α-glucosidase inhibitory properties in vitro that were stronger than the positive control acarbose. Furthermore, epicatechin (Lin et al., 2022) and pectolinarigenin (Amin et al., 2017) showed stronger inhibitory efficacy against α-glucosidase compared to acarbose. These phenolic and flavonoid compounds may contribute to the potent α-glucosidase inhibition of A. coriandriodora rhizome WE and EE. Hence, A. coriandriodora rhizome WE and EE could be utilized as a novel source of α-glucosidase inhibitors for the functional food and pharmaceutical industries.
4 Conclusions
In addition to the chemical composition of A. coriandriodora rhizome EO, this study is the first to analyze the phytochemical components of WE and EE and evaluate their antioxidant, antimicrobial, and enzyme inhibitory properties. According to the GC-MS data, the primary components of A. coriandriodora rhizome EO were (E)-2-decenal, (E)-2-decenyl acetate, (Z)-3-dodecenyl acetate, and (E)-2-octenal. A. coriandriodora rhizome WE and EE were abundant in phenolics and flavonoids. UHPLC-Q-Orbitrap-MS analysis further identified sixty-three compounds in WE and EE, including 14 phenolic compounds and 23 flavonoids. WE and EE exhibited a potent DPPH radical scavenging effect, superior to the positive control BHT. Besides, EO displayed powerful antimicrobial activity against E. faecalis, S. aureus, B. subtilis, E. coli, P. vulgaris, and A. flavus. In enzyme inhibitory activity, the EE and WE revealed a remarkable α-glucosidase inhibition, which were higher than positive control acarbose. Hence, A. coriandriodora rhizome may be used as a source of bioactive substances and has excellent potential for exploitation in the pharmaceutical and food fields.
Data availability statement
The datasets presented in this study can be found in online repositories. The names of the repository/repositories and accession number(s) can be found below: MetaboLights, MTBLS8631.
Author contributions
XW: Investigation, Methodology, Writing – original draft. FW: Investigation, Validation, Writing – original draft. FD: Investigation, Validation, Writing – original draft. NY: Investigation, Validation, Writing – original draft. JN: Investigation, Writing – original draft. YR: Formal Analysis, Writing – original draft. MT: Conceptualization, Funding acquisition, Methodology, Supervision, Validation, Writing – original draft, Writing – review & editing.
Funding
The author(s) declare financial support was received for the research, authorship, and/or publication of this article. This work was supported by the National Natural Science Foundation of China (82360834 and 32260747) and the Science and Technology Program of Guizhou province, China [Qian Ke He Ji Chu-ZK (2021) Yiban 150, Qian Ke He Zhi Cheng (2020) 1Y133].
Conflict of interest
The authors declare that the research was conducted in the absence of any commercial or financial relationships that could be construed as a potential conflict of interest.
Publisher’s note
All claims expressed in this article are solely those of the authors and do not necessarily represent those of their affiliated organizations, or those of the publisher, the editors and the reviewers. Any product that may be evaluated in this article, or claim that may be made by its manufacturer, is not guaranteed or endorsed by the publisher.
Supplementary material
The Supplementary Material for this article can be found online at: https://www.frontiersin.org/articles/10.3389/fpls.2023.1284931/full#supplementary-material
References
Amalan, V., Vijayakumar, N., Indumathi, D., Ramakrishnan, A. (2016). Antidiabetic and antihyperlipidemic activity of p-coumaric acid in diabetic rats, role of pancreatic GLUT 2: In vivo approach. Biomed. Pharmacother. 84, 230–236. doi: 10.1016/j.biopha.2016.09.039
Amin, A., Tuenter, E., Foubert, K., Iqbal, J., Cos, P., Maes, L., et al. (2017). In Vitro and In Silico Antidiabetic and Antimicrobial Evaluation of Constituents from Kickxia ramosissima (Nanorrhinum ramosissimum). Front. Pharmacol. 8. doi: 10.3389/fphar.2017.00232
Battinelli, L., Daniele, C., Cristiani, M., Bisignano, G., Saija, A., Mazzanti, G. (2006). In vitro antifungal and anti-elastase activity of some aliphatic aldehydes from Olea europaea L. fruit. Phytomedicine 13, 558–563. doi: 10.1016/j.phymed.2005.09.009
Bernal-Mercado, A. T., Vazquez-Armenta, F. J., Tapia-Rodriguez, M. R., Islas-Osuna, M. A., Mata-Haro, V., Gonzalez-Aguilar, G. A., et al. (2018). Comparison of Single and Combined Use of Catechin, Protocatechuic, and Vanillic Acids as Antioxidant and Antibacterial Agents against Uropathogenic Escherichia Coli at Planktonic and Biofilm Levels. Molecules 23, 2813. doi: 10.3390/molecules23112813
Bisignano, G., Laganà, M. G., Trombetta, D., Arena, S., Nostro, A., Uccella, N., et al. (2001). In vitro antibacterial activity of some aliphatic aldehydes from Olea europaea L. FEMS Microbiol. Lett. 198, 9–13. doi: 10.1111/j.1574-6968.2001.tb10611.x
Bojke, A., Tkaczuk, C., Bauer, M., Kamysz, W., Gołębiowski, M. (2020). Application of HS-SPME-GC-MS for the analysis of aldehydes produced by different insect species and their antifungal activity. J. Microbiol. Methods 169, 105835. doi: 10.1016/j.mimet.2020.105835
Brenner, M., Hearing, V. J. (2008). The protective role of melanin against UV damage in human skin. Photochem. Photobiol. 84, 539–549. doi: 10.1111/j.1751-1097.2007.00226.x
Cheng, X. L., Li, H. X., Chen, J., Wu, P., Xue, J. H., Zhou, Z. Y., et al. (2021). Bioactive diarylheptanoids from Alpinia coriandriodora. Nat. Prod. Bioprospect. 11, 63–72. doi: 10.1007/s13659-020-00264-y
China Pharmacopoeia Committee (2020). Pharmacopoeia of the People's Republic of China (Beijing: China Medical Science and Technology Press), 414–415.
CHMC-Chinese Herbal Medicine Company (1994). The Chinese Traditional Medicine Resource Records (Beijing: Science Press), 1502.
Cleveland, T. E., Carter-Wientjes, C. H., De Lucca, A. J., Boué, S. M. (2009). Effect of soybean volatile compounds on Aspergillus flavus growth and aflatoxin production. J. Food. Sci. 74, H83–H87. doi: 10.1111/j.1750-3841.2009.01078.x
da Cruz, J. D. D., Mpalantinos, M. A., Ramos, A. D. S., Ferreira, J. L. P., de Oliveira, A. A., Júnior, N. L. N., et al. (2020). Chemical standardization, antioxidant activity and phenolic contents of cultivated Alpinia zerumbet preparations. Ind. Crop Prod. 151, 112495. doi: 10.1016/j.indcrop.2020.112495
da Silva, F., Chalfoun, S. M., de Siqueira, V. M., Botelho, D. M. D. S., Lima, N., Batista, L. R. (2012). Evaluation of antifungal activity of essential oils against potentially mycotoxigenic Aspergillus flavus and Aspergillus parasiticus. Rev. Bras. Farmacogn. 22, 1002–1010. doi: 10.1590/S0102-695X2012005000052
Dutta, S., Kundu, A., Saha, S., Prabhakaran, P., Mandal, A. (2020). Characterization, antifungal properties and in silico modelling perspectives of Trachyspermum ammi essential oil. Lwt 131, 109786. doi: 10.1016/j.lwt.2020.109786
Eloff, J. N. (1998). A sensitive and quick microplate method to determine the minimal inhibitory concentration of plant extracts for bacteria. Planta Med. 64, 711–713. doi: 10.1055/s-2006-957563
Fernandes, P. A., Pereira, R. L., Santos, A. T., Coutinho, H. D., Morais-Braga, M. F., Da Silva, V. B., et al. (2022). Phytochemical analysis, antibacterial activity and modulating effect of essential oil from Syzygium cumini (L.) skeels. Molecules 27, 3281. doi: 10.3390/molecules27103281
Gao, Y., Bian, W., Fang, Y., Du, P., Liu, X., Zhao, X., et al. (2022). α-glucosidase inhibitory activity of fermented okara broth started with the strain Bacillus amyloliquefaciens SY07. Molecules 27, 1127. doi: 10.3390/molecules27031127
Ghosh, S., Rangan, L. (2013). Alpinia: the gold mine of future therapeutics. 3 Biotech. 3, 173–185. doi: 10.1007/s13205-012-0089-x
Goncçalves, S., Romano, A. (2017). “Inhibitory properties of phenolic compounds against enzymes linked with human diseases,” in Phenolic Compounds-Biological Activity. Eds. Soto-Hernández, M., Palma-Tenango, M., Garcia-Mateos, R. (London: InTech), 99–118.
Hashem, A. H., Saied, E., Amin, B. H., Alotibi, F. O., Al-Askar, A. A., Arishi, A. A., et al. (2022). Antifungal activity of biosynthesized silver nanoparticles (AgNPs) against Aspergilli causing aspergillosis: ultrastructure study. J. Funct. Biomater. 13, 242. doi: 10.3390/jfb13040242
Hong, Y., Liu, X., Wang, H., Zhang, M., Tian, M. (2022). Chemical composition, anticancer activities and related mechanisms of the essential oil from Alpinia coriandriodora rhizome. Ind. Crop Prod. 176, 114328. doi: 10.1016/j.indcrop.2021.114328
Honmore, V. S., Kandhare, A. D., Kadam, P. P., Khedkar, V. M., Sarkar, D., Bodhankar, S. L., et al. (2016). Isolates of Alpinia officinarum hance as COX-2 inhibitors: evidence from anti-inflammatory, antioxidant and molecular docking studies. Int. Immunopharmacol. 33, 8–17. doi: 10.1016/j.intimp.2016.01.024
Hossain, U., Das, A. K., Ghosh, S., Sil, P. C. (2020). An overview on the role of bioactive α-glucosidase inhibitors in ameliorating diabetic complications. Food Chem. Toxicol. 145, 111738. doi: 10.1016/j.fct.2020.111738
Ibrahim, H., Sivasothy, Y., Syamsir, D. R., Nagoor, N. H., Jamil, N., Awang, K. (2014). Essential Oil Composition and Antimicrobial Activities of Two Closely Related Species, Alpinia mutica Roxb. and Alpinia latilabris Ridl., from Peninsular Malaysia. Sci. World J. 2014, 430831. doi: 10.1155/2014/430831
Ji, B., Yuan, K., Li, J., Ku, B. J., Leung, P. S., He, W. (2021). Protocatechualdehyde restores endothelial dysfunction in streptozotocin-induced diabetic rats. Ann. Transl. Med. 9, 711. doi: 10.21037/atm-21-1431
Kakkar, S., Bais, S. (2014). A review on protocatechuic acid and its pharmacological potential. ISRN Pharmacol. 2014, 952943. doi: 10.1155/2014/952943
Kang, Y. J., Jung, U. J., Lee, M. K., Kim, H. J., Jeon, S. M., Park, Y. B., et al. (2008). Eupatilin, isolated from Artemisia princeps Pampanini, enhances hepatic glucose metabolism and pancreatic β-cell function in type 2 diabetic mice. Diabetes Res. Clin. Pract. 82, 25–32. doi: 10.1016/j.diabres.2008.06.012
Kim, S., Lee, J., Kim, M., Lee, J., Kim, Y. B., Jung, E., et al. (2013). Hordenine, a single compound produced during barley germination, inhibits melanogenesis in human melanocytes. Food Chem. 141, 174–181. doi: 10.1016/j.foodchem.2013.03.017
Ku, S. K., Kwak, S., Kwon, O. J., Bae, J. S. (2014). Hyperoside inhibits high-glucose-induced vascular inflammation in vitro and in vivo. Inflammation 7, 1389–1400. doi: 10.1007/s10753-014-9863-8
Kubo, I., Kinst-Hori, I. (1999). Tyrosinase inhibitory activity of the olive oil flavor compounds. J. Agric. Food Chem. 47, 4574–4578. doi: 10.1021/jf990165v
Kubo, A., Kubo, I. (1995). Antimicrobial agents from Tanacetum balsamita. J. Nat. Prod. 58, 1565–1569. doi: 10.1021/np50124a013
Kundu, A., Mandal, A., Dutta, A., Saha, S., Raina, A. P., Kumar, R., et al. (2022). Nanoemulsification of Kaempferia galanga essential oil: Characterizations and molecular interactions explaining fungal growth suppression. Process Biochem. 121, 228–239. doi: 10.1016/j.procbio.2022.07.008
Lasram, S., Zemni, H., Hamdi, Z., Chenenaoui, S., Houissa, H., Saidani Tounsi, M. S., et al. (2019). Antifungal and antiaflatoxinogenic activities of Carum carvi L., Coriandrum sativum L. seed essential oils and their major terpene component against Aspergillus flavus. Ind. Crop Prod. 134, 11–18. doi: 10.1016/j.indcrop.2019.03.037
Li, T., Shen, P., Liu, W., Liu, C., Liang, R., Yan, N., et al. (2014). Major polyphenolics in pineapple peels and their antioxidant interactions. Int. J. Food Prop. 17, 1805–1817. doi: 10.1080/10942912.2012.732168
Liarzi, O., Bar, E., Lewinsohn, E., Ezra, D. (2016). Use of the endophytic fungus Daldinia cf. concentrica and its volatiles as Bio-control agents. PloS One 11, e0168242. doi: 10.1371/journal.pone.0168242
Liarzi, O., Benichis, M., Gamliel, A., Ezra, D. (2020). trans-2-Octenal, a single compound of a fungal origin, controls Sclerotium rolfsii, both in vitro and in soil. Pest Manage. Sci. 76, 2068–2071. doi: 10.1002/ps.5744
Lin, Y. T., Lin, H. R., Yang, C. S., Liaw, C. C., Sung, P. J., Kuo, Y. H., et al. (2022). Antioxidant and Anti-α-Glucosidase activities of Various solvent extracts and major bioactive components from the fruits of. Crataegus pinnatifida. Antioxidants 11, 320. doi: 10.3390/antiox11020320
Liu, C., Xiang, W., Yu, Y., Shi, Z., Huang, X., Xu, L. (2015). Comparative analysis of 1-deoxynojirimycin contribution degree to α-glucosidase inhibitory activity and physiological distribution in Morus alba L. Ind. Crop Prod. 70, 309–315. doi: 10.1016/j.indcrop.2015.02.046
Lopes, T. I. B., Coelho, R. G., Honda, N. K. (2018). Inhibition of mushroom tyrosinase activity by orsellinates. Chem. Pharm. Bull. 66, 61–64. doi: 10.1248/cpb.c17-00502
López-Alarcón, C., Denicola, A. (2013). Evaluating the antioxidant capacity of natural products: A review on chemical and cellular-based assays. Anal. Chim. Acta 763, 1–10. doi: 10.1016/j.aca.2012.11.051
Luo, D., Mu, T., Sun, H. (2021). Profiling of phenolic acids and flavonoids in sweet potato (Ipomoea batatas L.) leaves and evaluation of their anti-oxidant and hypoglycemic activities. Food Biosci. 39, 100801. doi: 10.1016/j.fbio.2020.100801
Monteiro, P. C., Majolo, C., Chaves, F. C. M., Bizzo, H. R., Almeida O’Sullivan, F. L., Chagas, E. C. (2021). Antimicrobial activity of essential oils from Lippia sidoides, Ocimum gratissimum and Zingiber officinale against Aeromonas spp. J. Essent. Oil Res. 33, 152–161. doi: 10.1080/10412905.2020.1848653
Nurul Islam, M., Jung, H. A., Sohn, H. S., Kim, H. M., Choi, J. S. (2013). Potent α-glucosidase and protein tyrosine phosphatase 1B inhibitors from Artemisia capillaris. Arch. Pharm. Res. 36, 542–552. doi: 10.1007/s12272-013-0069-7
Park, H., Song, K. H., Jung, P. M., Kim, J., Ro, H., Kim, M. Y., et al. (2013). Inhibitory effect of arctigenin from fructus arctii extract on melanin synthesis via repression of tyrosinase expression. Evid. Based Complement. Altern. Med. 2013, 965312. doi: 10.1155/2013/965312
Pisoschi, A. M., Pop, A. (2015). The role of antioxidants in the chemistry of oxidative stress: A review. Eur. J. Med. Chem. 97, 55–74. doi: 10.1016/j.ejmech.2015.04.040
Rajasekar, R., Manokaran, K., Rajasekaran, N., Duraisamy, G., Kanakasabapathi, D. (2014). ). Effect of Alpinia calcarata on glucose uptake in diabetic rats-an in vitro and in vivo model. J. Diabetes Metab. Disord. 13, 33. doi: 10.1186/2251-6581-13-33
Ryu, B. I., Kim, K. T. (2022). Antioxidant activity and protective effect of methyl gallate against t-BHP induced oxidative stress through inhibiting ROS production. Food Sci. Biotechnol. 31, 1063–1072. doi: 10.1007/s10068-022-01120-0
Sagun, S., Collins, E., Martin, C., Nolan, E. J., Horzempa, J. (2016). Alarm odor compounds of the brown marmorated stink bu g exhibit antibacterial activity. J. Pharmacogn. Nat. Prod. 2, 119. doi: 10.4172/2472-0992.1000119
Sharma, K. (2019). Cholinesterase inhibitors as Alzheimer's therapeutics (Review). Mol. Med. Rep. 20, 1479–1487. doi: 10.3892/mmr.2019.10374
Sheikh, Y., Chanu, M. B., Mondal, G., Manna, P., Chattoraj, A., Chandra Deka, D., et al. (2019). Procyanidin A2, an anti-diabetic condensed tannin extracted from Wendlandia glabrata, reduces elevated G-6-Pase and mRNA levels in diabetic mice and increases glucose uptake in CC1 hepatocytes and C1C12 myoblast cells. Rsc Adv. 9, 17211. doi: 10.1039/C9RA02397F
Shi, S., Zhao, X., Liu, A., Liu, B., Li, H., Wu, B., et al. (2015). Protective effect of n-butanol extract from Alpinia oxyphylla on learning and memory impairments. Physiol. Behav. 139, 13–20. doi: 10.1016/j.physbeh.2014.11.016
Su, S., Cao, M., Wu, G., Long, Z., Cheng, X., Fan, J., et al. (2018). Hordenine protects against hyperglycemia-associated renal complications in streptozotocin-induced diabetic mice. Biomed. Pharmacother. 104, 315–324. doi: 10.1016/j.biopha.2018.05.036
Sun, L., Guo, Y., Zhang, Y., Zhuang, Y. (2017). Antioxidant and anti-tyrosinase activities of phenolic extracts from rape bee pollen and inhibitory melanogenesis by cAMP/MITF/TYR pathway in B16 mouse melanoma cells. Front. Pharmacol. 8. doi: 10.3389/fphar.2017.00104
Tang, X., Xu, C., Yagiz, Y., Simonne, A., Marshall, M. R. (2018). Phytochemical profiles, and antimicrobial and antioxidant activities of greater galangal [Alpinia galanga (Linn.) Swartz.] flowers. Food Chem. 255, 300–308. doi: 10.1016/j.foodchem.2018.02.027
Tian, M., Wu, X., Lu, T., Zhao, X., Wei, F., Deng, G., et al. (2020). Phytochemical analysis, antioxidant, antibacterial, cytotoxic, and enzyme inhibitory activities of Hedychium flavum rhizome. Front. Pharmacol. 11. doi: 10.3389/fphar.2020.572659
Tran, B. T., Vu, X. T., Dao, N. A., Do, T. K. T., Nguyen, T. T. M., Hoang, Q. C., et al. (2022). Synergic effect of essential oils from Vietnamese Cinnamomum cassia and Alpinia coriandriodora on Malassezia species. Int. J. Agr. Technol. 18, 1279–1292.
Trombetta, D., Saija, A., Bisignano, G., Arena, S., Caruso, S., Mazzanti, G., et al. (2002). Study on the mechanisms of the antibacterial action of some plant α,β-unsaturated aldehydes. Lett. Appl. Microbiol. 35, 285–290. doi: 10.1046/j.1472-765X.2002.01190.x
Van, H. T., Thang, T. D., Luu, T. N., Doan, V. D. (2021). An overview of the chemical composition and biological activities of essential oils from Alpinia genus (Zingiberaceae). Rsc Adv. 11, 37767. doi: 10.1039/D1RA07370B
Vessal, M., Hemmati, M., Vasei, M. (2003). Antidiabetic effects of quercetin in streptozocin-induced diabetic rats. Comp. Biochem. Physiol. Toxicol. Pharmacol. 135, 357–364. doi: 10.1016/S1532-0456(03)00140-6
Victório, C. P. (2011). Therapeutic value of the genus Alpinia, Zingiberaceae. Rev. Bras. Farmacogn. 21, 194–201. doi: 10.1590/S0102-695X2011005000025
Wang, Y., Liu, L., Ge, M., Cui, J., Dong, X., Shao, Y. (2023). Acacetin attenuates the pancreatic and hepatorenal dysfunction in type 2 diabetic rats induced by high-fat diet combined with streptozotocin. J. Nat. Med. 77, 446–454. doi: 10.1007/s11418-022-01675-6
WFO. (2023). Alpinia Roxb. Available at: http://www.worldfloraonline.org/taxon/wfo-4000001368 (Accessed 13 Jul 2023).
Wu, T. L., Larsen, K. (2000). “Zingiberaceae,” in Flora of China Vol. 24. Eds. Wu, Z. Y., Raven, P. H. (Beijing: Science Press & St. Louis: Missouri Botanical Garden Press), 322–377.
Wu, D. L., Liu, N., Ye, Y. S. (2016). The Zingiberaceous Resources in China (Wuhan: Huazhong University of Science and Technology Press), 14.
Yadav, V., Gupta, J., Mandhan, R., Chhillar, A. K., Dabur, R., Singh, D. D., et al. (2005). Investigations on anti-Aspergillus properties of bacterial products. Lett. Appl. Microbiol. 41, 309–314. doi: 10.1111/j.1472-765X.2005.01772.x
Yadav, A., Kujur, A., Kumar, A., Singh, P. P., Prakash, B., Dubey, N. K. (2019). Assessing the preservative efficacy of nanoencapsulated mace essential oil against food borne molds, aflatoxin B1 contamination, and free radical generation. LWT Food Sci. Technol. 108, 429–436. doi: 10.1016/j.lwt.2019.03.075
Yang, X., Eilerman, R. G. (1999). Pungent principal of Alpinia galangal (L.) Swartz and its applications. J. Agric. Food Chem. 47, 1657–1662. doi: 10.1021/jf9808224
Zhang, H., Gao, Y., Lai, P. (2017). Chemical composition, antioxidant, antimicrobial and cytotoxic activities of essential oil from Premna microphylla Turczaninow. Molecules 22, 381. doi: 10.3390/molecules22030381
Zhang, J. Q., Li, Y. B. (2015). Chinese Medicinal Zingiberaceae Plants (Beijing: China Medical Science Press), 145–147.
Keywords: Alpinia coriandriodora, phytochemical analysis, antioxidant, antimicrobial, enzyme inhibition
Citation: Wu X, Wei F, Ding F, Yang N, Niu J, Ran Y and Tian M (2023) Phytochemical analysis, antioxidant, antimicrobial, and anti-enzymatic properties of Alpinia coriandriodora (sweet ginger) rhizome. Front. Plant Sci. 14:1284931. doi: 10.3389/fpls.2023.1284931
Received: 29 August 2023; Accepted: 09 October 2023;
Published: 23 October 2023.
Edited by:
Dunja Šamec, University North, CroatiaReviewed by:
Aditi Kundu, Indian Council of Agricultural Research, IndiaMihasan Marius, Alexandru Ioan Cuza University, Romania
Copyright © 2023 Wu, Wei, Ding, Yang, Niu, Ran and Tian. This is an open-access article distributed under the terms of the Creative Commons Attribution License (CC BY). The use, distribution or reproduction in other forums is permitted, provided the original author(s) and the copyright owner(s) are credited and that the original publication in this journal is cited, in accordance with accepted academic practice. No use, distribution or reproduction is permitted which does not comply with these terms.
*Correspondence: Minyi Tian, mytian@gzu.edu.cn